Abstract
In this study, the active vibration control system design of a composite beam with three different lamination angles under forced vibration was investigated. The produced composite beam lamination angles have been selected as {0°, 90°, 0°, 90°}s, {− 30°, 60°, − 30°, 60°}s and {− 45°, 45°, − 45°, 45°}s for investigating the vibration characteristic. Different types of bluff-body geometries were attached to the free end of the cantilever composite beams. In this way, the composite beam's vibration amplitudes have more fluctuated with the help of bluff body geometries. Bluff body structures are generally preferred in energy harvesting applications by increasing the vibration in beams. The fact that this structure, which increases the vibration amplitude, is handled in an active vibration control mechanism adds a different novelty to the subject. Flow-induced vibrations were obtained for a particular period by applying air load on it. Two different geometries of bluff bodies were placed in a freestream airflow at a constant speed to trigger and enhance the vibration of the composite beam. The front surface areas of two different bluff bodies exposed to air load are identical. Therefore, the difference in the vibrations characteristics was only affected by the geometrical differences in the lateral areas of the bluff bodies. To demonstrate this situation, the airfoil efficiency was investigated for the bluff body geometries. A piezoelectric patch is attached to the surface of the composite beam, and the vibration control is acquired utilizing the PID control design. As a result of experimental studies, it has been shown that the forced vibrations on the composite structure can be suppressed successfully with the application of the PID control design.
Graphical abstract














Similar content being viewed by others
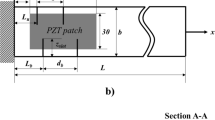
Data Availability Statement
This manuscript has associated data in a data repository. [Authors’ comment: The data that support the findings of this study are available from the corresponding author upon reasonable request.]
References
Y. Pu, H. Zhou, Z. Meng, Multi-channel adaptive active vibration control of piezoelectric smart plate with online secondary path modelling using PZT patches. Mech. Syst. Signal Process. 120, 166–179 (2019). https://doi.org/10.1016/j.ymssp.2018.10.019
S.S. Heganna, J.J. Joglekar, Active vibration control of smart structure using PZT patches. Proc. Comput. Sci. 89, 710–715 (2016). https://doi.org/10.1016/j.procs.2016.06.040
P. Shivashankar, S. Gopalakrishnan, Review on the use of piezoelectric materials for active vibration, noise, and flow control. Smart Mater. Struct. 29(5), 053001 (2020). https://doi.org/10.1088/1361-665X/ab7541
B. Tang, H. Akbari, M. Pouya, P.V. Pashaki, Application of piezoelectric patches for chatter suppression in machining processes. Measurement 138, 225–231 (2019). https://doi.org/10.1016/j.measurement.2019.02.003
Z. Arabjamaloei, M. Mofidi, M. Hosseini, R. Bahaadini, Vibration analysis of rotating composite blades with piezoelectric layers in hygrothermal environment. Eur. Phys. J. Plus 134(11), 556 (2019). https://doi.org/10.1140/epjp/i2019-12910-9
M. Li, F. Li, X. Jing, Active vibration control of composite pyramidal lattice truss core sandwich plates. J. Aerosp. Eng. 31(2), 04017097 (2018). https://doi.org/10.1061/(ASCE)AS.1943-5525.0000817
K. Gurses, B.J. Buckham, E.J. Park, Vibration control of a single-link flexible manipulator using an array of fiber optic curvature sensors and PZT actuators. Mechatronics 19(2), 167–177 (2009). https://doi.org/10.1016/j.mechatronics.2008.09.005
S.M. Kusagur, G. Arunkumar, T.C. Manjunath, Modelling of smart intelligent materials with PZT & PVDF sensors/actuators to control the active vibrations of flexible aluminum mechanical cantilever beams using proportional integral derivative (PID) techniques. Mater. Today Proc. 37, 2075–2082 (2021). https://doi.org/10.1016/j.matpr.2020.07.507
M. Azimi, E.F. Joubaneh, Dynamic modeling and vibration control of a coupled rigid-flexible high-order structural system: a comparative study. Aerosp. Sci. Technol. 102, 105875 (2020). https://doi.org/10.1016/j.ast.2020.105875
X. Yuan, X. Wang, M. Yan, F. Gao, S. Zhang, K. Zhou et al., Effects of composite layer thickness and driving conditions on the actuating performance of shear piezoelectric fiber composite. Measurement 154, 107500 (2020). https://doi.org/10.1016/j.measurement.2020.107500
G. Takács, T. Polóni, B. Rohal-Ilkiv, Adaptive model predictive vibration control of a cantilever beam with real-time parameter estimation. Shock Vib. (2014). https://doi.org/10.1155/2014/741765
A. Oveisi, M. Gudarzi, Adaptive sliding mode vibration control of a nonlinear smart beam: A comparison with self-tuning Ziegler–Nichols PID controller. J. Low Freq. Noise Vib. Active Control 32(1–2), 41–62 (2013). https://doi.org/10.1260/2F0263-0923.32.1-2.41
Q. Zhu, J.Z. Yue, W.Q. Liu, X.D. Wang, J. Chen, G.D. Hu, Active vibration control for piezoelectricity cantilever beam: an adaptive feedforward control method. Smart Mater. Struct. 26(4), 047003 (2017). https://doi.org/10.1088/1361-665X/aa64c6
S. Sivrioglu, F.C. Bolat, E. Erturk, Active vibration control of a blade element with uncertainty modeling in PZT actuator force. J. Vib. Control 25(21–22), 2721–2732 (2019). https://doi.org/10.1177/2F1077546319868883
S.H. Youn, J.H. Han, I. Lee, Neuro-adaptive vibration control of composite beams subject to sudden delamination. J. Sound Vib. 238(2), 215–231 (2000). https://doi.org/10.1006/jsvi.2000.3081
E.H. Koroishi, A.W. Faria, F.A. Lara-Molina, V. Steffen, Fuzzy modal control applied to smart composite structure. J. Phys. Conf. Ser. 628(1), 012090 (2015). https://doi.org/10.1088/1742-6596/628/1/012090
K. Ma, M.N. Ghasemi-Nejhad, Adaptive simultaneous precision positioning and vibration control of intelligent composite structures. J. Intell. Mater. Syst. Struct. 16(2), 163–174 (2005). https://doi.org/10.1177/2F1045389X05048848
E.H. Koroishi, F.A.L. Molina, A.W. Faria, V. Steffen Junior, Robust optimal control applied to a composite laminated beam. J. Aerosp. Technol. Manag. 7(1), 70–80 (2015). https://doi.org/10.5028/jatm.v7i1.389
L.H. Zheng, Y.W. Zhang, H. Ding, L.Q. Chen, Nonlinear vibration suppression of composite laminated beam embedded with NiTiNOL-steel wire ropes. Nonlinear Dyn. 103(3), 2391–2407 (2021). https://doi.org/10.1007/s11071-021-06258-w
M.M. Jovanović, A.M. Simonović, N.D. Zorić, N.S. Lukić, S.N. Stupar, S.S. Ilić, Experimental studies on active vibration control of a smart composite beam using a PID controller. Smart Mater. Struct. 22(11), 115038 (2013). https://doi.org/10.1088/0964-1726/22/11/115038
A.B. Shakir, A.M. Saber, Active vibration control analysis in smart composite structures using ANSYS. Revista Internacional de Métodos Numéricos para Cálculo y Diseño en Ingeniería (2020). https://doi.org/10.23967/j.rimni.2020.04.001
S. Varadarajan, K. Chandrashekhara, S. Agarwal, LQG/LTR-based robust control of composite beams with piezoelectric devices. J. Vib. Control 6(4), 607–630 (2000). https://doi.org/10.1177/2F107754630000600407
J. Warminski, M. Bochenski, W. Jarzyna, P. Filipek, M. Augustyniak, Active suppression of nonlinear composite beam vibrations by selected control algorithms. Commun. Nonlinear Sci. Numer. Simul. 16(5), 2237–2248 (2011). https://doi.org/10.1016/j.cnsns.2010.04.055
R. Rimašauskienė, V. Jūrėnas, M. Radzienski, M. Rimašauskas, W. Ostachowicz, Experimental analysis of active–passive vibration control on thin-walled composite beam. Compos. Struct. 223, 110975 (2019). https://doi.org/10.1016/j.compstruct.2019.110975
X.Q. Peng, K.Y. Lam, G.R. Liu, Active vibration control of composite beams with piezoelectrics: a finite element model with third order theory. J. Sound Vib. 209(4), 635–650 (1998). https://doi.org/10.1006/jsvi.1997.1249
A. Barrero-Gil, G. Alonso, A. Sanz-Andres, Energy harvesting from transverse galloping. J. Sound Vib. 329(14), 2873–2883 (2010). https://doi.org/10.1016/j.jsv.2010.01.028
Author information
Authors and Affiliations
Corresponding author
Ethics declarations
Conflict of interest
On behalf of all authors, the corresponding author states that there is no conflict of interest.
Rights and permissions
Springer Nature or its licensor holds exclusive rights to this article under a publishing agreement with the author(s) or other rightsholder(s); author self-archiving of the accepted manuscript version of this article is solely governed by the terms of such publishing agreement and applicable law.
About this article
Cite this article
Basaran, S., Bolat, F.C. Effect of lamination angle on control performance for composite beams subject to galloping-based flow-induced vibration. Eur. Phys. J. Plus 137, 911 (2022). https://doi.org/10.1140/epjp/s13360-022-03135-2
Received:
Accepted:
Published:
DOI: https://doi.org/10.1140/epjp/s13360-022-03135-2