Abstract
The dynamics of aggressive behavior of the underyearlings of the rainbow trout Oncorhynchus mykiss infected with metacercariae of the trematodes Diplostomum pseudospathaceum has been studied in an experimental heterogeneous environment for 48 days. The fish competed for the dark area of the bottom by charges, biting, chasing, and frontal and lateral displays. The sharply increased aggressiveness of fish when the parasites became infective (1.0–1.5 months after infection), i.e., capable of infecting the final host (piscivorous birds), manifested itself in an increased frequency of bites (more than 20 times compared to the control) and charges (almost three times) and was accompanied by fast movements, sharp maneuvers, and low ability to compete for shelters. The share of comparatively slow displays and chasing was much smaller. The strategy of D. pseudospathaceum manipulation of fish behavior consists of two stages: (1) protecting the host from predators; (2) increasing the vulnerability of the host. At the first stage, the general level of aggression decreases sharply; at the second, on the contrary, aggressiveness rapidly increases, but the ratio of different categories of aggression changes. The share of categories that make the host more vulnerable to predators increases. High activity and low competitiveness of infected fish contribute to their rapid extermination by predators or moving to another habitat. Such migration of fish can increase the fitness of the parasite, facilitating its dispersal in the water body.
Similar content being viewed by others
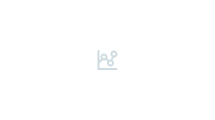
Avoid common mistakes on your manuscript.
INTRODUCTION
Competition for resources (food, shelter, sexual partner, etc.) in many animals is accompanied by agonistic behavior, including various manifestations of aggression and submission (Huntingford and Turner, 1987; Arnott and Elwood, 2009). To occupy and defend a territory, fish use a diverse arsenal of aggressive actions (Keenleyside and Yamamoto, 1962). Aggressive behavior requires a lot of time and energy (Katano and Iguchi, 1996), which can lead to growth retardation and reduced survival and reproductive success in individuals with a high level of aggression (Cutts et al., 1998; Grantner and Taborsky, 1998; Vøllestad and Quinn, 2003). Aggression is usually assessed by the total number of acts of aggression per unit of time (Kalleberg, 1958; Metcalfe, 1986), but a more detailed analysis takes into account individual categories of aggression that form the structure of the complex of such behavior: frontal and lateral displays, charges, chasing, and biting (nipping) (Keenleyside and Yamamoto, 1962; Mikheev et al., 2005).
However, researchers studying abiotic and biotic external factors that influence the aggressive behavior of fish (Grant, 1997; Mikheev, 2006; Earley and Hsu, 2013), relatively recently recognized macroparasites as a factor that modify the behavior of host fish (Mikheev et al., 2010; Slivko et al., 2021). Underyearlings of the rainbow trout Oncorhynchus mykiss infected with metacercariae of the trematode Diplostomum pseudospathaceum showed increasing aggressiveness as the parasites developed in the lenses of the fish eyes (Mikheev et al., 2010). In the work cited above, we recorded the total number of acts of aggression in fish over a standard period of time without singling out separate categories of aggressive behavior. In another study (Slivko et al., 2021), we found that juveniles of the European perch Perca fluviatilis with different infestation with plerocercoids of the cestodes Triaenophorus nodulosus showed not only different levels of aggression, but also demonstrated different types of aggressive actions. We interpreted the sharply increased aggressiveness of the rainbow trout underyearlings with infective metacercariae (Mikheev et al., 2010) as an example of host behavior manipulation (Parker et al., 2009; Poulin, 2010), which contributes to the success of parasite transmission.
We assume that the aggressiveness of infected fish is controlled both by the fish themselves and by parasites, and the goals of these two subjects are opposite. The parasite needs to get into its final host, while fish is seeking to avoid this fate. The increased aggressiveness of fish with infective trematode metacercariae may be beneficial to the parasite, making the host more visible (and vulnerable) to predators. On the other hand, if such behavior promotes the fish to acquire the shelter more successfully, it (fish) becomes less accessible to the predator, which is contrary to the parasite interests. By putting together infected and control fish in pairs, we found that, despite increased aggressiveness, infected fish almost always lost the fight for a shelter, which confirms the manipulation hypothesis (Mikheev et al., 2010).
Such an unexpected result may be associated not only with an increased total number of aggression acts in infected fish, but also with changes in the structure of aggressive behavior, i.e. the ratio of the frequency of manifestation of certain categories of aggression. Several modifications of the fish behavior caused by parasites developing in the eyes are possible: (1) the frequency of all categories of aggressive behavior increases equally; (2) new categories of aggressive behavior appear in infected fish; (3) the share of energy-consuming categories (charges and bites) accompanied by increased motor activity increases, although the deficiency of resources caused by the parasite-induced deterioration in foraging behavior (Crowden and Broom, 1980) may contradict this hypothesis; (4) the share of less costly categories (displays and chasing) increases, which leads to more economical use of resources.
The aim of this work is to study the dynamics of various categories of aggressive behavior in the rainbow trout underyearlings infected with D. pseudospathaceum. Our working hypothesis propose the following modifications of the aggressive behavior of infected fish: (1) an increased demonstration of aggression categories with a high level of activity, which can dramatically increase the visibility (vulnerability) of fish to predators; (2) on the contrary, the predominance of categories with a low level of activity that makes fish not so noticeable to predators, but can last for a long time, thus increasing the “window of vulnerability” for predators.
MATERIALS AND METHODS
The experiments were carried out at the Konnevesi Research Station of the University of Jyväskylä (Finland) in July–September 2005. Changes in the structure of aggressive behavior (the ratio of different acts of aggression) were observed in the rainbow trout underyearlings (mean total body length ± standard error (SE), 89.1 ± 1.6 mm), infected with metacercariae of D. pseudospathaceum. Fish from the same pool (obtained from a fish farm) with a low level of natural infection with D. pseudospathaceum (mean ± SE, 5.6 ± 0.6 metacercariae per fish) were used as a control. A comparison of the aggressive behavior of these fish with the behavior of underyearlings of the rainbow trout of similar size, completely free of parasites, showed that the behavior of the fish does not change at a low intensity of infection (Mikheev et al., 2010). The infection rate of the experimental group was (mean ± SE) 87.9 ± 5.8 metacercariae per fish. The method for assessing the intensity of fish infection with D. pseudospathaceum in experimental studies was described in detail earlier (Seppälä et al., 2005a; Mikheev et al., 2010).
Experiment Design
Fish (in total 280 individuals) were infected in four 150-liter aquariums. Fish were hold in these aquariums for 30 min at a concentration of cercariae in water of 200 ind./fish. After infection, the fish were kept in a 1000-liter flow tank at 15–16°C and natural photoperiod, where they were fed with food pellets of the appropriate size. The same number of control fish was kept under the same conditions. D. pseudospathaceum cercariae were obtained from eight infected pond snails Lymnaea peregra kept in a refrigerator in the dark. Four hours before fish infection, the pond snails were placed in 600 mL jars with filtered lake water, where they were kept at a temperature of 20°C and illumination of ~500 lx, which stimulated the emergence of cercariae. All cercariae were placed in one container, from which 10 samples of 1 mL were taken to assess the concentration. Observations of the behavior of experimentally infected and control fish continued for 48 days after infection. Groups of five randomly selected fish (infected or control) were released into the middle compartment of a rectangular opaque brown plastic aquarium 170 cm long and 180 liters in volume. From the middle compartment, 30 cm long, 30 cm wide, and 40 cm high, fish could move to the end compartments (70 × 30 × 40 cm) through rectangular bottom openings (5 × 3 cm) equipped with rising doors. The bottom of the middle and one of the end compartments was covered with white plastic, while the bottom of the other end compartment was dark brown. Juvenile trout prefer a dark bottom (Mikheev et al., 1996; Seppälä et al., 2005a); therefore, after a survey of the entire aquarium (takes from 0.5 to 1.0 h), the fish preferred to stay in a compartment with a dark bottom, which led to aggressive interactions.
Experiment Manipulations
At the beginning of each experiment, the fish were placed in the middle compartment with closed doors, where they got used to the environment for 15 min. The temperature was maintained within the range of 15–16°C, and the illumination was 150 lx. After acclimation, the doors were opened, and the fish were free to move around the entire aquarium for 3 h. The number of acts of aggression in a group of five fish per 30 min was counted separately by categories: charges, chasing, frontal and lateral displays, and bites (Keenleyside and Yamamoto, 1962). Two observers from behind screens with slits counted acts of aggression in the group during 15 minutes twice, first time 1.0 h after acclimation and second time 2.5 h after acclimation. For each replicate, the data from the two counts were summed. For two days, 12 observations were made on groups of infected and control fish. The behavior was observed four times: 1, 7, 30, and 48 days after infection. After each experiment, the fish were placed in separate containers and were not used in further behavioral experiments. At the end of the experimental period, the fish were weighed and their length was measured. The intensity of infection (the number of metacercariae in the fish eyes) was determined on the 7th and 48th days after infection.
Statistical Processing of the Data
The effect of infection intensity and time after infection on the frequency of aggression acts was assessed for each category of aggressive behavior using a two-way analysis of variance. To do this, the data were subjected to log(x + 1) transformation. Tukey’s test was used for pairwise post-hoc comparisons. Differences in body weight and size between control and experimental fish were assessed using the Mann–Whitney U-test.
RESULTS
The dynamics of the number of aggressive interactions (NA) in the groups of infected and control fish differed significantly during 1.5 months after infection with D. pseudospathaceum cercariae (Fig. 1). However, the nature of the differences in the first week after infection and at the end of the observation period differed significantly. Analysis of variance showed a highly significant effect of the infection factor for all categories of aggressive behavior (charges: F = 19.5, df = 1, p < 0.001; frontal and lateral displays: F = 33.28, df = 1, p < 0.001; bites: F = 124.9, df = 1, p < 0.001), except for chasing (F = 0.15, df = 1, p = 0.703). A significant effect of time after infection was noted for all categories of behavior (p < 0.001). The interaction between factors was also highly significant for all categories (p < 0.001). In control, NA changed insignificantly in all categories of aggressive behavior. In all pairwise comparisons in the control (with the only exception), NA did not differ in four time periods (Tukey’s test: p > 0.05). In infected fish, a significant decrease in aggressiveness relative to control at the beginning of the experiment (after infection) was replaced by a sharp increase in the number of charges and bites by the end of observations (Tukey’s test: p < 0.001) (Figs. 1a, 1d). The number of chasing (Fig. 1b) and displays (Fig. 1c), although increased compared to the control in the first week after infection, did not exceed the control level at the end of the observation period (Tukey’s test: p > 0.05). The average length and weight of the infected (118.7 ± 2.4 mm, 20.9 ± 1.28 g) and control (119.0 ± 1.7 mm, 21.3 ± 0.92 g) fish did not differ significantly after 48 days of the experiment (Mann–Whitney U test, p = 0.97 and 0.95, respectively).
Dynamics of the average number (12 observations) of acts of aggression per 30 min in groups of five underyearlings of Oncorhynchus mykiss during 48 days after infection of fish with Diplostomum pseudospathaceum cercariae: (a) charges; (b) chasing; (c) frontal and lateral displays; (d) bites; (◻), control, (), infected individuals, (
), standard deviation.
DISCUSSION
A detailed analysis of the dynamics (within 48 days after infection) of the aggressive behavior of the rainbow trout underyearlings infected with D. pseudospathaceum showed that the sharply increased aggressiveness of fish with infective metacercariae was associated with an increase of charges and bites, accompanied by rapid movements and abrupt maneuvers. The share of comparatively slow lateral and frontal displays and chasings was much smaller and did not differ from the control.
In the first week after infection, the aggressiveness of the fish decreased sharply compared to the control. This change in behavior is in good agreement with the hypothesis of “predation suppression”, predicted theoretically (Parker et al., 2009) and supported by empirical data (Dianne et al., 2011; Weinreich et al., 2013). Parasites that are not ready to infect the final host (fish-eating bird) are “not interested” in the early consumption of the second intermediate host (fish) by predators. Manipulation of the host behavior during this period is aimed at minimizing any activity that makes the fish visible and vulnerable to predators. D. pseudospathaceum metacercariae reach infectivity (i.e. the ability to infect the final host, piscivorous birds) in 29–47 days at a temperature of 18–22°С (Sweeting, 1974; Shigin, 1986; Voutilainen et al., 2010).
Since that time (30 days after infection), we have observed an increase in the number of acts of aggression in almost all categories of aggressive behavior. This trend was most pronounced for charges and bites, which frequency by the end of the observation period significantly exceeded the control level. On the contrary, the frequency of chasing remained at the control level, and the frequency of frontal and lateral displays was even somewhat lower. In general, increased aggressiveness of fish with infective parasites supports the hypothesis of adaptive manipulation of host behavior, i.e., parasites manipulate their intermediate hosts to enhance predation (Lafferty, 1999; Parker et al., 2009).
Furthermore, different changes in the categories of aggressive behavior with a general increase in fish aggression when parasites reach infectivity are of particular interest. In the control, throughout the entire observation period (about 1.5 months), the ratio of the number of charges, chasing, and displays was similar and changed insignificantly with time. Bites were extremely rare. In infected fish, the most significant increase in comparison with the control was noted for bites (more than 20 times) and charges (almost three times), the most energy-consuming categories of behavior. Energy consumption for such behavioral acts increases due to sharp maneuvers and accelerations (Katano and Iguchi, 1996; Cutts et al., 1998). The contribution of less costly behavior (displays and chasing) was much lower during this period. Despite the increased aggressiveness of fish with infective parasites, they almost always lost competition for shelters to less aggressive control fish (Mikheev et al., 2010).
Visual and foraging disturbances in infected fish (Crowden and Broom, 1980; Seppälä et al., 2005b) can reduce their diet and limit resources, especially valuable during periods of intense growth and locomotor activity. However, in our experiments, where fish were fed in excess, we did not observe a decrease in body weight in infected fish compared to controls, which indicates a high efficiency of feeding behavior in infected fish.
A sharp increase in the proportion of fast behavioral acts in the complex of aggressive behavior can increase the visibility of the second intermediate host for the next predator when the parasite is ready to infect the final host. We hypothesize that fast and energy-intensive acts such as charges and biting make infected fish particularly visible to the definitive hosts, piscivorous birds, attacking them from the air (Barber et al., 2000). Slower and longer displays and chasing probably make infected fish more vulnerable to piscivorous fish, which is disadvantageous for the parasite, since its life cycle is then interrupted.
Changes in the aggressive behavior of the rainbow trout underyearlings infected with D. pseudospathaceum metacercariae and their comparison with the behavior of control fish showed that the strategy of manipulating the behavior of the second intermediate host consists of two stages: (1) protecting the host from predators; (2) increasing the vulnerability of the host to predators. At the first stage, the parasite sharply reduces the intensity of all categories of aggressive behavior; at the second stage, the aggressiveness rapidly increases, but the frequency of manifestation of different categories of aggression is different. The proportion of those categories that make the host more visible to the predator increases.
Despite the high level of aggression and the prevalence of the most intense acts of aggression, such as charges and biting, infected fish lose competition for territory and shelter to uninfected ones. In such a situation, high locomotor activity of infected fish contributes to either their rapid consumption by predators or their migration to another habitat. Such migration can also increase the fitness of the parasite, facilitating its dispersal in the water body. The answer to a question whether juvenile salmonids infected with D. pseudospathaceum prefer to stay in their “home” habitat or to move to a new habitat (movers or stayers, according to Grant and Noakes, 1987) needs special experimental studies.
CONCLUSIONS
What does the ability to use different types of aggressive behavior give to fish? This probably helps them to solve vital tasks in various situations that fish face in a heterogeneous and changing environment. The diversity and physiological mechanisms of aggression in animals have long attracted researchers (Moyer, 1968); however studies of these aspects of behavior in fish are relatively rare. This is rather strange, since one of the highly cited papers on the Atlantic salmon Salmo salar behavior, with detailed description of categories of aggressive behavior (Keenleyside and Yamamoto, 1962), appeared more than half a century ago. Even less attention has been paid to the role of parasites in the regulation of aggressive behavior, although their influence on other aspects of fish behavior has long been recognized (Moore, 1995; Barber et al., 2000; Poulin, 2010).
It is known that prone to aggression fish do not always use their maximum capabilities, but quite finely tune their behavior to the situation. So, in the initial period of interactions, for example, in the struggle for a territory, intense acts of aggression in the forms of direct attacks are often; however, after some time, when the fish have explored the environment and social roles have been established, less risky and energy-consuming acts such as frontal and lateral displays prevail (Noleto-Filho et al., 2017).
A parasite inside a fish in order to solve its problems (they are fundamentally different from those of fish) probably does not need to add some new variants of aggressive behavior to the rather rich behavioral repertoire of the host. It is reasonable just to regulate the intensity of already existing behavioral acts, from their complete exclusion (by non-infecrtive parasites) during the protection-from-predators period to differentiated activation (by infective parasites) of those behavioral categories that make hosts hyperactive and aggressive. Such behavior against the background of other fish with normal activity significantly increases their visibility (Landeau and Terborgh, 1986) for the final host.
REFERENCES
Arnott, G. and Elwood, R.W., Assessment of fighting ability in animal contests, Anim. Behav., 2009, vol. 77, no. 5, pp. 991–1004. https://doi.org/10.1016/j.anbehav.2009.02.010
Barber, I., Hoare, D., and Krause, J., Effects of parasites on fish behaviour: A review and evolutionary perspective, Rev. Fish Biol. Fish, 2000, vol. 10, no. 2, pp. 131–165. https://doi.org/10.1023/A:1016658224470
Crowden, A.E. and Broom, D.M., Effects of eyefluke, Diplostomum spathaceum, on the behaviour of dace (Leuciscus leuciscus), Anim. Behav., 1980, vol. 28, no. 1, pp. 287–294. https://doi.org/10.1016/S0003-3472(80)80031-5
Cutts, C., Metcalfe, N.B., and Taylor, A.C., Aggression and growth depression in juvenile Atlantic salmon: The consequences of individual variation in standard metabolic rate, J. Fish. Biol., 1998, vol. 52, no. 5, pp. 1026–1037. https://doi.org/10.1111/j.1095-8649.1998.tb00601
Dianne, L., Perrot-Minnot, M-J., Bauer, A., et al., Protection first then facilitation: A manipulative parasite modulates the vulnerability to predation of its intermediate host according to its own developmental stage, Evolution, 2011, vol. 65, no. 9, pp. 2692–2698. https://doi.org/10.1111/j.1558-5646.2011.01330.x
Earley, R.L. and Hsu, Y., Contest behaviour in fishes, in Animal Contests, Cambridge: Cambridge Univ. Press, 2013, pp. 199–227. https://doi.org/10.1017/CBO9781139051248.012
Grant, J.W.A., Territoriality, in Behavioural Ecology of Teleost Fishes, Oxford: Oxford Univ. Press, 1997, pp. 81–103.
Grant, J.W.A. and Noakes, D.L.G., Movers and stayers: Foraging tactics of young-of-the-year brook charr, Salvelinus fontinalis, J. Anim. Ecol., 1987, vol. 56, no. 3, pp. 1001–1013. https://doi.org/10.2307/4962
Grantner, A. and Taborsky, M., The metabolic rates associated with resting, and with the performance of agonistic, submissive and digging behaviours in the cichlid fish Neolamprologus pulcher (Pisces: Cichlidae), J. Comp. Physiol. B, 1998, vol. 168, no. 6, pp. 427–433. https://doi.org/10.1007/s003600050162
Huntingford, F.A. and Turner, A.K., Animal Conflict, London: Chapman and Hall, 1987. https://doi.org/10.1007/978-94-009-3145-9
Kalleberg, V., Observations in a stream tank of territoriality and competition in juvenile salmon and trout (Salmo salar L. and S. trutta L.), Rep. Inst. Freshw. Res., Drottingholm, 1958, vol. 39, pp. 55–98.
Katano, O. and Iguchi, K., Individual differences in territory and growth of ayu, Plecoglossus altivelis (Osmeridae), Can. J. Zool., 1996, vol. 74, no. 12, pp. 2170–2177. https://doi.org/10.1139/z96-245
Keenleyside, M.H.A. and Yamamoto, F.T., Territorial behaviour of juvenile Atlantic salmon (Salmo salar L.), Behaviour, 1962, vol. 19, nos. 1–2, pp. 139–168. https://doi.org/10.1163/156853961X00231
Lafferty, K.D., The evolution of trophic transmission, Parasitol. Today, 1999, vol. 15, no. 3, pp. 111–115. https://doi.org/10.1016/S0169-4758(99)01397-6
Landeau, L. and Terborgh, J., Oddity and the “confusion effect” in predation, Anim. Behav., 1986, vol. 34, no. 5, pp. 1372–1380. https://doi.org/10.1016/S0003-3472(86)80208-1
Metcalfe, N.B., Intraspecific variation in competitive ability and food intake in salmonids: Consequences for energy budgets and growth rates, J. Fish. Biol., 1986, vol. 28, no. 5, pp. 525–531. https://doi.org/10.1111/j.1095-8649.1986.tb05190.x
Mikheev, V.N., Neodnorodnost’ sredy i troficheskie otnosheniya u ryb (Heterogeneity of the Environment and Trophic Relationships in Fish), Moscow: Nauka, 2006.
Mikheev, V.N., Adams, C.E., Huntingford, F.A., and Thorpe, J.E., Behavioural responses of benthic and pelagic arctic charr to substratum heterogeneity, J. Fish. Biol., 1996, vol. 49, no. 2, pp. 494–500. https://doi.org/10.1111/j.1095-8649.1996.tb00044.x
Mikheev, V.N., Pasternak, A.F., Tischler, G., and Wanzenböck, J., Contestable shelters provoke aggression among 0+ perch, Perca fluviatilis, Environ. Biol. Fish, 2005, vol. 73, no. 2, pp. 227–231. https://doi.org/10.1007/s10641-005-0558-8
Mikheev, V.N., Pasternak, A.F., Taskinen, J., and Valtonen, E.T., Parasite-induced aggression and impaired contest ability in a fish host, Parasites Vectors, 2010, vol. 3, Article 17. https://doi.org/10.1186/1756-3305-3-17
Moore, J., The behaviour of parasitized animals, BioScience, 1995, vol. 45, pp. 89–96. https://doi.org/10.2307/1312610
Moyer, K.E., Kinds of aggression and their physiological basis, Commun. Behav. Biol., 1968, vol. 2, no. 2, pp. 65–87.
Noleto-Filho, E.M., Santos, Gauy A.C., Pennino, M.G., and Goncalves-de-Freitas, E., Bayesian analysis improves experimental studies about temporal patterning of aggressive fish, Behav. Proc., 2017, vol. 145, pp. 18–26. https://doi.org/10.1016/j.beproc.2017.09.017
Parker, G.A., Ball, M.A., Chubb, J.C., et al., When should a trophically transmitted parasite manipulate its host?, Evolution, 2009, vol. 63, no. 2, pp. 448–458. https://doi.org/10.1111/j.1558-5646.2008.00565.x
Poulin, R., Parasite manipulation of host behaviour: An update and frequently asked questions, Adv. Stud. Behav., 2010, vol. 41, pp. 151–186. https://doi.org/10.1016/S0065-3454(10)41005-0
Seppälä, O., Karvonen, A., and Valtonen, E.T., Impaired crypsis of fish infected with a trophically transmitted parasite, Anim. Behav., 2005a, vol. 70, no. 4, pp. 895–900. https://doi.org/10.1016/j.anbehav.2005.01.021
Seppälä, O., Karvonen, A., and Valtonen, E.T., Manipulation of fish host by eye flukes in relation to cataract formation and parasite infectivity, Ibid., 2005b, vol. 70, no. 4, pp. 889–894. https://doi.org/10.1016/j.anbehav.2005.01.020
Shigin, A.A., Trematody fauny SSSR. Rod Diplostomum. Metatserkarii (Trematodes of the Fauna of the USSR. Genus Diplostomum. Metacercariae), Moscow: Nauka, 1986.
Slivko, V.M., Zhokhov, A.E., Gopko, M.V., and Mikheev, V.N., Agonistic behavior of young perch Perca fluviatilis: The effects of fish size and macroparasite load, J. Ichthyol., 2021, vol. 61, no. 3, pp. 476–481. https://doi.org/10.1134/S0032945221030127
Sweeting, R., Investigations into natural and experimental infections of freshwater fish by the common eye-fluke Diplostomum spathaceum Rud, Parasitology, 1974, vol. 69, no. 3, pp. 291–300. https://doi.org/10.1017/s0031182000062995
Vøllestad, L.A. and Quinn, T.P., Trade-off between growth rate and aggression in juvenile coho salmon, Oncorhynchus kisutch, Anim. Behav., 2003, vol. 66, no. 3, pp. 561–568. https://doi.org/10.1006/anbe.2003.2237
Voutilainen, A., Taskinen, J., and Huuskonen, H., Temperature-dependent effect of the trematode eye flukes Diplostomum spp. on the growth of Arctic charr Salvelinus alpinus (L.), Bull. Eur. Ass. Fish Pathol., 2010, vol. 30, no. 3, pp. 106–113.
Weinreich, F., Benesh, D.P., and Milinski, M., Suppression of predation on the intermediate host by two trophically-transmitted parasites when uninfective, Parasitology, 2013, vol. 140, no. 1, pp. 129–135. https://doi.org/10.1017/S0031182012001266
Funding
This work was financially supported by the Russian Foundation for Basic Research (project No. 20-04-00239, processing and analysis of materials), the Russian Science Foundation (project No. 19-14-00015-P, writing the article) and was carried out within the framework of State Order No. FMWE-2021-0007.
Author information
Authors and Affiliations
Corresponding author
Ethics declarations
Conflict of interests. The authors declare that they have no conflicts of interest.
Statement on the welfare of animals. All applicable international, national, and/or institutional guidelines for the care and use of animals were followed.
Additional information
Translated by T. Kuznetsova
Rights and permissions
Open Access. This article is licensed under a Creative Commons Attribution 4.0 International License, which permits use, sharing, adaptation, distribution and reproduction in any medium or format, as long as you give appropriate credit to the original author(s) and the source, provide a link to the Creative Commons license, and indicate if changes were made. The images or other third party material in this article are included in the article’s Creative Commons license, unless indicated otherwise in a credit line to the material. If material is not included in the article’s Creative Commons license and your intended use is not permitted by statutory regulation or exceeds the permitted use, you will need to obtain permission directly from the copyright holder. To view a copy of this license, visit http://creativecommons.org/licenses/by/4.0/.
About this article
Cite this article
Mikheev, V.N., Pasternak, A.F. Structure of Aggressive Behavior in Underyearlings of the Rainbow Trout Oncorhynchus mykiss (Salmonidae) Changes under the Influence of Diplostomum pseudospathaceum (Trematoda) Parasites. J. Ichthyol. 63, 816–821 (2023). https://doi.org/10.1134/S0032945223040136
Received:
Revised:
Accepted:
Published:
Issue Date:
DOI: https://doi.org/10.1134/S0032945223040136