Abstract
Mammalian neuroglobin (Ngb) protects neuronal cells under conditions of oxidative stress. We previously showed that human Ngb acts as a guanine nucleotide dissociation inhibitor (GDI) for the α-subunits of heterotrimeric Gi/o proteins and inhibits the decrease in cAMP concentration, leading to protection against cell death. In the present study, we used an eukaryotic expression vector driving high-level expression of human wild-type Ngb or Ngb mutants that either exhibit or lack GDI activities in human cells. We demonstrate that the GDI activity of human Ngb is tightly correlated with its neuroprotective activity. We further demonstrate that Glu53, Glu60 and Glu118 of human Ngb are crucial for both the neuroprotective activity and interaction with Gαi1. Moreover, we show that Lys46, Lys70, Arg208, Lys209 and Lys210 residues of Gαi1 are important for binding to human Ngb. We propose a molecular docking model of the complex between human Ngb and Gαi1.
Similar content being viewed by others
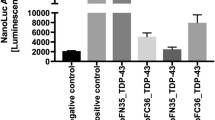
Introduction
Neuroglobin (Ngb) is a globin widely expressed in the brain and which binds reversibly to oxygen (O2)1,2,3,4,5,6. Mammalian Ngb proteins can protect neurons from hypoxic-ischemic insults and protect the brain from experimentally induced stroke in vivo7,8,9,10,11. To investigate the neuroprotective mechanism of human Ngb under conditions of oxidative stress, we previously performed yeast two-hybrid screening using human Ngb as a bait and identified flotillin-1, a lipid raft microdomain-associated protein, as a binding partner12. We demonstrated that human Ngb is recruited to lipid rafts by interacting with flotillin-1 only during oxidative stress and that lipid rafts are crucial for neuroprotection by Ngb11. Moreover, we found that human ferric Ngb, which is generated under oxidative stress conditions, binds exclusively to the GDP-bound form of the α-subunits of heterotrimeric Gi/o proteins (Gαi/o), which are present in lipid rafts and inhibit adenylate cyclase activity13, thereby acting as guanine nucleotide dissociation inhibitor (GDI) for Gαi/o and inhibiting the reduction of intracellular cAMP concentration to protect against cell death10,11,14,15,16.
Although Ngb was originally identified in mammalian species, it is also present in non-mammalian vertebrates17,18. We found that zebrafish Ngb does not exhibit GDI activity and cannot protect mammalian or zebrafish cells against oxidative stress-induced cell death10,15,19,20. In order to identify residues of human Ngb that are crucial for its GDI and neuroprotective activities, we prepared human Ngb mutants with a focus on residues differing between human and zebrafish Ngb and on exposed residues with positive or negative charges on the protein surface15. Protein transfection was achieved by using the protein delivery reagent Chariot, which can efficiently deliver a variety of proteins into several cell lines in a fully biologically active form10,11,16,19,21,22. We showed that human E53Q, R97Q, E118Q and E151N Ngb mutants, which did not function as GDI proteins, did not rescue cell death under oxidative stress conditions10,15, indicating that Glu53, Arg97, Glu118 and Glu151 of human Ngb are crucial residues for its GDI activity and that the GDI activity of human Ngb is tightly correlated with its neuroprotective activity. The analysis using E60Q Ngb mutant revealed that Glu60 is also an essential residue for GDI and neuroprotective activities16. Furthermore, matrix-assisted laser desorption/ionization time-of-flight (MALDI-TOF) mass spectrometry (MS) analysis of tryptic peptides derived from a cross-linked complex between human wild-type (WT) Ngb and Gαi1, which is a member of the Gαi/o family13, revealed cross-linking between Glu60 (Ngb) and Ser206 (Gαi1) and between Glu53 (Ngb) and Ser44 (Gαi1)23.
In the present study, in order to reconfirm the results using the protein transfection reagent “Chariot”, we used an eukaryotic expression vector which can provide the ability for high-level expression of human WT Ngb or Ngb mutants with or without GDI activities in human cells. We demonstrate that the GDI activity of human Ngb is tightly correlated with its neuroprotective activity. We also prepared site-directed mutants of Ngb or Gαi1 and investigated neuroprotective activities and protein-protein interactions by performing glutathione S-transferase (GST) pull-down assays. Moreover, we propose a model of the complex between human Ngb and Gαi1 based on our experimental results.
Results
Neuroprotective and Gαi1-binding assays of E53Q, E60Q, R97Q, E118Q and E151Q Ngb mutants
We used SH-SY5Y cells differentiated into a neuron-like type to investigate the neuroprotective mechanism of human Ngb under oxidative stress conditions. A pcDNA3.1-human WT or Ngb mutant expression vector, or a control vector (pcDNA3.1 empty vector) was transfected into SH-SY5Y cells by Lipofectamine and the protective effects of Ngb proteins against hydrogen peroxide-induced cell death was tested. Expression of human Ngb proteins was confirmed by Western blot analyses (Fig. 1A). Cell viability was measured by using 3-(4,5-dimethylthiazol-2-yl)-5-(3-carboxymethoxyphenyl)-2-(4-sulfophenyl)-2H-tetrazolium, inner salt (MTS). The absorbance at 490 nm was directly proportional to the number of living cells (Supplementary Fig. S1). As shown in Fig. 1B, MTS assays showed that human WT Ngb enhanced cell survival. By contrast, human E53Q, E60Q, R97Q, E118Q and E151N Ngb single mutants, which lack GDI activity, did not protect SH-SY5Y cells against cell death (Fig. 1B). These results are consistent with previous results using the protein transfection reagent “Chariot”10,16. Taken together, we reconfirmed that the GDI activity of human Ngb is tightly correlated with its neuroprotective activity.
Effects of E53Q, E60Q, R97Q, E118Q, or E151N mutation in human Ngb on its neuroprotective activity and interaction with Gαi1.
(A) Western blot analyses of SH-SY5Y cell lysates after transfection. Control vector, human WT or Ngb mutant expression vector was transfected into differentiated SH-SY5Y cells with Lipofectamine. The cells were then incubated for 24 h. Cell lysates were analyzed on 15.0% or 12.5% polyacrylamide-SDS gels and by Western blot analyses using rabbit anti-Ngb polyclonal antibody or mouse anti-β-actin monoclonal antibody, respectively. The arrow indicates the position expected for Ngb or β-actin. Molecular size markers (in kilodaltons) are shown at the left. (B) Effect of the mutation in human Ngb on SH-SY5Y cell death caused by hydrogen peroxide. Differentiated SH-SY5Y cells transfected with control vector, human WT or Ngb mutant expression vector with Lipofectamine were treated with hydrogen peroxide and cell viability was measured by MTS assays. All data are expressed as means ± standard error of means (SEM) from four independent experiments, each carried out in triplicate. Data were analyzed by one-way ANOVA followed by Tukey-Kramer post hoc tests. **P < 0.01. (C) GST pull-down assays of human ferric WT Ngb or Ngb mutant with the GDP-bound truncated Gαi1. GST, GST-human WT Ngb, or GST-human Ngb mutant was incubated with human GDP-bound Gαi1 in a buffer (pH 7.4). Western blot analyses were performed with anti-Gαi1 mouse monoclonal antibody. The arrow indicates the position expected for Gαi1. Molecular size markers (in kilodaltons) are shown at the left.
Next, to characterize the protein-protein interaction between human Ngb and Gαi1 in vitro, we performed GST pull-down assays employing human Ngb fused to GST (GST-human Ngb). We previously demonstrated that GST-human ferric Ngb bound to the GDP-bound form of Gαi111,24. These data are consistent with those obtained by surface plasmon resonance of non-tagged Ngb14, suggesting that the GST tag has no effect on protein-protein interactions between Ngb and Gαi1. In the present study, because the truncated Gαi1 protein, which lacks the N-terminal disordered region (25 amino acids), was more stable than the full-length enzyme25,26, GST-human ferric Ngb, or GST was purified (Supplementary Fig. S2), incubated with human truncated Gαi1 for GST pull-down assays and Western blot analyses were performed using antibody against Gαi1. As shown in Fig. 1C, GST-human WT Ngb bound to the GDP-bound form of the truncated Gαi1. Moreover, we found that human E53Q, E60Q, R97Q, E118Q and E151N Ngb mutants did not, suggesting that Glu53, Glu60, Arg97, Glu118 and Glu151 residues of human Ngb are all indispensable for its interaction with Gαi1.
Analyses based on a possible model of the complex between human Ngb and Gαi1
To gain further insight into the structure of the complex between human Ngb and Gαi1, we created a molecular docking model of the complex based on the following two experimental results: i) Glu53, Glu60, Arg97, Glu118 and Glu151 of human Ngb are all crucial residues for its neuroprotective effect and interaction with Gαi1; ii) Glu53 and Glu60 of human Ngb were cross-linked to Ser44 and Ser206 of Gαi1, respectively23. The molecular docking model was created by manually adjusting the strucutres of Ngb and Gαi1 to avoid steric hindrance between Ngb and Gαi1 and is shown in Fig. 2A.
Effects of R86A, K180L, R208A, K209A, K210A, E236Q, E238N, or E239Q mutation in human Gαi1 on its interaction with Ngb.
(A) A molecular docking modeling of the complex of Ngb and Gαi1. Tertiary structure of human Ngb (Protein Data Bank code: 4MPM) is highlighted in yellow. Residues in human Ngb crucial for its neuroprotective activity are indicated in magenta. Tertiary structure of human Gαi1, which binds to the GoLoco motif of RGS14 (Protein Data Bank code: 1KJY), is highlighted in green. Residues in Gαi1 are indicated in blue. (B,C) GST pull-down assays of human ferric WT Ngb with the GDP-bound form of truncated WT Gαi1, R86A, K180L, or E236Q Gαi1 single mutant, E238N, E239Q Gαi1 double mutant, or R208A, K209A, K210A Gαi1 triple mutant. GDP-bound WT Gαi1 or Gαi1 mutant was incubated with GST-human Ngb or GST in a buffer (pH 7.4). Western blot analyses of the input (B) and pull-down samples (C) were performed with anti-Gαi1 mouse monoclonal antibody. The arrows indicate the positions expected for Gαi1. Molecular size markers (in kilodaltons) are shown at the left.
Next, to evaluate this model, we prepared site-directed Gαi1 mutants at residues with positive or negative charges at the protein binding interface between human Gαi1 and Ngb: R86A, K180L and E236Q Gαi1 single mutants, an E238N, E239Q Gαi1 double mutant and a R208A, K209A, K210A Gαi1 triple mutant. For example, Lys180 of human Gαi1 was mutated to Leu because the corresponding residue of Lys180 of Gαi1 in human Gαs is Leu. An E238N, E239Q Gαi1 double mutant was generated because the corresponding residues of Glu238 and Glu239 of Gαi1 in human Gαs are Asn and Gln, respectively. As shown in Fig. 2B,C, R208A, K209A, K210A Gαi1 triple mutant did not interact with human ferric WT Ngb, whereas R86A, K180L and E236Q Gαi1 single mutants and the E238N, E239Q Gαi1 double mutant bound to Ngb to an extent similar to that of WT Gαi1. These data suggest that Arg208, Lys209, Lys210, but not Arg86, Lys180, Glu236, Glu238, Glu239, of Gαi1 are crucial for the interaction with Ngb and that the binding model in Fig. 2A is not appropriate.
Functional analyses of Ngb mutants prepared on the basis of amino acid sequence alignment among various species of Ngb
We previously reported that Glu53, Arg97, Glu118 and Glu151 of human Ngb are conserved only among boreoeutheria of mammalia and not among afrotheria, maetatheria, prototheria, aves, reptillia, amphibia, or osteichtyes27, suggesting that boreoeutheria Ngb proteins may protect neurons against oxidative stress-induced cell death. In the present study, we performed more detailed sequence comparisons by using sequence data of Ngb proteins from a greater number of species. As shown in Fig. 3A and Supplementary Fig. S3, the residue corresponding to Arg97 of human Ngb is a Cys in megabat Ngb. And the residue corresponding to Glu151 of human Ngb is a Gln in bush baby Ngb (Fig. 3A and Supplementary Fig. S3). Therefore, we created human R97C and E151Q Ngb mutants and investigated their structure, neuroprotective activity and interaction with Gαi1.
Effects of R97C or E151Q mutation in human Ngb on its structure, GDI and neuroprotective activities.
(A) Partial sequence alignment among human, bush baby, mouse, megabat, zebrafish and fugu Ngb proteins. Identical residues to human Ngb are highlighted in yellow. Numbers above the sequences correspond to those of the residues of human Ngb. (B) Electronic absorption spectra of the ferric form of human WT Ngb (bold line), R97C Ngb (fine line) and E151Q Ngb (dotted line). (C) CD spectra in the far UV region of the ferric form of human WT Ngb (bold line), R97C Ngb (fine line) and E151Q Ngb (dotted line). (D) Western blot analyses of SH-SY5Y cell lysates after transfection. Control vector, human WT or Ngb mutant expression vector was transfected into differentiated SH-SY5Y cells with Lipofectamine. The cells were then incubated for 24 h. Cell lysates were analyzed on 15.0% or 12.5% polyacrylamide-SDS gels and by Western blot analyses using rabbit anti-Ngb polyclonal antibody or mouse anti-β-actin monoclonal antibody, respectively. The arrow indicates the position expected for Ngb or β-actin. Molecular size markers (in kilodaltons) are shown at the left. (E) Effect of the mutation in human Ngb on SH-SY5Y cell death caused by hydrogen peroxide. Differentiated SH-SY5Y cells transfected with control vector, human WT or Ngb mutant expression vector with Lipofectamine were treated with hydrogen peroxide and cell viability was measured by MTS assay. All data are expressed as means ± SEM from four independent experiments, each carried out in triplicate. Data were analyzed by one-way ANOVA followed by Tukey-Kramer post hoc tests. **P < 0.01. (F) GST pull-down assays of the human Ngb mutant with GDP-bound Gαi1. GST, GST-human WT Ngb, or GST-human Ngb mutant was incubated with human GDP-bound Gαi1 in a buffer (pH 7.4). Western blot analyses were performed with anti-Gαi1 mouse monoclonal antibody. The arrow indicates the position expected for Gαi1. Molecular size markers (in kilodaltons) are shown at the left. (G) The effect of the R97C or E151Q mutation on dissociation of GDP from human GDP-bound Gαi1. The amount of [3H]GDP bound to Gαi1 in the absence of human ferric Ngb at 0 min was defined as 100%. All data are expressed as means ± SEM from three independent experiments. Data were analyzed by one-way ANOVA followed by Tukey-Kramer post hoc tests. *P < 0.05, **P < 0.01.
Initially, we evaluated the effects of the R97C or E151Q mutation on the electronic state of the heme group by measuring the absorption spectra. As shown in Fig. 3B and Table 1, the wavelength of the Soret peak of human ferric R97C or E151Q Ngb was the same as those of human ferric WT Ngb, demonstrating that the R97C or E151Q mutation of human Ngb did not perturb the electric state of the heme group. Next, to examine the effect of the R97C or E151Q substitution upon secondary structure, we measured the far-UV circular dichroism (CD) spectra. As shown in Fig. 3C, human WT, R97C and E151Q Ngb proteins exhibited two negative broad peaks around 222 and 208 nm, which are characteristic of an α-helical structure. The α-helical contents of the R97C and E151Q Ngb proteins were estimated to be 68.9% and 73.4%, which is almost identical to that of human WT Ngb (71.4%) (Table 1). These results showed that the secondary protein structure is not affected by the amino acid substitution.
Next, we tested whether R97C and E151Q Ngb can protect SH-SY5Y cells against oxidative stress. We first confirmed that human WT, R97C and E151Q Ngb proteins were expressed at a similar level in SH-SY5Y cells by Western blot analyses (Fig. 3D). MTS assays showed that the expression of human R97C or E151Q Ngb mutant in the cells rescued cell death under oxidative stress condition, as did that of human WT Ngb (Fig. 3E). Moreover, GST-human ferric Ngb, or GST was purified (Supplementary Fig. S2), incubated with human truncated Gαi1 and Western blot analyses were performed using antibody against Gαi1. As shown in Fig. 3F, GST pull-down assays showed that R97C and E151Q Ngb bound to the truncated Gαi1 as did the WT Ngb. Furthermore, to examine the effect of the R97C or E151Q mutation of human Ngb upon the release of GDP from Gαi1, we measured the rates of GDP dissociation in the absence or presence of human Ngb. In the presence of an excess amount of unlabeled GTP, [3H]GDP release from [3H]GDP-bound Gαi1 was inhibited by human ferric WT Ngb (Fig. 3G). As shown in Fig. 3G, human ferric R97C and E151Q Ngb functioned as the GDI for Gαi1 as did human WT Ngb. These results suggest that the positive charge of Arg97 and the negative charge of Glu151 of human Ngb are not essential for the GDI and neuroprotective activities. Taken together, we conclude that Glu53, Glu60 and Glu118 of human Ngb are the only crucial residues for its activities.
Search for residues of Gαi1 crucial for the interaction with human Ngb
Next, because we found that Arg97 and Glu151 residues of Ngb are not involved in the interface with Gαi1, we created a new molecular docking model of the complex between human Ngb and Gαi1, which is depicted in Fig. 4A. To clarify binding sites in the complex between human Ngb and Gαi1, we prepared K46A, K67A and K70A Gαi1 single mutants. As shown in Fig. 4B,C, K46A and K70A Gαi1 single mutants did not interact with Ngb. Moreover, the R208A, K209A, K210A Gαi1 triple mutant also did not interact with Ngb (Fig. 2B,C). These results suggest that the model of Fig. 4A is correct. Moreover, K67A Gαi1 bound to Ngb as did WT Gαi1 (Fig. 4B,C), indicating that Glu118 of human Ngb interacts with Lys70, but not Lys67, of Gαi1 in a site-specific manner.
Effects of K46A, K67A, or K70A mutation in human Gαi1 on its interaction with Ngb.
(A) A molecular docking model of the complex of Ngb and Gαi1. Tertiary structure of human Ngb (Protein Data Bank code: 4MPM) is highlighted in yellow. Residues in human Ngb crucial for its neuroprotective activity are indicated in magenta. Tertiary structure of human Gαi1, which binds to the GoLoco motif of RGS14 (Protein Data Bank code: 1KJY), is highlighted in green. Residues in Gαi1 are indicated in blue. (B,C) GST pull-down assays of human ferric WT Ngb with the GDP-bound form of truncated WT Gαi1, K46A, K67A, or K70A Gαi1 single mutant. GDP-bound form of WT Gαi1 or Gαi1 mutant was incubated with GST-human Ngb or GST in a buffer (pH 7.4). Western blot analyses of the input (B) and pull-down samples (C) were performed with anti-Gαi1 mouse monoclonal antibody. The arrows indicate the positions expected for Gαi1. Molecular size markers (in kilodaltons) are shown at the left.
Discussion
In the present study, by using eukaryotic expression vectors for human Ngb proteins we demonstrated that the GDI activity of human Ngb is tightly correlated with its neuroprotective activity. GST pull-down assays using GST-fused human Ngb demonstrated that Glu53, Glu60, Arg97, Glu118 and Glu151 residues of human Ngb are all indispensable for its interaction with Gαi1. However, R97C and E151Q Ngb single mutants, generated based on sequence alignment among various species of Ngb, showed that the positive charge of Arg97 and the negative charge of Glu151 of human Ngb are dispensable for both the GDI and neuroprotective activities. We therefore speculated that the lack of GDI and neuroprotective activities of the R97Q and E151N Ngb mutants may arise from structural alterations induced by the mutations and concluded that only the negative charges of Glu53, Glu60 and Glu118 of human Ngb are crucial for the GDI and neuroprotective activities. We measured the UV-visible and CD spectra of the WT and mutants but could not detect any significant structural differences among them. Further studies are in progress to clarify structural alterations between the WT and R97Q or E151N Ngb mutant.
Moreover, we identified residues of Gαi1 crucial for the interaction with human Ngb; Lys46, Lys70, Arg208, Lys209 and Lys210 residues of Gαi1 are important for its binding to human Ngb. The present results imply that electrostatic interactions between the negative charges of Glu53, Glu60 and Glu118 of human Ngb and the positive charges of Lys46, Lys70, Arg208, Lys209, and/or Lys210 of Gαi1 are crucial for the formation of a complex between Ngb and Gαi1. This is consistent with the previous observation that electrostatic complementarity is an important factor for the interaction of Gα with its regulator28. Further more detailed studies are in progress to investigate the significance of Arg208, Lys209, and/or Lys210 of Gαi1. Moreover, because the residue corresponding to Arg97 of human Ngb is a Gln in yak Ngb (Supplementary Fig. S3), it is interesting to investigate whether yak Ngb has the GDI and neuroprotective activities.
The present results support that a molecular docking model of the complex between human Ngb and Gαi1 in Fig. 4A. In this model, Glu60 (Ngb), which was cross-linked to Ser206 (Gαi1) by chemical cross-linking23, interacts with Arg208, Lys209, and/or Lys210 of Gαi1. The amino acid sequence surrounding Glu60 in human Ngb has a motif homologous to those of the R6A-1 peptide and the KB-752 peptide, which interact with GDP-bound Gαi116. It has previously been reported that the R6A-1 and KB-752 peptides interact with the switch II (a.a. 199–219) of Gαi126,29,30. The molecular docking model of the complex between human Ngb and Gαi1 shows that Glu60 of human Ngb is located near the switch II of Gαi1, as are R6A-1 and KB-752 peptides, suggesting that the motif including Glu60 in human Ngb functions as the core motif for the binding with Gαi1.
As shown in Fig. 4A, Glu53 (Ngb), which was cross-linked to Ser44 (Gαi1) by chemical cross-linking23, binds to Lys46 of Gαi1. X-ray structural data of Gαi1 show that Ser44 and Lys46 of Gαi1 are located in the vicinity of GDP25. This implies that Ngb could be positioned near the GDP-binding site in the Ngb-Gαi complex, which would inhibit the dissociation of GDP from the binding site. Moreover, it should be also noted that Glu53 of Ngb is conserved among boreotheria except for the harp or hooded seal (Supplementary Fig. S3). In seals Ngb is mainly localized in astrocytes, whereas other boreotheria Ngb exists in neuron31,32. The different localization of Ngb suggests that the function of seal Ngb may be different from that of other boreotheria Ngb.
Figure 4A shows that Glu118 of Ngb interacts with Lys70 of Gαi1. We previously carried out chemical cross-linking of the Ngb and Gαi1 complex by treatment with zero-length cross-linkers to identify the sites of interaction between Ngb and Gαi123. In the peptide map of tryptic peptides derived from the cross-linked Ngb-Gαi1 complex, the MS peak of a Ngb peptide (amino acids 103–119 of Ngb), which was observed in the tryptic peptide map of Ngb, was missing or significantly decreased in intensity23, suggesting that the 103–119 amino acid residues of Ngb are involved in the Ngb-binding site for Gαi1, but information about the detailed residues cross-linked in the complex of Ngb and Gαi1 remained unclear. The present results suggest that Glu118, which is located in the 103–119 region, of human Ngb interacts with Lys70 residue, which is located in the α-helical domain of Gαi1, as shown in Fig. 4A. Strikingly, crystal structure of GoLoco motifs of LGN protein, which act as GDI for Gαi1, elucidated that they interact with the switch II region and Tyr69 and Val72, which are close to Lys70, in the α-helical domain of Gαi133. It is also worth noting that RGS domains, which bind to Gαi selectively, interact with some residues in the α-helical domain of Gα as well as the switch regions28.
Lys46, Arg208, Lys209 and Lys210 residues, which are crucial for the interaction with Ngb and their sequential neighborhoods of Gαi1 are conserved among Gαi/o and Gαs. In contrast, Lys70 residue of Gαi1 is conserved among Gαi/o, but corresponds to Val in Gαs based on sequence alignment among members of the Gα family13. Moreover, sequential neighborhoods around Lys70 of Gαi/o are very different from those of Gαs. Therefore, the positive charge of the 70th residue of Gα, which interacts with the negative charge of carboxylic group of Glu118 through electrostatic interaction, may be crucial for the Gαi/o–specific binding property of Ngb.
Methods
Cell culture
SH-SY5Y cells (CRL-2266) were obtained from the American Type Culture Collection (ATCC; Manassas, VA) and maintained in a 1:1 mixture of Dulbecco’s modified Eagle’s medium and Ham’s F-12 nutrient mixture containing 2.5 mM glutamine, supplemented with 10% (v/v) fetal bovine serum, 100 U/ml penicillin and 100 μg/ml streptomycin (all from Invitrogen, Carlsbad, CA) in a humidified atmosphere containing 5% CO2 at 37 °C. The medium was changed every 4 days and the cultures were split at a 1:20 ratio once a week. Cultured cells were induced to differentiate into a neuronal phenotype by treatment with 10 μM retinoic acid (Sigma-Aldrich, St. Louis, MO) over a period of 6 days (media were exchanged every 3 days during sub-culture). Differentiation was verified by monitoring macroscopic changes to the cells.
Transfection of Ngb expression vector into SH-SY5Y cells and treatment of cells with hydrogen peroxide
The eukaryotic expression vector pcDNA3.1 (Invitrogen) for human Ngb was prepared as described previously11. A QuikChangeTM site-directed mutagenesis system (Stratagene, La Jolla, CA) was used for site-directed mutagenesis and the constructs were confirmed by DNA sequencing (FASMAC Co., Ltd., DNA sequencing services, Atsugi, Japan). Differentiated SH-SY5Y cells were plated on poly-D-lysine coated 96-well plates at a density of 5.0 × 105 cells/mL for 24 h. The pcDNA3.1-human WT or Ngb mutant expression vector or control vector (pcDNA3.1 empty vector) was transfected by using LipofectamineTM 2000 (Invitrogen) according to the manufacturer’s instructions. After 24 h of transfection, hydrogen peroxide (100 μM) was added and cells were incubated for 24 h.
Western blot analyses
After cell lysates were resolved by electrophoresis on polyacrylamide-SDS gels, proteins were electroblotted onto Hybond-P PVDF membranes (GE Healthcare Biosciences, Piscataway, NJ), which were then blocked with phosphate-buffered saline (PBS) and 5% skim milk (Wako Pure Chemical Industries, Osaka, Japan) and incubated with rabbit anti-Ngb (FL-151) polyclonal antibody (Santa Cruz Biotechnology, Santa Cruz, CA), or mouse anti-β-actin monoclonal antibody (Sigma-Aldrich). After washing, membranes were incubated with an HRP-linked F(ab′)2 fragment of donkey anti-rabbit IgG or an HRP-linked whole antibody of sheep anti-mouse IgG (GE Healthcare Biosciences). Proteins were visualized using ECLTM Western blotting detection reagents (GE Healthcare Biosciences). Chemiluminescent signals were detected using a LAS-4000 mini luminescent image analyzer (GE Healthcare Biosciences).
Cell viability assay
Cell viability was measured with the CellTiter 96® AQueous One Solution Cell Proliferation Assay Reagent (Promega, Madison, WI), containing [3-(4,5-dimethylthiazol-2-yl)-5-(3-carboxymethoxyphenyl)-2-(4-sulfophenyl)-2H-tetrazolium, inner salt; MTS]. Cultured cells were incubated with the MTS reagent at 37 °C for 4 h in a humidified, 5% CO2 atmosphere. The amount of colored formazan dye formed was then quantified by measuring absorbance at 490 nm with a Beckman Coulter DTX880 plate reader (Beckman Coulter, Fullerton, CA).
Preparation and purification of GST and a fusion protein of GST and Ngb
Human Ngb cDNA was cloned into the pGEX-4T-1 vector (GE Healthcare Biosciences) to produce the fusion protein GST-Ngb11,24. A QuikChangeTM site-directed mutagenesis system (Stratagene) was used for site-directed mutagenesis. The constructs were confirmed by DNA sequencing (FASMAC Co., Ltd., DNA sequencing services). Overexpression of GST-Ngb and GST alone (as a control) was induced in the Escherichia coli strain BL21 (DE3) (Novagen, Madison, WI) by treatment with isopropyl-β-D-thiogalactopyranoside (IPTG) for 4 h. Both GST-ferric Ngb and GST were purified by using glutathione-Sepharose 4B beads (GE Healthcare Biosciences) according to the manufacturer’s instructions.
Preparation of recombinant human truncated Gαi1 protein
The DNA fragment containing the human truncated Gαi1 subunit (residues 26–354) was amplified by PCR and cloned into the pET151/D-TOPO® vector (Invitrogen) to be expressed as human WT truncated Gαi1 protein (residues 26–354) fused to a TEV protease recognition site directly after an N-terminal tag of six histidine residues (His6-tag). A QuikChangeTM site-directed mutagenesis system (Stratagene) was used for site-directed mutagenesis. The constructs were confirmed by DNA sequencing (FASMAC Co., Ltd., DNA sequencing services). The resulting Gαi1 was expressed in E. coli strain BL21 (DE3) by induction with IPTG and purified by using a nickel affinity column (His·Bind® resin; Novagen), as described previously11. Then, the sample was incubated with His6-tagged TEV protease (MoBiTec GmbH, Göttingen, Germany) and loaded onto a His·Bind® column to separate the cleaved Gαi1 from the cleaved His6-tag, any uncleaved protein and His6-tagged TEV protease, as described previously11.
GST pull-down assays using truncated Gαi1
Truncated Gαi1 was incubated with either GST alone or GST–ferric Ngb immobilized on glutathione-Sepharose 4B beads (GE Healthcare Biosciences) in HEPES buffer (10 mM HEPES, 150 mM NaCl, 10 mM MgCl2, 10 μM GDP, 0.1% Tween20, pH 7.4) for 1 h at 4 °C. The beads were washed extensively three times with the buffer and the samples were then resuspended in Laemmli sample buffer, heated for 5 min at 95 °C and separated on 12.0% polyacrylamide-SDS gels. For Western blot analyses, the proteins were transferred onto Hybond-P PVDF membranes (GE Healthcare Biosciences), which were then blocked with PBS and 5% skim milk (Wako Pure Chemical Industries) and incubated with mouse anti-Gαi1 (Ab-3; clone R4.5) monoclonal antibody (Thermo Fisher Scientific, Fremont, CA). After washing, the membranes were incubated with an HRP-linked whole antibody of sheep anti-mouse IgG (GE Healthcare Biosciences). Proteins were visualized using ECLTM western blotting detection reagents (GE Healthcare Biosciences). Chemiluminescent signals were detected using a LAS-4000 mini luminescent image analyzer (GE Healthcare Biosciences).
Preparation of non-tagged recombinant human Ngb proteins
Plasmids for human Ngb were prepared as described previously14,15. A QuikChangeTM site-directed mutagenesis system (Stratagene) was used for site-directed mutagenesis. The constructs were confirmed by DNA sequencing (FASMAC Co., Ltd., DNA sequencing services). Overexpression of each Ngb was induced in E. coli strain BL 21 (DE 3) by treatment with IPTG for 4 h and each Ngb protein was purified as described previously10,11,14,15,16. Briefly, soluble cell extracts were loaded onto DEAE sepharose anion-exchange columns equilibrated with buffer A (20 mM Tris-HCl, pH 8.0). Ngb proteins were eluted from columns with buffer A containing 150 mM NaCl and further purified by passage through Sephacryl S-200 HR gel filtration columns. The protein concentration of human ferric Ngb was determined spectrophotometrically using an extinction coefficient of 122 mM−1cm−1 at the Soret peak.
UV-visible spectra
Electronic absorption spectra of purified proteins were recorded with a UV-visible spectrophotometer (UV-2450; Shimadzu, Kyoto, Japan) at ambient temperature (~20 °C). Spectra were recorded in PBS (pH 7.4).
CD spectra
CD spectra in the far-UV region were measured with a spectropolarimeter (J-805; JASCO Co., Tokyo, Japan) at 20 °C. The samples were measured at a concentration of approximately 5 μM in 50 mM sodium phosphate buffer (pH 7.4). The path length of the cells used for the measurements was 1 mm. The molar ellipticity (deg cm2 dmol−1) was determined on the mean residue basis. The α-helix content (fH) was calculated according to Chen et al.34 by the following equation:

[3H]GDP dissociation assays
GDP dissociation assays were performed, as described previously11,16. In brief, Gαi1 complexed with [3H]GDP (0.3 μM) was prepared by incubating 0.3 μM Gαi1 with 2 μM [8,5′-3H]GDP (PerkinElmer Life Sciences, Boston, MA) in buffer B [20 mM Tris-HCl, 100 mM NaCl and 10 mM MgSO4 at pH 8.0] for 1.5 h at 25 °C. Excess unlabeled GTP (2 mM) was added to monitor dissociation of [3H]GDP from Gαi1 in the absence or presence of non-tagged ferric Ngb (10 μM). Aliquots were withdrawn at 0, 5 and 10 min and were passed through nitrocellulose filters (0.45 μm) (Advantec Toyo, Tokyo, Japan). The filters were then washed three times with 1 ml of ice-cold buffer B and were counted in a liquid scintillation counter (LS6500; Beckman Coulter).
Additional Information
How to cite this article: Takahashi, N. and Wakasugi, K. Identification of residues crucial for the interaction between human neuroglobin and the α-subunit of heterotrimeric Gi protein. Sci. Rep. 6, 24948; doi: 10.1038/srep24948 (2016).
References
Burmester, T., Weich, B., Reinhardt, S. & Hankeln, T. A vertebrate globin expressed in the brain. Nature 407, 520–523 (2000).
Dewilde, S. et al. Biochemical characterization and ligand binding properties of neuroglobin, a novel member of the globin family. J. Biol. Chem. 276, 38949–38955 (2001).
Trent, J. T. 3rd, Watts, R. A. & Hargrove, M. S. Human neuroglobin, a hexacoordinate hemoglobin that reversibly binds oxygen. J. Biol. Chem. 276, 30106–30110 (2001).
Pesce, A. et al. Neuroglobin and cytoglobin: Fresh blood for the vertebrate globin family. EMBO Rep. 3, 1146–1151 (2002).
Hankeln, T. et al. Neuroglobin and cytoglobin in search for their role in the vertebrate globin family. J. Inorg. Biochem. 99, 110–119 (2005).
Greenberg, D. A., Jin, K. & Khan, A. A. Neuroglobin: an endogenous neuroprotectant. Curr. Opin. Pharmacol. 8, 1–5 (2007).
Sun, Y., Jin, K., Mao, X. O., Zhu, Y. & Greenberg, D. A. Neuroglobin is up-regulated by and protects neurons from hypoxic-ischemic injury. Proc. Natl. Acad. Sci. USA 98, 15306–15311 (2001).
Sun, Y. et al. Neuroglobin protects the brain from experimental stroke in vivo. Proc. Natl. Acad. Sci. USA 100, 3497–3500 (2003).
Khan, A. A. et al. Neuroglobin-overexpressing transgenic mice are resistant to cerebral and myocardial ischemia. Proc. Natl. Acad. Sci. USA 103, 17944–17948 (2006).
Watanabe, S. & Wakasugi, K. Neuroprotective function of human neuroglobin is correlated with its guanine nucleotide dissociation inhibitor activity. Biochem. Biophys. Res. Commun. 369, 695–700 (2008).
Watanabe, S., Takahashi, N., Uchida, H. & Wakasugi, K. Human neuroglobin functions as an oxidative stress-responsive sensor for neuroprotection. J. Biol. Chem. 287, 30128–30138 (2012).
Wakasugi, K., Nakano, T., Kitatsuji, C. & Morishima, I. Human neuroglobin interacts with flotillin-1, a lipid raft microdomain-associated protein. Biochem. Biophys. Res. Commun. 318, 453–460 (2004).
Gilman, A. G. G proteins: transducers of receptor-generated signals. Annu. Rev. Biochem. 56, 615–649 (1987).
Wakasugi, K., Nakano, T. & Morishima, I. Oxidized human neuroglobin as a heterotrimeric Gα protein guanine nucleotide dissociation inhibitor. J. Biol. Chem. 278, 36505–36512 (2003).
Wakasugi, K. & Morishima, I. Identification of residues in human neuroglobin crucial for guanine nucleotide dissociation inhibitor activity. Biochemistry 44, 2943–2948 (2005).
Takahashi, N., Watanabe, S. & Wakasugi, K. Crucial roles of Glu60 in human neuroglobin as a guanine nucleotide dissociation inhibitor and neuroprotective agent. PLoS ONE 8, e83698 (2013).
Awenius, C., Hankeln, T. & Burmester, T. Neuroglobins from the zebrafish Danio rerio and the pufferfish Tetraodon nigroviridis. Biochem. Biophys. Res. Commun. 287, 418–421 (2001).
Fuchs, C. et al. Zebrafish reveals different and conserved features of vertebrate neuroglobin gene structure, expression pattern and ligand binding. J. Biol. Chem. 279, 24116–24122 (2004).
Watanabe, S. & Wakasugi, K. Zebrafish neuroglobin is a cell-membrane-penetrating globin. Biochemistry 47, 5266–5270 (2008).
Kamioka, Y. et al. Functional characterization of fish neuroglobin: zebrafish neuroglobin is highly expressed in amacrine cells after optic nerve injury and can translocate into ZF4 cells. Biochim. Biophys. Acta 1834, 1779–1788 (2013).
Morris, M. C., Depollier, J., Mery, J., Heitz, F. & Divita, G. A peptide carrier for the delivery of biologically active proteins into mammalian cells. Nat. Biotechnol. 19, 1173–1176 (2001).
Wakasugi, K., Takahashi, N. & Watanabe, S. Chimeric ZHHH neuroglobin is a novel cell membrane-penetrating, neuroprotective agent. Am. J. Neuroprotec. Neuroregen. 3, 42–47 (2011).
Kitatsuji, C., Kurogochi, M., Nishimura, S., Ishimori, K. & Wakasugi, K. Molecular basis of guanine nucleotide dissociation inhibitor activity of human neuroglobin by chemical cross-linking and mass spectrometry. J. Mol. Biol. 368, 150–160 (2007).
Wakasugi, K., Kitatsuji, C. & Morishima, I. Possible neuroprotective mechanism of human neuroglobin. Ann. NY Acad. Sci. 1053, 220–230 (2005).
Kimple, R. J., Kimple, M. E., Betts, L., Sondek, J. & Siderovski, D. P. Structural determinants for GoLoco-induced inhibition of nucleotide release by Galpha subunits. Nature 416, 878–881 (2002).
Johnston, C. A. et al. Structure of Gαi1 bound to a GDP-selective peptide provides inshight into guanine nucleotide exchange. Structure 13, 1069–1080 (2005).
Wakasugi, K., Takahashi, N., Uchida, H. & Watanabe, S. Species-specific functional evolution of neuroglobin. Mar. Genomics 4, 137–142 (2011).
Baltoumas, F. A., Theodoropoulou, M. C. & Hamodrakas, S. J. Interactions of the α-subunits of heterotrimeric G-proteins with GPCRs, effectors and RGS proteins: a critical review and analysis of interacting surfaces, conformational shifts, structural diversity and electrostatic potentials. J. Struct. Biol. 182, 209–218 (2013).
Ja, W. W., Adhikari, A., Austin, R. J., Sprang, S. R. & Roberts, R. W. A peptide core motif for binding to heterotrimeric G protein α subunits. J. Biol. Chem. 280, 32057–32060 (2005).
Willard, F. S. & Siderovski, D. P. The R6A-1 peptide binds to switch II of Gαi1 but is not a GDP-dissociation inhibitor. Biochem. Biophys. Res. Commun. 339, 1107–1112 (2006).
Mitz, S. A. et al. When the brain goes diving: glial oxidative metabolism may confer hypoxia tolerance to the seal brain. Neuroscience 163, 552–560 (2009).
Schneuer, M. et al. Neuroglobin of seals and whales: evidence for a divergent role in the diving brain. Neuroscience 223, 35–44 (2012).
Jia, M. et al. Crystal structures of the scaffolding protein LGN reveal the general mechanism by which GoLoco binding motifs inhibit the release of GDP from Gαi. J. Biol. Chem. 287, 36766–36776 (2012).
Chen, Y. H., Yang, J. T. & Martinez, H. M. Determination of the secondary structures of proteins by circular dichroism and optical rotatory dispersion. Biochemistry 11, 4120–4131 (1972).
Acknowledgements
This work was supported in part by research grants from the Fuji Foundation for Protein Research (to K.W.), the Institute for Fermentation, Osaka, Japan (to K. W.), the Naito Foundation (to K.W.), the Narishige Zoological Science Award (to K. W.) and the Yamazaki Spice Promotion Foundation (to K. W.) and a Grant-in-Aid for Scientific Research (C) (No. 26440047) (to K.W.) from the Ministry of Education, Culture, Sports, Science and Technology of Japan.
Author information
Authors and Affiliations
Contributions
N.T. and K.W. designed the study and wrote the paper. N.T. performed the experiments. N.T. and K.W. analyzed the results and approved the final version of the manuscript.
Ethics declarations
Competing interests
The authors declare no competing financial interests.
Electronic supplementary material
Rights and permissions
This work is licensed under a Creative Commons Attribution 4.0 International License. The images or other third party material in this article are included in the article’s Creative Commons license, unless indicated otherwise in the credit line; if the material is not included under the Creative Commons license, users will need to obtain permission from the license holder to reproduce the material. To view a copy of this license, visit http://creativecommons.org/licenses/by/4.0/
About this article
Cite this article
Takahashi, N., Wakasugi, K. Identification of residues crucial for the interaction between human neuroglobin and the α-subunit of heterotrimeric Gi protein. Sci Rep 6, 24948 (2016). https://doi.org/10.1038/srep24948
Received:
Accepted:
Published:
DOI: https://doi.org/10.1038/srep24948
- Springer Nature Limited
This article is cited by
-
Neuroglobin Expression in the Brain: a Story of Tissue Homeostasis Preservation
Molecular Neurobiology (2019)
-
Investigating molecular interactions between oxidized neuroglobin and cytochrome c
Scientific Reports (2018)