Abstract
Background
The coronavirus disease 2019 (COVID-19) pandemic has changed respiratory infection patterns globally. However, its impact on community-acquired pneumonia (CAP) in high-risk patients with haematological malignancies (HM) is uncertain. We aimed to examine how community-acquired pneumonia aetiology in patients with haematological malignancies changed during the COVID-19 pandemic.
Methods
This was a retrospective study that included 524 patients with haematological malignancies hospitalised with community-acquired pneumonia between March 2018 and February 2022. Patients who underwent bronchoscopy within 24 h of admission to identify community-acquired pneumonia aetiology were included. Data on patient characteristics, laboratory findings, and results of bronchioalveolar lavage fluid cultures and polymerase chain reaction tests were analysed and compared to identify changes and in-hospital mortality risk factors.
Results
Patients were divided into the ‘pre-COVID-19 era’ (44.5%) and ‘COVID-19 era’ (55.5%) groups. The incidence of viral community-acquired pneumonia significantly decreased in the COVID-19 era, particularly for influenza A, parainfluenza, adenovirus, and rhinovirus (pre-COVID-19 era vs. COVID-19 era: 3.0% vs. 0.3%, P = 0.036; 6.5% vs. 0.7%, P = 0.001; 5.6% vs. 1.4%, P = 0.015; and 9.5% vs. 1.7%, P < 0.001, respectively), whereas that of bacterial, fungal, and unknown community-acquired pneumonia aetiologies remain unchanged. Higher Sequential Organ Failure Assessment scores and lower platelet counts correlated with in-hospital mortality after adjusting for potential confounding factors.
Conclusions
In the COVID-19 era, the incidence of community-acquired pneumonia with viral aetiologies markedly decreased among patients with haematological malignancies, with no changes in the incidence of bacterial and fungal pneumonia. Further studies are required to evaluate the impact of COVID-19 on the prognosis of patients with haematological malignancies and community-acquired pneumonia.
Similar content being viewed by others
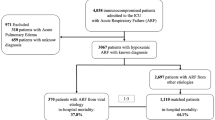
Background
The coronavirus disease 2019 (COVID-19) pandemic led to significant changes in respiratory infection patterns. After its outbreak in December 2019, in Wuhan, China, COVID-19 spread rapidly worldwide. Governments globally have extensively advocated for a range of measures to curb the COVID-19 pandemic, such as wearing masks, education on hand hygiene, social distancing, and travel restrictions. The Korean government has implemented several measures since February 2020 to prevent COVID-19 outbreaks, including active epidemiological investigations, quarantine of patients suspected of having the disease, and extensive public lockdowns [1]. The implementation of these strategies not only resulted in a decrease in the spread of COVID-19 but also substantially reduced that of other respiratory infections. Previous studies have reported a reduction in seasonal influenza activity [2,3,4], leading to a subsequent decrease in the occurrence of community-acquired pneumonia and hospital as well as intensive care unit (ICU) admissions [5].
Owing to both the characteristics of their condition and antineoplastic therapies, patients with haematological malignancies (HM) are immunocompromised and at high risk of infectious complications. They have a high incidence of community-acquired pneumonia (CAP), leading to substantial morbidity and mortality within this population [6, 7]. Early identification of CAP aetiology in patients with HM is important; however, despite the use of a comprehensive diagnostic workup, the aetiology of pneumonia is often under identified [8, 9]. Previous studies have revealed that immunocompromised patients with pneumonia of unknown aetiology have increased mortality rates [10, 11]. Furthermore, due to their immunocompromised status, the aetiology of CAP in these patients presents distinct characteristics from that in the general population. Therefore, a thorough exploration of the changing patterns in the aetiology of CAP, specifically in patients with HM, is warranted. Moreover, the impact of the COVID-19 era on the changing patterns of CAP in patients with HM remains uncertain.
Methods
Patients
This study aimed to examine the changes in CAP aetiology in patients with HM during the COVID-19 pandemic. We retrospectively studied a cohort of patients with HM who visited the emergency department or outpatient clinic of Seoul St. Mary’s Hospital (Seoul, Korea) with respiratory symptoms and required admission between March 2018 and February 2022. In this hospital, more than 500 patients undergo haematopoietic stem cell transplantations annually. Eligible patients included those who exhibited abnormal findings on chest radiographs and underwent bronchoscopy (BRS) within 24 h of admission. Patients who did not undergo BRS within 24 h of admission, those diagnosed with hospital-acquired pneumonia (HAP), those diagnosed with a non-infectious disease, and those newly diagnosed with COVID-19 were excluded (Fig. 1). All patients admitted to the hospital from January 31, 2020, when COVID-19 testing became available in South Korea, were tested for COVID-19. Patients with COVID-19 were excluded because they could not undergo invasive procedures, such as bronchoalveolar lavage (BAL), to determine the aetiology of their respiratory infection, in accordance with hospital policy. Patients with HM were defined as those who underwent concurrent evaluations and treatment without remission [12]. This study was approved by the Institutional Review Board of Seoul St. Mary’s Hospital (KC23RISI0113), and the requirement for informed consent was waived by the Institutional Review Board of Seoul St. Mary’s Hospital.
Data collection
Epidemiological and clinical data were collected from the patients’ medical charts at admission. Data included sex; age; laboratory findings, including white blood cell (WBC) count, absolute neutrophil count (ANC), absolute lymphocyte count (ALC), haemoglobin level, haematocrit level, platelet count, and C-reactive protein (CRP) level; characteristics of the HMs, including the type of malignancy, current status, and prior treatments; Sequential Organ Failure Assessment (SOFA) score at admission; body temperature at admission; and pattern of chest radiographic abnormalities. The results of BAL fluid culture, BAL galactomannan test, special staining (Gomori methenamine silver, periodic acid Schiff, or Ziehl–Neelsen) of BAL cells, atypical pneumonia serological panel (Chlamydia, Legionella, and Mycoplasma), blood culture, and serum galactomannan were also reviewed. A respiratory virus polymerase chain reaction (PCR) multiplex panel (AdvanSure RV real-time PCR Kit; LG Life Sciences, Seoul, Korea) was used to test for influenza A and B viruses, parainfluenza, respiratory syncytial virus (RSV), adenovirus, bocavirus, human metapneumovirus, coronavirus, and human rhinovirus in BAL samples. Nucleic acid extraction was performed using a QIAamp DNA Mini Kit in an automated extractor (Qiacube, Qiagen, Hilden, Germany).
Pneumonia was defined as the presence of a new infiltration on a chest radiograph at the time of the hospital visit with more than one of the following criteria: (1) new or increased cough with or without sputum production, (2) fever (temperature ≥ 38.0℃) or hypothermia (< 35.0℃), and (3) evidence of systemic inflammation (abnormal WBC count or increased CRP level) [6]. Invasive fungal diseases, including pulmonary aspergillosis, were defined based on the presence of compatible host factors, clinical features, and mycological evidence according to the European Organisation for Research and Treatment of Cancer/Mycoses Study Group criteria [13]. The diagnosis of Pneumocystis jirovecii pneumonia was based on the identification of the organism and/or positive PCR results from the BAL fluid, along with compatible clinical features and radiological findings [13]. CMV pneumonia was defined as cases that conformed to the criteria for either proven or probable CMV pneumonia and where the patient received appropriate treatment for CMV [14]. CAP cases of unknown aetiology were characterised by negative results in both BAL culture and PCR tests as well as negative serological examinations where no other identifiable cause of pulmonary infiltration could be found. Patients with HAP were defined as those admitted to the hospital for a reason other than acute respiratory infection, in whom respiratory symptoms developed ≥ 72 h after admission. Finally, we examined the clinical outcomes, including ICU admission and in-hospital mortality.
Statistical analysis
Continuous variables were reported as median (range), whereas categorical variables were described as numbers (%). Patient characteristics were compared using the chi-squared test or Fisher’s exact test, as appropriate, for categorical variables and independent sample t-tests for continuous variables. The odds ratios (OR) and their corresponding confidence intervals (CI) were computed. Goodness-of-fit was computed to assess the relevance of the logistic regression model. All tests were two-sided, and a P value < 0.05 was considered statistically significant. All statistical analyses were performed using SPSS for Windows software (ver. 20.0; IBM Corp., Armonk, NY, USA) and R (ver. 4.3.1, R Foundation, Venna, Austria).
Results
Patient characteristics
Among the 1,296 patients with HM, respiratory symptoms, and chest radiographic infiltration admitted to our hospital between March 2018 and February 2022, 524 were included in our analysis (Fig. 1). As the Korean government implemented COVID-19 containment policies in February 2020, we divided the timeline into ‘pre-COVID-19’ and ‘COVID-19’ eras, using February 2020 as the point of demarcation [1]. The ‘pre-COVID-19 era’ and ‘COVID-19 era’ groups comprised 233 (44.5%) and 291 (55.5%) patients, respectively. Aside from a higher proportion of patients with bilateral pulmonary infiltration on their chest radiographs in the pre-COVID-19 era group than in the COVID-19 era group (81.1% vs. 69.4%, respectively, P = 0.003), no significant differences were observed between the two groups in terms of patient characteristics (Table 1).
Changes in CAP aetiology
No significant differences were observed in the number of HM patients admitted or in the proportion of those admitted due to CAP when comparing the pre-COVID-19 era and COVID-19 era groups (P = 0.322) (Fig. 2). Table 2; Fig. 3 show the changes in the aetiology of CAP during the pandemic. During the pre-COVID-19 era, the proportions of aetiologies were as follows: unknown aetiology (39.5%), respiratory virus (30.7%), bacteria (23.2%), and fungus (16.3%). During the COVID-19 era, the proportion of aetiologies changed to the following: unknown aetiology (45.7%), bacteria (24.4%), fungus (18.9%), and respiratory virus (6.6%). From the pre-COVID-19 era to the COVID-19 era, the proportions of bacterial, fungal, and unknown aetiology CAP remained unchanged, whereas that of viral CAP significantly decreased. As shown in Table 2; Fig. 4, the proportion of respiratory viruses decreased during the pandemic. In particular, a significant reduction in the incidence of influenza A (pre-COVID-19 era vs. COVID-19 era: 3.0% vs. 0.3%, P = 0.036), parainfluenza (6.5% vs. 0.7%, P = 0.001), adenovirus (5.6% vs. 1.4%, P = 0.015), and rhinovirus (9.5% vs. 1.7%, P < 0.001) were observed during the pandemic. However, in the winter of 2021, an increase in the proportion of respiratory viruses was observed, which appeared to be primarily due to a rise in RSV cases (Figs. 3 and 4).
Seasonal distribution of respiratory virus infections. ADENO, adenovirus; BOCA, bocavirus; CORONA, human coronavirus; INF A, Influenza A; INF B, Influenza B; META, metapneumovirus; PARA, parainfluenza virus; RHINO, rhinovirus; RSV, respiratory syncytial virus. Spring, from March to May; Summer, from June to August; Fall, from September to November; Winter, from December to February
Immune status and aetiology of CAP
An additional Table 1 illustrates the relationship between immune status of patients and the etiology of CAP [see additional Table S1]. The data indicates no significant overall variations in CAP etiology correlated with immune status. However, the percentage of patients with acute leukemia was lower among patients with respiratory viruses than those with other aetiologies (35.6% vs. 48.8%, P = 0.029). Additionally, a higher proportion of relapsed disease was noted in patients with CMV than in those with other aetiologies (77.8% vs. 23.1%, P = 0.001).
Factors associated with increased mortality
An additional Table 1 presents comparisons of the clinical characteristics between patients experiencing in-hospital death and survivors [see additional Table S2]. Non-survivors had higher active HM rates (non-survivors vs. survivors: 83.0% vs. 71.3%, P = 0.020), CRP levels (12.2 [6.4–21.3] vs. 8.4 [3.7–17.5], P = 0.001), disease relapse rates (34.9% vs. 21.3%, P = 0.005), and SOFA scores (4.0 [2.0–5.0] vs. 3.0 [2.0–5.0], P < 0.001) and lower ANCs (1.4 [0.2–6.1] vs. 3.3 [0.6–6.4], P = 0.049), haemoglobin levels (8.6 [7.6–10,60] vs. 9.7 [8.4–11.8], P < 0.001), haematocrit levels (25.1 [22.1–31.2] vs. 29.3 [25.2–35.5], P < 0.001), and platelet counts (27.5 [14.0–73.0] vs. 98.0 [36.0–191.0], P < 0.001) than survivors.
The results of the logistic regression analysis of the clinical parameters used to evaluate the risk factors associated with in-hospital mortality are shown in Table 3. A high SOFA score and CRP level, an active disease, and a low haemoglobin level, haematocrit level, and platelet count were independent risk factors for in-hospital mortality. After adjusting for potential confounding factors, high SOFA scores and low platelet counts were independently associated with in-hospital mortality (P < 0.001 and P = 0.004, respectively).
OR: odds ratio; CI: confidence interval; SOFA: Sequential Organ Failure Assessment.
Discussion
In the present study, we compared the aetiology of CAP among patients with HM before and during the COVID-19 pandemic. During the pandemic, numerous studies have focused on the incidence and treatment of COVID-19 in immunocompromised patients; however, limited research has been conducted on aetiologies of CAP other than COVID-19, particularly on changes in them, in such patients. Nonetheless, considering the susceptibility of immunocompromised patients to various opportunistic infections, it is crucial to examine the changes in pathogens that can cause CAP in the COVID-19 era.
In contrast to the findings of previous studies that reported a decrease in the incidence of CAP in the COVID-19 era [15,16,17,18], in this study, the number of patients with HM hospitalised with CAP in the COVID-19 era did not decrease compared with that during the pre-COVID-19 era. Notably, the incidence of viral pneumonia demonstrated a significant decrease; however, the incidence of bacterial and fungal pneumonia remained unaffected, in contrast to other research findings. Although previous studies have reported a decline in the incidence of bacterial pneumonia [15], this study has not exhibited any decrease in its incidence during the COVID-19 pandemic. The unchanged incidence of bacterial pneumonia despite the rigorous infection control measures adopted during the COVID-19 pandemic indicates that the most significant risk factor for bacterial CAP in patients with HM is their immune status [19].
The incidence of pneumonia caused by respiratory viruses, including influenza A, parainfluenza, adenovirus, and rhinovirus, decreased significantly during the COVID-19 pandemic. Bilateral pulmonary infiltration on chest radiographs also showed a significant decrease during the COVID-19 pandemic, which was hypothesised to be a result of a decreased incidence of viral pneumonia [20]. Respiratory viruses usually cause mild upper respiratory tract infections; however, previous studies have reported that respiratory viruses are important pathogens causing CAP in immunocompromised patients and older adults [21, 22]. As reported in other studies, the implementation of various preventive measures following COVID-19 outbreaks is likely to not only curtail the transmission of COVID-19 but also reduce the incidence of viral pneumonia caused by other respiratory viruses [2,3,4].
In our study, we observed an increase in RSV incidence during the winter of 2021, which appears closely linked to the RSV outbreak in South Korea, likely due to the relaxation of domestic infection control measures in November 2021 [23]. This increase suggests a ‘rebound’ effect in the transmission of respiratory viruses, particularly RSV, following the easing of public health measures implemented during the COVID-19 pandemic. The phenomenon could be attributed to the combination of reduced herd immunity during periods of decreased infection rates and the concurrent relaxation of these measures. This underscores the importance of continuous surveillance of RSV and other respiratory viruses following changes in public health policies, urging preparedness in the healthcare system for similar future scenarios.
CAP of unknown aetiology is relatively common among immunocompromised patients [24, 25]. In this study, a substantial number of patients (42.9%) presented with CAP of unknown aetiology, despite our only including patients who underwent BRS to minimise the number of patients with an undetermined aetiology. The incidence of CAP of unknown aetiology did not decrease during the COVID-19 pandemic; however, this may not necessarily indicate that these cases were not caused by pathogens such as viruses or bacteria. The lack of testing for atypical infections and diagnostic limitations of laboratory techniques prevents us from drawing a definitive conclusion. It is plausible that these cases were associated with the patients’ underlying diseases or immunocompromised status, but further investigation is needed to establish a causal relationship.
We investigated the risk factors for in-hospital mortality in patients with HM who had CAP. Consistent with previous studies [26, 27], high SOFA scores and low platelet counts were found to be risk factors for in-hospital mortality in patients with HM who had CAP. In general, while the CURB-65 (confusion, uraemia, respiratory rate, blood pressure, age ≥ 65 years) criteria or pneumonia severity index (PSI) are commonly used to predict the prognosis of patients with pneumonia, previous studies have shown that they are not effective in immunocompromised patients with cancer [28]. Thus, we opted to use the SOFA score instead. Thrombocytopenia is also associated with the severity of and poor prognosis in patients with infections [29, 30]. Platelets play a role in inflammation and host defence mechanisms against microbial agents. Additionally, thrombocytopenia reflects bone marrow failure in patients with HM [31]. Therefore, thrombocytopenia could be a significant predictor of poor prognosis in patients with HM and CAP.
This study has several limitations. First, its retrospective design and single-centre implementation may introduce a selection bias, potentially affecting the significance of our findings. Nevertheless, we meticulously examined all admitted patients with CAP who underwent BRS in this single, large, 4-year cohort with consistent treatment protocols. The objective of our study was to assess the impact of the COVID-19 pandemic on the changing pattern of aetiology of CAP in patients with HM; thus, the setting of this study did not significantly deviate from that of a prospective observational study. Second, conducting a single-centre study may have had limitations in determining the overall aetiology of CAP in all patients with HM. However, this institution serves as the largest Asian centre for haematological disorders, performing over 500 bone marrow transplantations annually. Therefore, these data can be considered reasonably representative. Third, patients’ COVID-19 histories were not investigated. Previous studies reported that COVID-19 is a risk factor for bacterial or fungal co-infections and can play a significant role in patient prognosis [32, 33]. However, due to the retrospective nature of the study, it was not possible to conduct an investigation. Moreover, COVID-19 presents unique challenges, including limitations on conducting bronchoscopy and comprehensive pathogen evaluations. This has inevitably impacted our study, as excluding COVID-19 cases may have skewed the overall assessment of the disease spectrum. Recognizing that we are now in the post-pandemic era, it becomes evident that including known respiratory virus infections, such as COVID-19, would offer a more meaningful insight. This inclusion would enable an analysis of the aetiology during different stages of the COVID-19 pandemic and assess its impact on mortality rates.
Conclusions
In the context of pandemic eras such as that of COVID-19, understanding the changes in CAP aetiology among immunocompromised patients, including those with HM, is crucial. The CAP incidence in patients with HM did not decrease during the COVID-19 pandemic, unlike that in the general population. A notable shift in the aetiology of CAP emerged among patients with HM during the COVID-19 pandemic, with a significant reduction in the incidence of viral pneumonia but no change in that of bacterial and fungal pneumonia. Further research is needed to evaluate the effects of COVID-19 on the prognosis of patients with HM and CAP.
Data availability
The datasets used and/or analysed during the current study are available from the corresponding author on reasonable request.
Abbreviations
- ALC:
-
Absolute lymphocyte count
- ANC:
-
Absolute neutrophil count
- BAL:
-
Bronchoalveolar lavage
- BRS:
-
Bronchoscopy
- CAP:
-
Community-acquired pneumonia
- CI:
-
Confidence intervals
- CMV:
-
Cytomegalovirus
- COVID-19:
-
Coronavirus disease 2019
- CRP:
-
C-reactive protein
- HAP:
-
Hospital-acquired pneumonia
- HM:
-
Haematological malignancies
- ICU:
-
Intensive care unit
- OR:
-
Odds ratios
- PCR:
-
Polymerase chain reaction
- PSI:
-
Pneumonia severity index
- RSV:
-
Respiratory syncytial virus
- SOFA:
-
Sequential Organ Failure Assessment
- WBC:
-
White blood cell
References
Park IN, Yum HK. Stepwise strategy of Social Distancing in Korea. J Korean Med Sci. 2020;35(28):e264.
Lee H, Lee H, Song KH, Kim ES, Park JS, Jung J, Ahn S, Jeong EK, Park H, Kim HB. Impact of Public Health Interventions on Seasonal Influenza Activity during the COVID-19 outbreak in Korea. Clin Infect Dis. 2021;73(1):e132–40.
Sakamoto H, Ishikane M, Ueda P. Seasonal Influenza Activity during the SARS-CoV-2 outbreak in Japan. JAMA. 2020;323(19):1969–71.
Kuitunen I, Artama M, Makela L, Backman K, Heiskanen-Kosma T, Renko M. Effect of Social Distancing due to the COVID-19 pandemic on the incidence of viral respiratory tract infections in children in Finland during Early 2020. Pediatr Infect Dis J. 2020;39(12):e423–7.
Tang H-J. Changing epidemiology of respiratory tract infection during COVID-19 pandemic. Antibiotics; 2022.
Di Pasquale MF, Sotgiu G, Gramegna A, Radovanovic D, Terraneo S, Reyes LF, Rupp J, González Del Castillo J, Blasi F, Aliberti S, et al. Prevalence and etiology of community-acquired Pneumonia in Immunocompromised patients. Clin Infect Dis. 2019;68(9):1482–93.
Schmedt N, Heuer OD, Hackl D, Sato R, Theilacker C. Burden of community-acquired pneumonia, predisposing factors and health-care related costs in patients with cancer. BMC Health Serv Res. 2019;19(1):30.
Sharma S, Nadrous HF, Peters SG, Tefferi A, Litzow MR, Aubry MC, Afessa B. Pulmonary complications in adult blood and marrow transplant recipients: autopsy findings. Chest. 2005;128(3):1385–92.
Roychowdhury M, Pambuccian SE, Aslan DL, Jessurun J, Rose AG, Manivel JC, Gulbahce HE. Pulmonary complications after bone marrow transplantation: an autopsy study from a large transplantation center. Arch Pathol Lab Med. 2005;129(3):366–71.
Azoulay E, Mokart D, Rabbat A, Pene F, Kouatchet A, Bruneel F, Vincent F, Hamidfar R, Moreau D, Mohammedi I, et al. Diagnostic bronchoscopy in hematology and oncology patients with acute respiratory failure: prospective multicenter data. Crit Care Med. 2008;36(1):100–7.
Hilbert G, Gruson D, Vargas F, Valentino R, Gbikpi-Benissan G, Dupon M, Reiffers J, Cardinaud JP. Noninvasive ventilation in immunosuppressed patients with pulmonary infiltrates, fever, and acute respiratory failure. N Engl J Med. 2001;344(7):481–7.
Park JY, Guo W, Al-Hijji M, El Sabbagh A, Begna KH, Habermann TM, Witzig TE, Lewis BR, Lerman A, Herrmann J. Acute coronary syndromes in patients with active hematologic malignancies - incidence, management, and outcomes. Int J Cardiol. 2019;275:6–12.
Donnelly JP, Chen SC, Kauffman CA, Steinbach WJ, Baddley JW, Verweij PE, Clancy CJ, Wingard JR, Lockhart SR, Groll AH, et al. Revision and update of the Consensus definitions of Invasive Fungal Disease from the European Organization for Research and Treatment of Cancer and the Mycoses Study Group Education and Research Consortium. Clin Infect Dis. 2020;71(6):1367–76.
Ljungman P, Boeckh M, Hirsch HH, Josephson F, Lundgren J, Nichols G, Pikis A, Razonable RR, Miller V, Griffiths PD, et al. Definitions of cytomegalovirus infection and disease in transplant patients for use in clinical trials. Clin Infect Dis. 2017;64(1):87–91.
Huang C. The COVID-19 pandemic and the incidence of the Non-COVID-19 pneumonia in adults. Front Med (Lausanne). 2021;8:737999.
Zhang LN, Cao L, Meng LH. Pathogenic changes of community-acquired pneumonia in a children’s hospital in Beijing, China before and after COVID-19 onset: a retrospective study. World J Pediatr. 2022;18(11):746–52.
Yamamoto T, Komiya K, Fujita N, Okabe E, Hiramatsu K, Kadota JI. COVID-19 pandemic and the incidence of community-acquired pneumonia in elderly people. Respir Investig. 2020;58(6):435–6.
Metlay JP, Waterer GW. Treatment of Community-Acquired Pneumonia during the Coronavirus Disease 2019 (COVID-19) pandemic. Ann Intern Med. 2020;173(4):304–5.
Harris B, Geyer AI. Diagnostic evaluation of Pulmonary abnormalities in patients with hematologic malignancies and hematopoietic cell transplantation. Clin Chest Med. 2017;38(2):317–31.
Johnstone J, Majumdar SR, Fox JD, Marrie TJ. Viral infection in adults hospitalized with community-acquired pneumonia: prevalence, pathogens, and presentation. Chest. 2008;134(6):1141–8.
Martino R, Porras RP, Rabella N, Williams JV, Ramila E, Margall N, Labeaga R, Crowe JE Jr., Coll P, Sierra J. Prospective study of the incidence, clinical features, and outcome of symptomatic upper and lower respiratory tract infections by respiratory viruses in adult recipients of hematopoietic stem cell transplants for hematologic malignancies. Biol Blood Marrow Transpl. 2005;11(10):781–96.
Lee J, Kim SC, Rhee CK, Lee J, Lee JW, Lee DG. Prevalence and clinical course of upper airway respiratory virus infection in critically ill patients with hematologic malignancies. PLoS ONE. 2021;16(12):e0260741.
Kim JH, Kim HY, Lee M, Ahn JG, Baek JY, Kim MY, Huh K, Jung J, Kang JM. Respiratory Syncytial Virus Outbreak without Influenza in the Second Year of the Coronavirus Disease 2019 pandemic: a National Sentinel Surveillance in Korea, 2021–2022 season. J Korean Med Sci. 2022;37(34):e258.
Rano A, Agusti C, Jimenez P, Angrill J, Benito N, Danes C, Gonzalez J, Rovira M, Pumarola T, Moreno A, et al. Pulmonary infiltrates in non-HIV immunocompromised patients: a diagnostic approach using non-invasive and bronchoscopic procedures. Thorax. 2001;56(5):379–87.
Chaoui D, Legrand O, Roche N, Cornet M, Lefebvre A, Peffault de Latour R, Sanhes L, Huchon G, Marie JP, Rabbat A. Incidence and prognostic value of respiratory events in acute leukemia. Leukemia. 2004;18(4):670–5.
Al-Dorzi HM, Al Orainni H, Al Eid F, Tlayjeh H, Itani A, Al Hejazi A, Arabi YM. Characteristics and predictors of mortality of patients with hematologic malignancies requiring invasive mechanical ventilation. Ann Thorac Med. 2017;12(4):259–65.
Mirsaeidi M, Peyrani P, Aliberti S, Filardo G, Bordon J, Blasi F, Ramirez JA. Thrombocytopenia and thrombocytosis at time of hospitalization predict mortality in patients with community-acquired pneumonia. Chest. 2010;137(2):416–20.
Gonzalez C, Johnson T, Rolston K, Merriman K, Warneke C, Evans S. Predicting pneumonia mortality using CURB-65, PSI, and patient characteristics in patients presenting to the emergency department of a comprehensive cancer center. Cancer Med. 2014;3(4):962–70.
Maquet J, Lafaurie M, Sommet A, Moulis G. Thrombocytopenia is independently associated with poor outcome in patients hospitalized for COVID-19. Br J Haematol. 2020;190(5):e276–9.
Hui P, Cook DJ, Lim W, Fraser GA, Arnold DM. The frequency and clinical significance of Thrombocytopenia complicating critical illness: a systematic review. Chest. 2011;139(2):271–8.
Salacz ME, Lankiewicz MW, Weissman DE. Management of thrombocytopenia in bone marrow failure: a review. J Palliat Med. 2007;10(1):236–44.
Hoenigl M, Seidel D, Sprute R, Cunha C, Oliverio M, Goldman GH, Ibrahim AS, Carvalho A. COVID-19-associated fungal infections. Nat Microbiol. 2022;7(8):1127–40.
Shafran N, Shafran I, Ben-Zvi H, Sofer S, Sheena L, Krause I, Shlomai A, Goldberg E, Sklan EH. Secondary bacterial infection in COVID-19 patients is a stronger predictor for death compared to influenza patients. Sci Rep. 2021;11(1):12703.
Acknowledgements
Not applicable.
Funding
This research received no specific grant from any funding agency in the public, commercial, or not-for-profit sectors.
Author information
Authors and Affiliations
Contributions
JW analysed and interpreted the patient data and drafted the work. SC made substantial contributions to the conception. JM made design of the work and interpreted the data and substantively revised the manuscript. All authors read and approved the final manuscript.
Corresponding author
Ethics declarations
Ethics approval and consent to participate
This study was approved by the Institutional Review Board of Seoul St. Mary’s Hospital (KC23RISI0113), and the requirement for informed consent was waived by the Institutional Review Board of Seoul St. Mary’s Hospital.
Consent for publication
Not applicable.
Competing interests
The authors declare no competing interests.
Additional information
Publisher’s Note
Springer Nature remains neutral with regard to jurisdictional claims in published maps and institutional affiliations.
Electronic supplementary material
Below is the link to the electronic supplementary material.
Rights and permissions
Open Access This article is licensed under a Creative Commons Attribution 4.0 International License, which permits use, sharing, adaptation, distribution and reproduction in any medium or format, as long as you give appropriate credit to the original author(s) and the source, provide a link to the Creative Commons licence, and indicate if changes were made. The images or other third party material in this article are included in the article’s Creative Commons licence, unless indicated otherwise in a credit line to the material. If material is not included in the article’s Creative Commons licence and your intended use is not permitted by statutory regulation or exceeds the permitted use, you will need to obtain permission directly from the copyright holder. To view a copy of this licence, visit http://creativecommons.org/licenses/by/4.0/. The Creative Commons Public Domain Dedication waiver (http://creativecommons.org/publicdomain/zero/1.0/) applies to the data made available in this article, unless otherwise stated in a credit line to the data.
About this article
Cite this article
Ryoo, J., Kim, S.C. & Lee, J. Changes in respiratory infection trends during the COVID-19 pandemic in patients with haematologic malignancy. BMC Pulm Med 24, 259 (2024). https://doi.org/10.1186/s12890-024-03071-0
Received:
Accepted:
Published:
DOI: https://doi.org/10.1186/s12890-024-03071-0