Abstract
Background and objective
Endothelial dysfunction has been widely recognized in chronic airway diseases, including chronic obstructive pulmonary disease (COPD) and asthma; however, it remains unclear in asthma-COPD overlap (ACO). Neopterin (NP), a metabolite of guanosine triphosphate, is a novel biomarker for identifying the increased risk of adverse cardiovascular events. This study aims to investigate the association of NP with endothelial dysfunction and impaired lung function in COPD, asthma, and ACO patients.
Methods
A total of 77 subjects were prospectively recruited. All the participants underwent lung function test, endothelial function evaluation, including pulse wave velocity (PWV) and flow-mediated dilation (FMD), and blood sample detection. Moreover, the effect of NP on endothelial cells (ECs) in anoxic environments was assessed in vitro.
Results
Endothelial function was significantly decreased in the COPD and ACO patients compared with that in the healthy controls (P < 0.05). Forced expiratory volume in 1 s (FEV1) was negatively correlated with PWV and positively correlated with FMD (P < 0.05). NP was significantly increased in patients with chronic respiratory diseases compared with that in the control group, with COPD being the highest, followed by asthma, and ACO as the last (P < 0.05). The plasma level of NP exhibited negative correlations with FEV1 and positive correlations with PWV (P < 0.05). In vitro, a high level of NP increased the reactive oxygen species (ROS) and decreased the mitochondrial membrane potential (ΔΨm) of ECs dose-dependently in a hypoxic environment (P < 0.05).
Conclusion
NP was related to disease severity of chronic airway diseases and involved in the pathogenesis of endothelial dysfunction. A high NP level may contribute to endothelial dysfunction by increasing the oxidative stress of ECs dose-dependently in a hypoxic environment. Our findings may provide a novel evaluation and therapeutic target for endothelial dysfunction related to chronic airway diseases.
Similar content being viewed by others
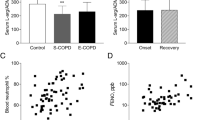
Introduction
Asthma and chronic obstructive pulmonary disease (COPD) are two of the most prevalent chronic airway diseases that pose a major public health problem [1, 2]. Patients with features of asthma and COPD, defined as asthma-COPD overlap (ACO), have been reported to have more rapid disease progression and higher mortality [3]. Accumulating evidence indicates that asthma, COPD, and ACO are correlated with higher risk of cardiovascular events [4,5,6]. The formation of local and systemic chronic inflammation [7], activation of cytokines and phlogogenic mediators, and involvement of oxidative stress [8] may lead to impairment in endothelial function, which is the link between chronic airway diseases and cardiovascular risk.
Given the inflammatory response to vascular injury, endothelial dysfunction has been widely recognized in COPD [4] and asthma [9] patients with higher cardiovascular risk and associated with pulmonary disease severity. Endothelial inflammation stimulates the production of proinflammatory cytokines, such as interleukin (IL)-6, IL-17, and IL-10 in endothelial cells (ECs) [10,11,12]. Despite the available data on the changes of cytokine levels and the bioassay of endothelial function, including pulse wave velocity (PWV) and flow-mediated dilation (FMD), in patients with COPD and asthma [9, 13], evidence for their participation in the development of systemic inflammation and endothelial function in ACO patients remains minimal.
Neopterin (NP), a metabolite of guanosine triphosphate, is produced by activated macrophages after their stimulation by γ-interferon [14]. NP has been extensively used as a novel biomarker of immune activation during inflammation and stress. Previous studies have reported that patients with coronary artery disease or hypertension have elevated plasma concentration of NP, which is associated with endothelial dysfunction [15]. However, the effect of NP on endothelial function and its association with lung function in COPD, asthma, and ACO patients have not yet been validated.
Accordingly, in the current study, we investigated the relationships among inflammatory cytokines, biomarker NP level, endothelial function (PWV and FMD), and lung function in COPD, asthma, and ACO patients, and explored the underlying mechanisms by detecting endothelial reactive oxygen species (ROS) and mitochondrial membrane potential (ΔΨm) after stimulating ECs with different concentrations of NP. This study may provide valuable knowledge for future clinical studies that focus on a useful biomarker to detect endothelial dysfunction and impaired lung function in patients with chronic airway diseases.
Methods
Subjects
This study was approved by the Human Experimentation and Ethics Committees of Sun Yat-sen University (No. [2021]072), and all the subjects signed a written informed consent. A total of 77 subjects were prospectively included from the division of pulmonary and critical care medicine of the First Affiliated Hospital of Sun Yat-sen University. The diagnosis of asthma and COPD was based on the Global Initiative for Asthma and the Global Initiative for Chronic Obstructive Lung Disease guidelines [1, 16]. The diagnosis of ACO was based on the following conditions: (1) asthma patients aged 40 years or above with persistent airflow restriction [post-bronchodilator forced expiratory volume in 1 s (FEV1) and forced vital capacity (FVC) ratio < 0.7] and had smoke or significant biomass exposure (≥ 10 pack-years for smoke and ≥ 100 h/year for wood or coal), and (2) COPD patients with a history of asthma before the age of 40 years or present a positive bronchodilator response test. A total of 17 control subjects with normal lung function (FEV1 ≥ 80% predicted and FEV1/FVC ≥ 0.7) were recruited from healthy nonsmokers. Subject exclusion criteria include the following: (1) patients with other coexisting pulmonary disease, such as interstitial lung disease, pulmonary embolism, bronchiectasis, or lung cancer; (2) patients with acute respiratory tract infection, surgery, or acute exacerbation within 1 month; and (3) patients with chronic cardiovascular diseases (CVDs), metabolic disorders, and renal or liver dysfunctions.
Lung function test
All the subjects underwent lung function test following the recommendations of the American Thoracic Society and the European Respiratory Society. All the subjects underwent lung function test in a reproducible manner, and the best values were selected. The parameters, which included FVC, FEV1, percent predicted values of these parameters (%FVC and %FEV1), FEV1/FVC ratio, and maximum voluntary ventilation (MVV), were collected. Bronchodilator response test was performed 15–20 min after the inhalation of 400 µg of salbutamol. A positive response was defined as a ≥ 12% improvement in %FEV1 and an absolute increase of ≥ 200 mL.
Endothelial function evaluation
FMD was assessed to evaluate endothelial function by using high-resolution ultrasonography (UNEX EF 38G, OMRON, Japan) in accordance with the current guidelines [17]. In summary, the resting diameter and flow data of the right brachial artery were measured. Then, supra-systolic forearm occlusion (50 mmHg above systolic blood pressure) was performed for 5 min, the cuff was subsequently deflated, and vascular dilation response was measured. FMD was calculated as the maximum percentage of change from the resting diameter to the peak diameter of the brachial artery after cuff deflation. A decrease in FMD indicates an increase in cardiovascular risk.
Arterial stiffness
Arterial stiffness was assessed via brachial-ankle PWV with applanation tonometry (BP-203RPE III, OMRON, Japan) by following the current guidelines [18]. A plethysmographic sensor was attached to the cuff wrapped around the brachial arm and ankles and used to record pulse pressure waveforms simultaneously. The surface distance between the two recording sites (Δd) and transit time (Δt) were recorded. PWV was calculated as PWV = Δd/ΔT (cm/s). The mean value of the left and right PWV was used for data analysis. An increase in PWV indicates greater arterial stiffness and cardiovascular risk.
Blood sample collection and measurement of inflammatory markers
Venous blood was collected from all the subjects after fasting overnight for at least 8 h. Inflammatory markers, i.e., serum IL-10, IL-17, IL-6, and NP, were measured using commercial enzyme-linked immunosorbent assay (MEIMIAN, Wuhan, China).
Cell cultures
Human aortic ECs (HAECs) were cultured in endothelial growth medium (EGM)-2 (Lonza, Verviers, Belgium) and exposed to hypoxia (1% oxygen) as optimized and characterized in previous studies.
ROS assay
Intracellular ROS levels were measured using the oxidant-sensing probe dichlorodihydrofluorescein diacetate (DCFH-DA). HAECs grown in six-well culture plates were exposed to hypoxia with or without NP treatment. After 24 h, the cells were incubated with 10 µM of DCFH-DA diluted in EGM-2 (serum-free) at 37 ℃ in the dark. After 20 min of incubation, the cells were washed three times with phosphate-buffered saline (PBS) and determined under flow cytometry (BD Biosciences, CA, USA).
ΔΨm measurement
ΔΨm was measured using JC-1 dye (Beyotime) in accordance with the manufacturer’s instructions. HAECs were cultured in six-well culture plates and exposed to hypoxia with or without NP treatment for 24 h. Thereafter, the cells were dyed with JC-1 staining solution at 37 ℃ for 20 min and observed under a fluorescence microscope (Leica, Wetzlar, Germany). Red fluorescent JC-1 aggregates formed in the hyperpolarized membranes, while green fluorescent JC-1 monomers indicated membrane depolarization. The higher the ratio of red-to-green fluorescence, the more intact the mitochondrial membrane.
Statistical analysis
SPSS 25.0 (SPSS, Chicago, IL) was used for statistical analysis. Normally distributed data were expressed as mean ± SD and non-normally distributed data as median (percentile 25–75). The comparison of differences among the four groups was conducted via ANOVA with post-hoc contrast tests by using the least significant difference test. Categorical variables were compared using the chi-squared test. Potential correlations among variables were explored using Pearson’s correlation test. Statistical significance was considered when P < 0.05.
Results
Clinical characteristics
The clinical characteristics of all the subjects are provided in Table 1. Among the 77 subjects, 20 were diagnosed with asthma, 20 were ACO patients, 20 had COPD, and 17 were healthy controls. No significant difference in age, height, weight, and blood pressure was found among the four groups. Patients in the COPD group were all males with lower body mass index (BMI) compared with the subjects in the other groups. The three patient groups exhibited lower lung function than the healthy controls. In addition, the spirometry parameters, including FVC% predicted, FEV1% predicted, the FEV1/FVC ratio, and MVV% predicted, in the ACO group were between those in the asthma and COPD groups.
Endothelial function was significantly decreased in the COPD (PWV: 1830.3 ± 554.6 cm/s versus 1432.4 ± 286.4 cm/s, P < 0.05) and ACO patients (FMD: 5.36 ± 1.61% versus 7.24 ± 3.30%) as compared to health control (P < 0.05). No significant difference in the levels of endothelial function was found among the asthma, ACO, and COPD patients (Fig. 1A and B).
The inflammatory marker NP was significantly increased in patients with chronic respiratory diseases than in the control group, with COPD being the highest, asthma as the second, and ACO as the last. (NP: 9.0 ± 2.6 nmol/L in COPD, 8.1 ± 2.6 nmol/L in asthma, and 7.8 ± 2.6 nmol/L in ACO versus 5.9 ± 2.7 nmol/L in the healthy controls, P < 0.05), as shown in Fig. 1C. Other inflammatory markers, including IL-10, IL-17, and IL-6, were comparable in all four groups.
Comparison among subjects with different lung functions
In accordance with the lung function parameter FEV1, the subjects were further divided into four groups as presented in Table 2: Group I (FEV1 ≥ 80% predicted), Group II (80% > FEV1 ≥ 50% predicted), Group III (50% > FEV1 ≥ 30% predicted) and Group IV (FEV1 < 30% predicted). We then compared clinical manifestation among the four groups. No significant difference was found with regard to age, gender, height, weight, and blood pressure. The subjects with poor lung function had lower BMI, indicating poorer nutrition condition.
PWV in Group IV was significantly increased compared with those in the three other groups (PWV: 2037.7 ± 727.6 cm/s in Group IV versus 1524.5 ± 337.3 cm/s in Group I, 1664.6 ± 421.2 cm/s in Group II, and 1667.0 ± 355.7 cm/s in Group III, P<0.05). FMD was significantly decreased in Groups III and IV compared with that in Group I (FMD: 5.5 ± 2.0% in Group III and 4.7 ± 2.5% in Group IV versus 7.1 ± 3.5% in Group I, P < 0.05) (Fig. 1D and E).
With regard to the plasma level of NP, all the patients with impaired lung function exhibited a significant increased level compared with the subjects in Group I (NP: 8.5 ± 2.9 nmol/L in Group II, 8.3 ± 2.4 nmol/L in Group III, and 8.9 ± 3.5 nmol/L in Group IV versus 6.7 ± 2.6 nmol/L in Group I, P < 0.05). No significant difference in IL-10, IL-17, and IL-6 was found among the four groups (Fig. 1F).
Relationships between lung function and endothelial parameter
All the study subjects were aggregated as a group to explore the possible correlations between lung function and endothelial parameter. The lung function FEV1 was found to be negatively correlated with PWV but positively correlated with FMD (P < 0.05) (Fig. 2A and B). The plasma level of NP exhibited negative correlations with FEV1 and positive correlations with PWV (P < 0.05) (Fig. 2C and D).
Effects of NP on oxidative stress in HAECs exposed to hypoxia
The effects of NP on the hypoxia-mediated accumulation of ROS in HAECs at 24 h were investigated using DCFH-DA staining. The flow cytometry results showed that the generation of ROS triggered by hypoxia in HAECs was intensified by NP treatment dose-dependently (Fig. 3A and B). Then, we assessed the effects of NP on mitochondrial function through ΔΨm detection in HAECs exposed to hypoxia. As shown in Fig. 3 C and 3D, ΔΨm damage was measured using JC-1 dye. Reduced ΔΨm (J-aggregates/monomer ratio was decreased) was detected in a hypoxia environment and was worsen with the treatment of NP dose-dependently compared with the group without NP treatment (P < 0.0001). These data suggest that NP treatment affected oxidative stress dose-dependently in hypoxic salvage.
Effects of NP on oxidative stress in HAECs exposed to hypoxia. (A–B): ROS accumulation in HAECs with or without neopterin treatment (0-200 nmol /L) were detected using DCFH-DA staining in hypoxia environment (n = 7). (C–D): Mitochondrial function in HAECs with or without NP treatment (0–200 nmol /L) was detected through ΔΨm (J-aggregates/monomer ratio) in a hypoxic environment (n = 3). (*P < 0.05, ****P < 0.0001)
Discussion
The findings of the present study revealed a significant correlation between lung function measured by FEV1 and vascular endothelial dysfunction by using PWV and FMD in patients with COPD, asthma, and ACO. Moreover, reduced FEV1, increased PWV, and decreased FMD were significantly associated with elevated NP level in patients with varying degrees of disease severity, providing a useful biomarker for the early detection of endothelial dysfunction. In addition, a high NP level increased the oxidative stress of ECs dose-dependently in a hypoxic environment, and this phenomenon can be correlated with inflammatory vascular injury. This study demonstrates the association among NP, lung function, and endothelial dysfunction in patients with chronic airway diseases for the first time, suggesting that serum NP may be attributed to cardiovascular risk as a biomarker of systemic inflammation and a participant of endothelial dysfunction in obstructive airway diseases.
Chronic airway diseases, including asthma, COPD, and ACO, have been reported to be associated with the risk of CVD [4]. The early identification of changes in the cardiovascular system before the onset of CVD is of considerable significance for preventing such diseases. Endothelial dysfunction occurs prior to the formation of atherosclerotic plaque and interferes with the normal homeostasis of vascular integrity, which can be detected early with the noninvasive measurement of FMD and PWV [19]. Chronic airway diseases have been reported to present with endothelial dysfunction by several studies [9, 13] and our previous studies [20, 21]. Individuals with COPD [13] and asthma [9] have exhibited vascular impairments, resulting in increased vascular stiffness (PWV) and worse endothelial function (FMD) in systemic arteries. In the current study, we extended these observations in asthma and COPD and found that the endothelial dysfunction of ACO was an intermediate condition between those of asthma and COPD. Moreover, a positive correlation existed between lung function assessed via FEV1 and endothelial function, suggesting that lung function is more important than disease type in predicting endothelial dysfunction. These findings underscore the importance of the early detection of vascular dysfunction in patients with chronic airway diseases accompanied by decreased lung function.
Inflammation plays an important role in the occurrence and progression of atherosclerosis and cardiovascular events. NP is considered a biomarker of inflammation and immune system activation. Elevated NP level has been reported to identify subjects at higher risk for major adverse cardiovascular events [22]. Previous investigations have also shown a close relationship between NP level and arterial stiffness and endothelial function in patients with hypertension [15]. In line with the preceding evidence, we first reported that NP level was higher in asthma, COPD, and ACO patients than in the healthy controls and significantly associated with PWV and FMD. In addition, we found that NP level was directly related to lung function, implying that NP may be a key factor that links impaired lung function and endothelial dysfunction. Therefore, patients with chronic airway diseases with high NP level may require aggressive CVD risk factor assessment and more intensive therapy. Moreover, NP level may serve as a therapeutic target, because a previous study in hypertension suggested that NP level can be reduced by antihypertensive treatment and the decrease in NP is linearly correlated with the improvement in vascular endothelial function [15]. Thus, more intervention studies that target NP are warranted in patients with obstructive airway diseases to attenuate CVD risk.
Serum NP has been associated with chronic airway obstruction and endothelial dysfunction; however, the mechanism remains unclear and probably multifactorial, involving hypoxia, inflammation, and increased ROS. Our previous studies have demonstrated that ROS and mitochondrial function are involved in the modulation of the endothelial function under various physiologic and pathologic conditions [23, 24]. In addition, the amount of NP has been reported to be related to its ability to release ROS and the toxic effects caused by enhancing ROS [25]. Therefore, NP can be regarded as an indirect estimate of oxidative stress that emerges during cell-mediated immune response. Here, we detected the ROS and mitochondrial function of ECs in vitro to determine whether NP was related to cell function and found that a high NP level could increase ROS and reduce ΔΨm in an anoxic environment dose-dependently. This finding is consistent with the effect of other inflammatory cytokines on oxidative stress [26]. Thus, the increased level of ROS and decreased ΔΨm induced by elevated NP concentrations may contribute to endothelial dysfunction in chronic airway diseases. The data reported here for the first time proved the association between NP and the development of endothelial dysfunction through the regulation of mitochondrial dysfunction in anoxic environments. Therefore, our current study confirms the importance of NP and oxidative stress in the vascular health of patients with chronic airway diseases, providing a new target for the treatment of airway disease-related cardiovascular comorbidity.
Our study has several limitations. First, the cross-sectional design and the lack of follow-up data limit the analysis of the causal relationship among chronic airway diseases, endothelial dysfunction, serum NP, and future cardiovascular events, warranting further investigation. Second, the sample size of the study is relatively small, which may lead to the trend of some data changes, but without statistical difference. Therefore, studies with a larger sample size are necessary to confirm our conclusions. Third, patients with COPD and poor lung function were all males and presented with significantly decreased BMI, which may underestimate the difference in endothelial function compared with those in the other groups. Finally, although our data suggest that NP may be involved in vascular injury by increasing endothelial cell ROS and decreasing ΔΨm in a hypoxic environment, the specific regulatory mechanisms remain to be explored further.
Conclusion
In summary, patients with chronic airway diseases, including asthma, COPD, and ACO, with impaired lung function suffer from endothelial dysfunction assessed via PWV and FMD, which are early warnings of cardiovascular risk. As a biomarker of inflammation, NP is related to disease severity and involved in the pathogenesis of endothelial dysfunction. NP may be involved by increasing the oxidative stress of ECs in a hypoxic environment, providing a novel biomarker and therapeutic target for the treatment of chronic airway diseases related to endothelial dysfunction.
Data availability
The datasets used during the current study are available from the corresponding author on reasonable request.
Abbreviations
- COPD:
-
chronic obstructive pulmonary disease
- ACO:
-
asthma-COPD overlap
- NP:
-
neopterin
- baPWV:
-
brankial-ankle pulse wave velocity
- FMD:
-
flow-mediated dilation
- ECs:
-
endothelial cells
- IL:
-
interleukin
- FVC:
-
forced vital capacity
- FEV1:
-
forced expiratory volume in one second
- MVV:
-
maximal voluntary ventilation
- BMI:
-
body mass index
- SBP:
-
systolic blood pressure
- DBP:
-
diastole blood pressure
- ROS:
-
reactive oxygen species
- CVD:
-
cardiovascular disease
References
Halpin D, Criner GJ, Papi A, Singh D, Anzueto A, Martinez FJ, et al. Global Initiative for the diagnosis, management, and Prevention of Chronic Obstructive Lung Disease. The 2020 GOLD Science Committee Report on COVID-19 and Chronic Obstructive Pulmonary Disease. Am J Respir Crit Care Med. 2021;203:24–36. https://doi.org/10.1164/rccm.202009-3533SO.
Reddel HK, Bacharier LB, Bateman ED, Brightling CE, Brusselle GG, Buhl R, et al. Global Initiative for Asthma Strategy 2021: executive summary and rationale for key changes. Eur Respir J. 2022;59. https://doi.org/10.1183/13993003.02730-2021.
Kendzerska T, Sadatsafavi M, Aaron SD, To TM, Lougheed MD, FitzGerald JM, et al. Concurrent physician-diagnosed asthma and chronic obstructive pulmonary disease: a population study of prevalence, incidence and mortality. PLoS ONE. 2017;12:e0173830. https://doi.org/10.1371/journal.pone.0173830.
Yeh JJ, Wei YF, Lin CL, Hsu WH. Association of asthma-chronic obstructive pulmonary disease overlap syndrome with coronary artery disease, cardiac dysrhythmia and heart failure: a population-based retrospective cohort study. BMJ Open. 2017;7:e017657. https://doi.org/10.1136/bmjopen-2017-017657.
Chen W, Thomas J, Sadatsafavi M, FitzGerald JM. Risk of cardiovascular comorbidity in patients with chronic obstructive pulmonary disease: a systematic review and meta-analysis. Lancet Respir Med. 2015;3:631–9. https://doi.org/10.1016/S2213-2600(15)00241-6.
Xu M, Xu J, Yang X. Asthma and risk of cardiovascular disease or all-cause mortality: a meta-analysis. Ann Saudi Med. 2017;37:99–105. https://doi.org/10.5144/0256-4947.2017.99.
Wang L, Cheng CK, Yi M, Lui KO, Huang Y. Targeting endothelial dysfunction and inflammation. J Mol Cell Cardiol. 2022;168:58–67. https://doi.org/10.1016/j.yjmcc.2022.04.011.
Batty M, Bennett MR, Yu E. The role of oxidative stress in atherosclerosis. Cells. 2022;11. https://doi.org/10.3390/cells11233843.
Henry SL, Moore LE, Brotto AR, Rowland S, Fuhr D, Stickland MK. Systemic vascular health is compromised in both confirmed and unconfirmed asthma. Respir Med. 2022;200:106932. https://doi.org/10.1016/j.rmed.2022.106932.
Yue Y, Wang C, Benedict C, Huang G, Truongcao M, Roy R, et al. Interleukin-10 Deficiency alters endothelial progenitor cell-derived exosome reparative effect on myocardial repair via integrin-linked kinase Enrichment. Circ Res. 2020;126:315–29. https://doi.org/10.1161/CIRCRESAHA.119.315829.
Ohta M, Kihara T, Toriuchi K, Aoki H, Iwaki S, Kakita H, et al. IL-6 promotes cell adhesion in human endothelial cells via microRNA-126-3p suppression. Exp Cell Res. 2020;393:112094. https://doi.org/10.1016/j.yexcr.2020.112094.
Zhang L, Liu M, Liu W, Hu C, Li H, Deng J, et al. Th17/IL-17 induces endothelial cell senescence via activation of NF-κB/p53/Rb signaling pathway. Lab Invest. 2021;101:1418–26. https://doi.org/10.1038/s41374-021-00629-y.
Piccari L, Del Pozo R, Blanco I, García-Lucio J, Torralba Y, Tura-Ceide O, et al. Association between systemic and pulmonary vascular dysfunction in COPD. Int J Chron Obstruct Pulmon Dis. 2020;15:2037–47. https://doi.org/10.2147/COPD.S257679.
Gieseg SP, Crone EM, Flavall EA, Amit Z. Potential to inhibit growth of atherosclerotic plaque development through modulation of macrophage neopterin/7,8-dihydroneopterin synthesis. Br J Pharmacol. 2008;153:627–35. https://doi.org/10.1038/sj.bjp.0707408.
Zhang YY, Tong XZ, Xia WH, Xie WL, Yu BB, Zhang B, et al. Increased plasma neopterin levels are associated with reduced endothelial function and arterial elasticity in hypertension. J Hum Hypertens. 2016;30:436–41. https://doi.org/10.1038/jhh.2015.72.
Bateman ED, Hurd SS, Barnes PJ, Bousquet J, Drazen JM, FitzGerald JM, et al. Global strategy for asthma management and prevention: GINA executive summary. Eur Respir J. 2008;31:143–78. https://doi.org/10.1183/09031936.00138707.
Thijssen DH, Black MA, Pyke KE, Padilla J, Atkinson G, Harris RA, et al. Assessment of flow-mediated dilation in humans: a methodological and physiological guideline. Am J Physiol Heart Circ Physiol. 2011;300:H2–12. https://doi.org/10.1152/ajpheart.00471.2010.
Laurent S, Cockcroft J, Van Bortel L, Boutouyrie P, Giannattasio C, Hayoz D, et al. Expert consensus document on arterial stiffness: methodological issues and clinical applications. Eur Heart J. 2006;27:2588–605. https://doi.org/10.1093/eurheartj/ehl254.
Vlachopoulos C, Aznaouridis K, Stefanadis C. Prediction of cardiovascular events and all-cause mortality with arterial stiffness: a systematic review and meta-analysis. J Am Coll Cardiol. 2010;55:1318–27. https://doi.org/10.1016/j.jacc.2009.10.061.
Liu Y, Liu X, Lin G, Sun L, Li H, Xie C. Decreased CD34 + cell number is correlated with cardiac dysfunction in patients with acute exacerbation of COPD. Heart Lung Circ. 2014;23:875–82. https://doi.org/10.1016/j.hlc.2014.03.008.
Liu Y, Huang X, Chen D, Chen F, Mo C, Guo Y, et al. The detrimental qualitative and quantitative alterations of circulating endothelial progenitor cells in patients with bronchiectasis. Respir Med. 2021;176:106270. https://doi.org/10.1016/j.rmed.2020.106270.
Ray KK, Morrow DA, Sabatine MS, Shui A, Rifai N, Cannon CP, et al. Long-term prognostic value of neopterin: a novel marker of monocyte activation in patients with acute coronary syndrome. Circulation. 2007;115:3071–8. https://doi.org/10.1161/CIRCULATIONAHA.106.666511.
He J, Liu X, Su C, Wu F, Sun J, Zhang J, et al. Inhibition of mitochondrial oxidative damage improves reendothelialization capacity of endothelial progenitor cells via SIRT3 (Sirtuin 3)-Enhanced SOD2 (Superoxide dismutase 2) Deacetylation in Hypertension. Arterioscler Thromb Vasc Biol. 2019;39:1682–98. https://doi.org/10.1161/ATVBAHA.119.312613.
Yu BB, Zhi H, Zhang XY, Liang JW, He J, Su C, et al. Mitochondrial dysfunction-mediated decline in angiogenic capacity of endothelial progenitor cells is associated with capillary rarefaction in patients with hypertension via downregulation of CXCR4/JAK2/SIRT5 signaling. EBioMedicine. 2019;42:64–75. https://doi.org/10.1016/j.ebiom.2019.03.031.
Murr C, Fuith LC, Widner B, Wirleitner B, Baier-Bitterlich G, Fuchs D. Increased neopterin concentrations in patients with cancer: indicator of oxidative stress. Anticancer Res. 1999;19:1721–8.
Wu B, You S, Qian H, Wu S, Lu S, Zhang Y, et al. The role of SIRT2 in vascular-related and heart-related diseases: a review. J Cell Mol Med. 2021;25:6470–8. https://doi.org/10.1111/jcmm.16618.
Acknowledgements
Not Applicable.
Funding
This work was supported by the National Natural Science Foundation of China (82100054, 82200029, 82300027, 82230125), the Basic and Applied Basic Research Foundation of Guangdong Province (2020A1515110933), the Nature Science Foundation of Guangdong (2021A1515010914) and the Science and Technology Project of Guangzhou City (2024A04J9906).
Author information
Authors and Affiliations
Contributions
Conception and design: LYL, CFJ, ZZM and ZXY; Samples collection: CFJ, ZZM and CDB; Experiment performance: CFJ, ZZM, LCC and CDB; Analysis and interpretation of data: LYL and ZXY. Drafting the article: LYL and ZXY. All authors reviewed the manuscript.
Corresponding authors
Ethics declarations
Ethics approval and consent to participate
The study protocol was approved by the Ethical Committee of Human Experimentation in the First Affiliated Hospital of Sun Yat-sen University and all participants provided written informed consent.
Consent to publish
Not applicable.
Competing interests
The authors declare no competing interests.
Additional information
Publisher’s Note
Springer Nature remains neutral with regard to jurisdictional claims in published maps and institutional affiliations.
Rights and permissions
Open Access This article is licensed under a Creative Commons Attribution 4.0 International License, which permits use, sharing, adaptation, distribution and reproduction in any medium or format, as long as you give appropriate credit to the original author(s) and the source, provide a link to the Creative Commons licence, and indicate if changes were made. The images or other third party material in this article are included in the article’s Creative Commons licence, unless indicated otherwise in a credit line to the material. If material is not included in the article’s Creative Commons licence and your intended use is not permitted by statutory regulation or exceeds the permitted use, you will need to obtain permission directly from the copyright holder. To view a copy of this licence, visit http://creativecommons.org/licenses/by/4.0/. The Creative Commons Public Domain Dedication waiver (http://creativecommons.org/publicdomain/zero/1.0/) applies to the data made available in this article, unless otherwise stated in a credit line to the data.
About this article
Cite this article
Liu, Y., Chen, F., Zeng, Z. et al. Neopterin in patients with COPD, asthma, and ACO: association with endothelial and lung functions. Respir Res 25, 171 (2024). https://doi.org/10.1186/s12931-024-02784-4
Received:
Accepted:
Published:
DOI: https://doi.org/10.1186/s12931-024-02784-4