Abstract
Clinacanthus nutans (Burm. f.) Lindau has been extensively utilized in Thai folk medicine. However, there has been no prior exploration of its genetic diversity or its correlation with biological activity and phytochemical profiles. Herein, a total of 10 samples of C. nutans were collected from different geographic locations in different environments of Thailand, encompassing Northern, Northeastern, and Central regions. The genetic diversity study using sequence-related amplified polymorphism (SRAP) markers showed that all C. nutans samples were closely related, as indicated by UPGMA cluster analysis. When comparing the biological activities of C. nutans extracts, our findings demonstrated that those sourced from Northern Thailand exhibited the most potent activity in reducing lipopolysaccharide-inducing cell death, as accessed by cell viability assay. Furthermore, they showed remarkable antioxidant and antibacterial activities against Staphylococcus epidermidis, Staphylococcus aureus, Pseudomonas aeruginosa, and Escherichia coli. High-performance liquid chromatography (HPLC) analysis of phytochemical profiles revealed consistent chromatography peak patterns across all C. nutans extracts. However, they exhibited varying levels of phenolic contents, as judged by the Folin-Ciocalteu assay, which positively correlated with their observed activities. In conclusion, this study highlights the limited genetic variation within C. nutans population in Thailand. Furthermore, it underscores the association between the biological activity and the total phenolic contents which might be mainly impacted by environmental conditions.
Similar content being viewed by others
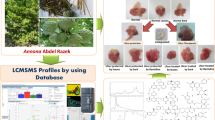
Introduction
Natural products derived from various sources such as plants, animals, and fungi have been used in therapeutic approaches for treating several diseases in folk medicine for more than centuries. The bioactive substances originating from herbs and medicinal plants have been continuously receiving great attention and made substantial contributions to modern medicine1,2. These compounds, metabolites, and secondary metabolites potentially serve as starting materials for drug synthesis2. To date, bioactive ingredients derived from medicinal plants account for approximately 25% of prescription pharmaceuticals, especially as anti-microbial and anti-cancer agents.3.
Southeast Asia is a well-known hotspot for plant diversity richness according to the diverse climate of this region4. Local communities have long utilized herbs and medicinal plants for therapeutic purposes since ancient times revealing the potential of traditional medicine and its application in modern pharmaceutical research and development (R&D)2. The Acanthaceae family, found mainly in tropical and subtropical regions across Southeast Asia, is among the most diverse plant families, containing over 4,300 species3. Clinacanthus nutans (C. nutans) Lindau belonging to the Acanthaceae family has traditionally been employed for treating conditions such as skin rashes, insect bites, and diabetes mellitus in Indonesia, Malaysia, and Thailand5. Notably, C. nutans is included in Thailand's national list of essential herbal medicines (NLEM) (2016) and is recommended for treating aphthous ulcers, insect bites and herpes simplex virus, varicella-zoster virus infections. Currently, extensive research has unveiled the pharmacological activities of C. nutans extract including antioxidant, anti-inflammatory, anti-diabetic, anti-bacterial (i.e., Bacillus cereus, Escherichia coli, Salmonella enterica)6 and anti-virus activities (i.e., herpes simplex virus7,8, varicella-zoster virus9, dengue virus10). However, despite these insights into its biological activity, only a limited number of studies have investigated the genetic diversity of C. nutans whereas its correlation to the phytochemical profile and biological activity has never been explored.
Understanding the genetic diversity and its impact on the bioactivity of plant extracts is important for crop development. Genetic markers linked to quantitative traits and biological activity can serve as selection markers for plant breeders to improve the productivity and pharmacological properties of the plant11. Therefore, this study aims to investigate the relationship between the genetic diversity of C. nutans collected from the different regions and their biological activities. We investigated the genetic diversity of C. nutans samples from the different regions with various climate environments in Thailand including Northern, Northeastern, and Central Thailand. Ten samples were investigated and compared for their biological activities including anti-cell death activity against LPS-induced endothelial cell death, antioxidant activity, and antibacterial activity. Additionally, we compared the phytochemical profiles and phenolic contents of C. nutans extracts. This comprehensive understanding of the genetic profile and its correlation with biological function would contribute to the promotion, enhancement, and sustainable conservation of C. nutans for therapeutic and commercial applications.
Results
Genetic diversity of C. nutans in Thailand
Genetic diversity greatly contributed to the bioactivity of the plant extract, particularly in relation to the content of bioactive compounds. We collected the C. nutans samples from 10 different regions, including 5 regions in Northern Thailand, 4 regions in the Northeastern part of the country, and 1 region in Central Thailand, each exhibiting variations in either geographic locations or environmental conditions (Table 1). The assessment of genetic diversity was determined using the SRAP technique, coupled with UPGMA cluster analysis. Seven pairs of SRAP markers revealed consistent patterns among C. nutans samples from 10 regions. Notably, these patterns were remarkably different from the outgroup, which included Phlogacanthus pulcherrimus and Ruellia tuberosa, both of which belong to the Acanthaceae family, like C. nutans (Fig. 1A, with a full-length gel provided in Supplementary Fig. 1). Furthermore, the UPGMA cluster analysis confirmed the close genetic relationship among C. nutans samples, allowing for their classification into 2 distinct divisions, each with a similarity coefficient of 0.95 (Fig. 1B). The first division was composed of Chiang Mai, Chiang Rai, Phayao, Khon Kean, Nakhon Ratchasima, and Nonthaburi provinces, while the other group included Lamphun, Kalasin, and Loei provinces. This technique effectively distinguished the C. nutans from the outsource group, yielding a UPGMA coefficient of 0.44.
Genetic diversity of C. nutans. (A) SRAP technique using 7 pairs of primers (Lane 1–10: C. nutans from Chiang Mai, Chiang Rai, Phayoa1, Phayoa2, Lamphun, Khon Kaen, Nakhon Ratchasima, Kalasin, Loie and Nonthaburi; Lane 11: P. pulcherrimus; Lane 12: R. tuberosa) (B) Dendrogram of UPGMA cluster analysis.
Anti-cell death activity of C. nutans extract in LPS-induced endothelial cells
To compare the biological activity of C. nutans samples, the ethanol extracts were tested for their protective activity against LPS-induced cell death in bovine endothelial cells. Our previous study reported a novel biological function of C. nutans in lowering the effect of LPS in the bovine mastitis model12. Sublethal doses, as determined in cytotoxicity tests, were used in the experiments with bovine endothelial CPAE cells (Supplementary Fig. 2). In accompanied to our earlier findings, C. nutans extracts from 10 different regions exhibited significant potential to neutralize the effects of LPS (Fig. 2). LPS treatment at the concentration of 20 ng/mL dramatically caused cell death, reducing cell viability to less than 25% after 24 h of exposure (Fig. 2A). However, treatment with C. nutans extract was able to inhibit the LPS-induced cell death, increasing cell viability to more than 80% at the highest tested concentration (500 μg/mL), except for the extract from Nakhon Ratchasima (Fig. 2A,B). When comparing the effectiveness among C. nutans extracts, those from the Northern region of Thailand, including Chiang Mai and Phayao, demonstrated the greatest potential to inhibit more than 50% cell viability at a concentration as low as 31.25 μg/mL.
Anti-cell death activity of C. nutans extracts. The ethanol extracts of C. nutans were determined for their ability to prevent the cell death caused by LPS. CPAE bovine endothelial cells were treated with 20 ng/mL of LPS in absence or presence of C. nutans extracts (7.81–500 μg/mL). (A) The percentage of cell viability was monitored after 24 h of treatment relative to non-treatment control (set as 100%) (*p < 0.05; **p < 0.01, ***p < 0.001). (B) The cell morphology was observed after 24 h of treatment.
Antioxidant activity of C. nutans extract
The antioxidant activity of C. nutans extract is well-recognized. To compare the antioxidant properties of these extracts, the DPPH assay was performed and calculated for the half-maximal inhibitory concentration (IC50) using non-linear regression analysis. The result confirmed the presence of free radical scavenging capacity in all extracts, although the activity levels varied across different sources (Table 2). The IC50 values ranged from 2.559 ± 0.378 (Chiang Mai) to 9.219 ± 0.210 (Kalasin). Notably, the extract from Chiang Mai exhibited the highest activity; with no statistically significant differences observed when compared to extracts from Chiang Rai (IC50 value of 2.799 ± 0.454), Loei (IC50 value of 3.019 ± 0.092), and Nonthaburi (IC50 value of 3.059 ± 0.270).
Antibacterial activity of C. nutans extract
Previous research has already reported the anti-bacterial activity of C. nutans in inhibiting the growth of certain bacteria and yeast including Bacillus cereus, Escherichia coli, Salmonella enterica serovar Typhimurium, and Candida albicans13. In our study, we aimed to compare the activities of C. nutans extracts in inhibiting the growth of Staphylococcus epidermidis, Staphylococcus aureus, Pseudomonas aeruginosa, and Escherichia coli, which are common opportunistic pathogens in humans. Among 10 extracts, those from Chiang Mai province conferred the most potent activity against S. epidermidis, S. aureus, P. aeruginosa, and E. coli with MIC values of 31.25, 62.5, 62.5, and 31.25 mg/mL, respectively. Furthermore, MBC values for these extracts were determined to be 250, 500, 250, and 500 mg/mL, respectively (Table 3).
Phytochemical profiles of C. nutans
While the genetic profiles revealed a close genetic relationship among 10 C. nutans samples, the biological activities of these samples varied across different function assays. To elucidate the connection between biological compounds and biological activities, we conducted an investigation of the phytochemical profiles of C. nutans. The extracts were determined for their total phenolic contents using the Folin-Ciocalteu assay. The result showed the extracts from the Northern region had, on average, higher total phenolic content compared to those from Northeastern, and Central regions of Thailand (Fig. 3A). The highest total phenolic content was found in the extract from Chiang Mai province (7.30 μg GAE/g extract), whereas the lowest content was observed in the extract from Nonthaburi (2.96 μg GAE/g extract). Furthermore, the profile was characterized using HPLC (Fig. 3B). The HPLC chromatogram exhibited a consistent pattern, but differences were observed in the area under the curves, particularly in the case of four major peaks (indicated by retention times). When we determined the gallic acid and quercetin which were the common active ingredients in plants, interestingly, we observed their presence in relatively low amounts under our tested conditions and mobile phase (Fig. 3A,B) suggesting that the gallic acid and quercetin may not be the major phenolic compounds in C. nutans extracts.
Phytochemical analysis. (A) The ethanol extracts of C. nutans were determined for their phenolic contents using Folin-Ciocalteu assay. (B) Phytochemical profile of the extracts was evaluated using HPLC with a mobile phase consisting of H2O containing 0.5% glacial acetic acid (solvent A) and methanol (solvent B). The pure gallic acid and quercetin were used as references to identify and calculate the gallic acid and quercetin contents in the extracts.
Discussion
C. nutans is widely distributed in tropical Asia, where it has been originally found5. Although C. nutans has diverse biological activity (i.e., antioxidant, anti-inflammation, anti-diabetes, anti-bacterial, anti-viral activities) as well as its long history of safe traditional use, unfortunately, there is a limited availability of commercial C. nutans products in the market. These products are primarily utilized for treating the clinical symptoms of herpes simplex virus and varicella-zoster virus infection14. Currently, although the biological activities of C. nutans have been extensively studied, none of these studies have explored the relationship between its genetic background, phytochemical profile, and biological activity5. In this present study, we aim to provide insight into the genetic background, phytochemical profile, and biological activity of C. nutans obtained from 10 different regions in Thailand.
We conducted an investigation into the genetic polymorphism of C. nutans using DNA fingerprinting, a highly effective and straightforward technique for studying genetic variations15. The genotype of a living organism naturally influences its phenotypic traits. However, even slight genetic differentiation and/or variation can have significant implications for quantitative traits within populations. Understanding the naturally occurring genetic variation and its impact on the bioactive compound compositions and/or biological activities is vital for the breeding programs aimed at enhancing productivity, quality, and the sustainable conservation of C. nutans. In our study, C. nutans samples collected from 10 regions in Thailand were characterized using SRAP technique which was used to amplify coding regions of DNA. SRAP technique is the potential tool applied in various aspects of plant biology, including agronomic and horticultural purposes, analysis of linkage map construction, and identification of quantitative trait loci for advanced hybrids11,16. In comparison to the other dominant markers like inter-simple sequence repeat (ISSR), random amplified polymorphic DNA (RAPD), and amplified fragment length polymorphism (AFLP), SRAP markers have the advantage of efficiently elucidating genetic variation at different taxonomic levels with lower cost, reduced technique complexity, and high reproducibility11,17. Our results demonstrated seven pairs of SRAP markers effectively distinguished C. nutans from outgroup species, including Phlogacanthus pulcherrimus and Ruellia tuberosa which belong to the same Acanthaceae family. This indicates the potential applicability of SRAP in identifying of C. nutans from the other species, especially in case where misidentification occurs due to closely related morphological and test characteristics or when identification becomes challenging after the drying process. However, the genetic fingerprint of the 10 C. nutans samples revealed homogeneity with a similarity coefficient of 0.95 (Fig. 1B). This aligns with previous findings, as C. nutans has been reported to exhibit high genetic homogeneity18. In agreement with our results, Fong and colleagues reported low genetic variation in C. nutans among 12 samples collected from Malaysia, Vietnam, and Thailand, using techniques such as restriction fragment length polymorphism (RFLP), RAPD, and microsatellite marker18. The primary reason for the low variation of C. nutans is its common propagation method among local communities, involving stem cutting (vegetative propagation), which results in genetically identical plant replicas.
Based on our findings using the SRAP technique, low genetic variation was observed among C. nutans samples from different locations. This suggests that genetic variation is unlikely to have a substantial impact on the biological activity of C. nutans. However, it is important to note that we cannot conclude that genetic diversity has no effect on biological activity, as there are limitations associated with the technique we used, including its sensitivity and the sample size. The SRAP marker is a dominant marker; thus, SRAP amplicons cannot yield heterozygosity descriptors that could directly influence the patterns of the diversity11. Recent research has utilized the random amplified microsatellite polymorphism (RAMP) technique, which is a co-dominant molecular marker, to evaluate the genetic diversity of 80 C. nutans accessions in Malaysia at the population levels15. Therefore, the combined use of SRAP with other markers such as RAPD, AFLP, RAMP, etc. could offer benefits in elucidating greater levels of variation, especially within a group of highly related populations.
The extensive biological activity spectrum of C. nutans has been well-documented, indicating the potential in diverse areas, encompassing both infectious (anti-bacterial and anti-virus activity) and noninfectious diseases (anti-inflammation, antioxidant, and anti-diabetes). In our study, we determined the anti-cell death, antioxidant, and anti-bacterial activities and compared the efficacy of C. nutans extracts derived from 10 different regions. We demonstrated the anti-bacterial activity of C. nutans against common pathogenic bacteria, including S. epidermidis, S. aureus, P. aeruginosa, and E. coli (Table 3), thereby confirming the efficacy of the extract as an anti-bacterial agent. Recent research has highlighted the protective effect of C. nutans extract in a hepatitis B virus (HBV) mouse model19. Administration of C. nutans in HBV mouse model not only lowered the expression level of HBV antigen but caused the alteration of gut microbiota evidenced by a significant decrease in the proportion of Lactobacillaceae and Lactobacillus, accompanied by a substantial increase in the relative abundance of Bacteroidales_S24-7 group, Rikenellaceae, and Alistipes. It is important to note that while the impact of C. nutans extract on the alteration of these bacterial strains was observed, there is currently no direct evidence demonstrating the direct effect of the extract on the growth of these bacteria. It is plausible that the observed result may be attributed to cumulative effects within the gut microbiota community. However, these findings along with metabolomic results (demonstrating the upregulation of hippuric acid, L-histidine, trehalose, D-threitol, and stachyose, and downregulation of uridine 5′-diphosphate, cholic acid, trimethylamine N-oxide, CDP-ethanolamine, and phosphorylcholine) underscore the effectiveness of C. nutans in reducing HBV clinical symptoms and conferring protective effects in HBV model mice. This suggests the potential application of C. nutans in controlling bacterial growth, which may extend to various diseases, not only bacterial infection but also those related to gut microbiota disorders such as anti-HBV infection.
In addition to its anti-bacterial activity, C. nutans treatment exhibited a protective effect against LPS-induced cell death in bovine endothelial cells (Fig. 2) and demonstrated antioxidant activity, as accessed by DPPH assay (Table 2). Oxidative stress, characterized by the excessive production of reactive oxygen or nitrogen species (ROS/RNS), has been extensively documented to play a crucial role in cell death. Elevated ROS/RNS levels in cells lead to lipid membrane disruption, increased fluidity and permeability, protein dysfunction, and aggregation, as well as DNA damage, ultimately resulting in cell damage and apoptosis. This oxidative stress is implicated in the pathogenesis of various diseases, including metabolic diseases, cancer, and other chronic diseases20. Considering the common mechanism, it is reasonable to infer that the anti-oxidant activity plays a role in the anti-cell death properties of C. nutans extract. On the other hands, the anti-bacterial and anti-oxidant activities has been reported to correlate with the polyphenolic levels of the extract21. In our experiments, when comparing the biological effectiveness of 10 C. nutans extracts, the extract from the Northern part of Thailand demonstrated the highest biological potency compared to the extracts from other regions. Specifically, extracts from Chiang Rai (DPPH IC50 = 2.799 ± 0.454) exhibited the greatest efficacy in protecting the bovine endothelial cells from LPS-induced cell death (Fig. 2). Additionally, extracts from Chiang Mai (DPPH IC50 = 2.559 ± 0.378) had the highest levels of anti-bacterial activities, as determined by MIC/MBC technique (Table 3).
The evaluation of phytochemical compounds using the Folin-Ciocalteu assay to determine the phenolic content revealed that extracts from the Northern regions contained higher phenolic content than those from other regions. Notably, the extract from Chiang Mai exhibited the highest content, measuring 7.30 μg GAE/g extract (Fig. 3A) suggesting that the biological activity of C. nutans extract is associated with the bioactive contents present in each extract. Furthermore, we investigated the phytochemical profiles of these extracts, comparing them across 10 regions using HPLC. Our results indicated that all extracts shared a common profile of chromatogram but varied in terms of quantity based on the peak area. We used gallic acid and quercetin, which have been reported as common phenolic compounds of C. nutans, as the references22,23. However, our result revealed that gallic acid and quercetin were not the major compounds in C. nutans extracts and were not detectable in some extracts, at least under the conditions we tested (Fig. 3).
Total phenolic and flavonoid contents were positively associated with the biological activity of C. nutans extract, especially the terms of antioxidant activity22. The amount of gallic acid and quercetin can vary depending on the metabolite content itself, as well as the extract method and extraction solvent used. For instance, extractions of C. nutans with hot aqueous, aqueous, and aqueous methanol contained sub-detectable levels of gallic acid although these extracts performed the highest DPPH radical scavenging activity when compared to other extract solvents such as hexane, ethyl acetate, absolute methanol24. On the other hand, other phenolic compounds were confirmed in these extracts, including protocatechuic acid, chlorogenic acid, ferulic acid, and caffeic acid24, which may contribute to the antioxidant activity. In our study, gallic acid and quercetin were found as minor compounds in our extracts, they may not be suitable as biomarkers for pharmacological selection. However, it is important to note that the contribution of these compounds to the activity of C. nutans cannot be entirely ruled out, as they may synergistically with the other phenolic and flavonoid compounds in the extract. Alternatively, the basic measuring of total phenolic/flavonoid content serves as a reasonable indicator, at least for assessing antioxidant properties.
Ghasemzadeh and colleagues previously reported on the relationship between the pharmacological activity of C. nutans and the age of the plant22. Their study compared plants with ages ranging from 1 month old to 1 year old and observed that plant age influenced the variation in photochemical synthesis. Specifically, the age of plant appeared to affect the phytochemical contents of buds, with 6-month-old C. nutans buds showing the highest amount of total phenolic content (18.21 ± 1.12 mg/g dry weight) and total flavonoid content (6.32 ± 0.74 mg/g dry weight). However, the age of the plant had a relatively lesser effect on the leaves, whether they were 1 month, 6 months, or 1 year old, with the total phenolic content ranging from 7.29 ± 0.80 to 11.32 ± 1.2 mg/g dry weight, and total flavonoid content ranging from 3.79 ± 0.29 to 4.66 ± 0.43 mg/g dry weight22. Notably, both buds and leaves exhibited a similar trend, with phytochemical content decreasing after 6 months of age. In our study, we did not compare the age of samples, we thus cannot conclude that the variations observed in our study were due to differences in plant age. Further investigations, especially considering factors such as cultivation conditions (e.g., growth period, minerals, stress), and their associations with phytochemical content and biological activity, are necessary to establish the best practices for C. nutans cultivation.
Conclusion
C. nutans collected from different regions of Thailand exhibited low genetic variation. The observed differences in their biological activities, which included anti-apoptotic, antioxidant, and anti-bacterial activities, were mainly associated with the level of total phenolic and flavonoid contents within the extracts which were possibly influenced by environmental factors.
Methods
Collection of plant materials and extraction
C. nutans leaves were collected from different regions of Thailand during 2019–2020. The collection of plant material complied with relevant institutional, national, and international guidelines and legislation. The fresh leaves of cultured plants were collected from Mae Rim District (Chiang Mai Province), Chiang Khong District (Chiang Rai Province), Chiang Kham District (Phayao Province; Phayao Field 1), Li District (Lamphun Province), Sikhio District (Nakhon Ratchasima Province). The others were bought at the local markets from Muang District (Phayao Province; Phayao Field 2), Muang District (Khon Kaen Province), Na Haeo District (Loei Province), Muang District (Kalasin Province), Bamg Yai District (Nonthaburi Province). The plant specimens were identified by botanist Dr. Wittaya Pongamornkul and deposited at the Queen Sirikit Botanic Garden Herbarium (QSBG herbarium) as following voucher numbers; WP8603 (Chiang Mai), WP8604 (Chiang Rai), WP8605 (Phayao Field 1), WP8606 (Phayao Field 2), WP8607 (Lamphun), WP8608 (Khon Kaen), WP8609 (Nakhon Ratchasima), WP8610 (Kalasin), WP8611 (Loei), and WP8612 (Nonthaburi).
The fresh leaves were oven-died at 50 ºC and subsequently ground into a fine powder using a conventional grinder. The extraction procedures followed the established protocol outlined in our previous studies12,25. Briefly, the extraction was performed by using 70% ethanol at the ratio of 1:20, with shaking at 160 rpm/minute (room temperature) for 12 h. The extract was filtrated using Whatman No. 1 filter paper and subjected to evaporation in a rotary evaporator. The weight after evaporation was measured to calculate the % yield of the extracts (Table 1). The extracts were stored at 4 ºC until used.
Sequence-related amplified polymorphism (SRAP) and UPGMA cluster analysis
SRAP technique was performed as described previously16. Briefly, DNA was isolated using the NucleoSpin Plant II (MACCHEREY-NAGEL, Germany. Ten nanograms of DNA were used as the template of PCR using High-Purity i-Taq™ PCR core kit (iNtRON Biotechnology, Inc., Korea) and 7 different pairs of primers including M2/E7, M2/E8, M3/E6, M3/E7, M4/E2, M4/E3, and M4/E5 (Supplementary Table 1). The PCR reaction was conducted with steps of initial melting (95 °C 5 min) followed by 5 rounds of denaturation (94 °C 1 min), annealing (35 °C 1 min), and extension (72 °C 2 min). After then, the reaction was continued by 35 rounds of denaturation (94 °C 30 s), annealing (56 °C 30 s), extension (72 °C 2 min) followed by a final extension at 72 °C for 5 min. The PCR product was analyzed by gel electrophoresis using 1.5% agarose gel. The DNA band was visualized using by ImageQuant LAS 500 Chemiluminescent Imaging System (GE, Boston, MA, USA).
Cell line and cell culture
Bovine endothelial cell line: CPAE, (CCL209TM, ATCC, VA, USA) was cultured in the minimal essential medium (MEM) with 20% (v/v) fetal bovine serum (FBS) supplementation. The cells were incubated at 37 ºC under a 5% CO2 humidified atmosphere and subcultured when the cell growth reached 80% confluence.
LPS treatment and cell viability assay
The effect of LPS on CPAE cell death and the anti-cell death activity of C. nutans extracts were determined by using a cell viability assay as previously described12. The term “anti-cell death activity” refers to the ability of the extract to prevent cell death triggered by LPS stimulation. In our study, we employed endothelial cells (CPAE), which are highly sensitive to LPS activation and play a crucial role in inflammatory response, to illustrate the capacity of extracts to inhibit LPS-induced cell death. We compared the cell viability between treatment conditions with or without extracts as a measure of their anti-cell death activity. Briefly, CPAE was plated a day before the experiment in a 96-well plate (7,000 cells/well). The LPS derived from Escherichia coli (catalog no. L4391, E. coli 0111: B4, Sigma-Aldrich, St. Louis, MO, USA) was treated to the CPAE at the concentration of 20 ng/mL in the presence or absence of C. nutans extracts at the various concentrations ranging from 7.81 to 500 μg/mL. After 24 h of treatment, the cells were harvested and measured for the cell viability by using PrestoBLUE reagent (Thermo Fisher Scientific, MA, USA) to monitor the reducing capacity of living cells relative to non-treatment control. The changes in absorbance were determined at 570 nm and 600 nm using a microplate reader (EZ Read 2000, Biochrom, Cambridge, UK) and used to calculate the percentage of cell viability (% cell viability) relative to that of non-treatment control which set as 100% following this equation.
DPPH radical scavenging assay
The 2,2-diphenyl-1-picrylhydrazyl (DPPH) radical scavenging assay was performed by the method reported by Prieto et al.26 to determine the antioxidative activity of C. nutans extracts. The extracts were prepared in methanol at various concentrations and mixed with a 0.1 mM DPPH reagent (Sigma-Aldrich, Germany). The plate was incubated for 20 min in a dark place at room temperature and measured for the absorbance at the wavelength of 517 nm and compared to the standard curve obtained from gallic acid (Sigma-Aldrich, Darmstadt, Germany). The antioxidative activity of C. nutans extracts was represented as mg of gallic acid equivalents per gram extract (mg GAE/g extracts).
Minimum inhibitory (MIC) and minimum bactericidal concentration (MBC) evaluations
Minimum Inhibitory (MIC) and minimum Bactericidal Concentration (MBC) evaluations were conducted as previously described27. The MIC values were determined by using the broth dilution method. Standard laboratory strains of bacteria including Staphylococcus epidimidis (ATCC 14990), Staphylococcus aureus (ATCC 25923), Pseudomonas aeruginosa (ATCC 27853) and Escherichia coli (ATCC 25922) were kindly obtained from the Microbiology Section, Department of Medical Technology, Faculty of Associated Medical Science, Chiang Mai University, Chiang Mai, Thailand. The bacterial suspension was prepared in McFarland No. 0.5. The C. nutans extract (7.8–500 mg/mL) was then added to the bacterial culture. The turbidity reflecting bacterial cell growth was measured after 24 h of incubation at 37 °C. The MBC values was determined by streaking the plate from the tubes in which the bacterial was not visible. The plate was incubated for 24 h at 37 °C to evaluate the MBC endpoint which defined as the lowest concentration that C. nutans inhibited up to 99.9% of bacterial growth.
Folin-Ciocalteu assay
The Folin-Ciocalteu assay was conducted as described by Wolfe et al.28 to determine the total phenolic contents in C. nutans extracts. Briefly, the extracts were mixed with 50% (w/v) Folin-Ciocalteu reagent (Merck, USA) and incubated for 5 min in a dark place before adding 5% (w/v) sodium carbonate. The reaction was incubated for 1 h and measured for the absorbance at OD725. The gallic acid (Sigma-Aldrich, Darmstadt, Germany) was used as the reference in which the total phenolic contents were calculated and represented as mg of gallic acid equivalents per gram extract (mg GAE/g extracts).
High-performance liquid chromatography (HPLC)
The phytochemical profiles of C. nutans extracts were determined by using gradient HPLC systems with the ZORBAX Eclipse XDB-C18 column (Agilent Technologies, USA) as the protocol described previously29. HPLC used a mobile phase consisting of water with 0.5% glacial acetic acid (solvent A) and methanol (solvent B). The gradient steps were conducted at ambient temperature using the following steps: 100% A, 0–20 min; 50% A, 20–30 min; 40% A, 432 30–35 min; 30% A, 35–40 min; 20% A, 40 min; post-time, 5 min before next injection. The flow rate was 1.0 mL/minute, and the injection volume was 20 μL. A UV photodiode array detector (270 nm) was used to monitor the wavelength. The gallic acid and quercetins (Sigma-Aldrich, Darmstadt, Germany) were used as the standard compound to calculate the amount of gallic acid and quercetins in C. nutans extracts.
Statistical analysis
The data from at least three independent experiments were used to analyze the statistical differences using the Student’s t-test (GraphPad Prism version 5.0).
Data availability
The data supporting this study's findings are available from the corresponding author upon reasonable request.
Abbreviations
- SRAP:
-
Sequence-related amplified polymorphism
- LPS:
-
Lipopolysaccharide
- MIC:
-
Minimum inhibitory concentration
- MBC:
-
Minimum bactericidal concentration
- DPPH:
-
2,2-Diphenyl-1-picrylhydrazyl
- HPLC:
-
High-performance liquid chromatography
References
Yuan, H., Ma, Q., Ye, L. & Piao, G. The traditional medicine and modern medicine from natural products. Molecules 21, 559 (2016).
Dias, D. A., Urban, S. & Roessner, U. A historical overview of natural products in drug discovery. Metabolites 2, 303–336 (2012).
Shinwari, Z. K. et al. Ethnobotany and medicinal uses of folklore medicinal plants belonging to family acanthaceae: An updated review. MOJ Biol. Med. 1, 34–38 (2017).
Aung, M. H. et al. Documentation of plant diversity of Southeast Asia: The new role of Belt and Road Initiative. PhytoKeys 138, 1–2 (2020).
Alam, A. et al. Clinacanthus nutans: A review of the medicinal uses, pharmacology and phytochemistry. Asian Pac. J. Trop. Med. 9, 402–409 (2016).
Arullappan, S., Rajamanickam, P., Thevar, N. & Kodimani, C. In vitro screening of cytotoxic, antimicrobial and antioxidant activities of Clinacanthus nutans (Acanthaceae) leaf extracts. Trop. J. Pharm. Res. 13, 1455–1461 (2014).
Kunsorn, P. et al. The identities and anti-herpes simplex virus activity of Clinacanthus nutans and Clinacanthus siamensis. Asian Pac. J. Trop. Biomed. 3, 284–290 (2013).
Vachirayonstien, T. et al. Molecular evaluation of extracellular activity of medicinal herb Clinacanthus nutans against herpes simplex virus type-2. Nat. Prod. Res. 24, 236–245 (2010).
Sangkitporn, S. et al. Treatment of herpes zoster with Clinacanthus nutans (bi phaya yaw) extract. J. Med. Assoc. Thai. 78, 624–627 (1995).
Sittiso, S. et al. Effects of compounds from Clinacanthus nutans on dengue virus type 2 infection. Srinagarind Med. J. 25, 272–275 (2010).
Robarts, D. W. & Wolfe, A. D. Sequence-related amplified polymorphism (SRAP) markers: A potential resource for studies in plant molecular biology (1). Appl. Plant. Sci. 2, 1400017 (2014).
Panya, A. et al. Antibiotic-antiapoptotic dual function of Clinacanthus nutans (Burm. F.) Lindau leaf extracts against bovine mastitis. Antibiotics 9, 429 (2020).
Arullappan, S., Rajamanickam, P., Thevar, N. & Kodimani, C. C. In vitro screening of cytotoxic, antimicrobial and antioxidant activities of Clinacanthus nutans (Acanthaceae) leaf extracts. Trop. J. Pharm. Res. 13, 1455–1461 (2014).
Zulkipli, I. N., Rajabalaya, R., Idris, A., Sulaiman, N. A. & David, S. R. Clinacanthus nutans: A review on ethnomedicinal uses, chemical constituents and pharmacological properties. Pharm. Biol. 55, 1093–1113 (2017).
Ismail, N. Z., Arsad, H., Samian, M. R., Ab Majid, A. H. & Hamdan, M. R. Evaluation of genetic diversity of Clinacanthus nutans (Acanthaceaea) using RAPD, ISSR and RAMP markers. Physiol. Mol. Biol. Plants 22, 523–534 (2016).
Li, G. & Quiros, C. F. Sequence-related amplified polymorphism (SRAP), a new marker system based on a simple PCR reaction: Its application to mapping and gene tagging in Brassica. Theor. Appl. Genet. 103, 455–461 (2001).
Amiteye, S. Basic concepts and methodologies of DNA marker systems in plant molecular breeding. Heliyon 7, e08093 (2021).
Fong, S. Y., Piva, T., Utban, S. & Huynh, T. Genetic homogeneity of vegetatively propagated Clinacanthus nutans (Acanthaceae). J. Med. Plant Res. 8, 903–914 (2014).
Zhang, R. et al. Protective effects of Clinacanthus nutans (Burm.f.) Lindau aqueous extract on HBV mouse model by modulating gut microbiota and liver metabolomics. Evid. Based Complement. Alternat. Med. 2023, 5625222 (2023).
Sharifi-Rad, M. et al. Lifestyle, oxidative stress, and antioxidants: Back and forth in the pathophysiology of chronic diseases. Front. Physiol. 11, 694 (2020).
Stagos, D. et al. Correlation of total polyphenolic content with antioxidant and antibacterial activity of 24 extracts from Greek domestic Lamiaceae species. Food Chem. Toxicol. 50, 4115–4124 (2012).
Ghasemzadeh, A., Nasiri, A., Jaafar, H. Z., Baghdadi, A. & Ahmad, I. Changes in phytochemical synthesis, chalcone synthase activity and pharmaceutical qualities of sabah snake grass (Clinacanthus nutans L.) in relation to plant age. Molecules 19, 17632–17648 (2014).
Nisar, A. B. & Khan, W. A. Identification of phenolic compound from the leaves extract of Clinacanthus nutans Lindau. Plant Cell Biotechnol. Mol. Biol. 21, 115–120 (2020).
Sarega, N. et al. Phenolic rich extract from Clinacanthus nutans attenuates hyperlipidemia-associated oxidative stress in rats. Oxid. Med. Cell Longev. 2016, 4137908 (2016).
Thongyim, S. et al. Anti-inflammatory activity of glyceryl 1,3-distearate identified from Clinacanthus nutans extract against bovine mastitis pathogens. Antibiotics 12, 549 (2023).
Prieto, P., Pineda, M. & Aguilar, M. Spectrophotometric quantitation of antioxidant capacity through the formation of a phosphomolybdenum complex: Specific application to the determination of vitamin E. Anal. Biochem. 269, 337–341 (1999).
CLSI. M100 Performance Standards for Antimicrobial Susceptibility Testing (Clinical and Laboratory Standards Institute, 2019).
Wolfe, K., Wu, X. & Liu, R. H. Antioxidant activity of apple peels. J. Agric. Food Chem. 51, 609–614 (2003).
Panya, A. et al. Triphala in traditional ayurvedic medicine inhibits dengue virus infection in Huh7 hepatoma cells. Pharmaceuticals 14, 1236 (2021).
Acknowledgements
We would like to acknowledge the Department of Biology, Faculty of Science for facilities and special equipment. Saruda Thongyim was supported by Office of Research Administration, Chiang Mai University.
Funding
This research project was supported by Fundamental Fund 2023, Chiang Mai University [Grant no. FF66/038].
Author information
Authors and Affiliations
Contributions
S.C. conducted the experiments and data analysis. A.I., S.W., and W.P. collected plant samples, identification, and voucher number deposition. S.T., H.P., T.K. performed the herb extraction and data analysis. Y.T. and S.J. designed the experiment and edited a manuscript. A.P. conducted the experiment, and drafted and revised a manuscript.
Corresponding author
Ethics declarations
Competing interests
The authors declare no competing interests.
Additional information
Publisher's note
Springer Nature remains neutral with regard to jurisdictional claims in published maps and institutional affiliations.
Supplementary Information
Rights and permissions
Open Access This article is licensed under a Creative Commons Attribution 4.0 International License, which permits use, sharing, adaptation, distribution and reproduction in any medium or format, as long as you give appropriate credit to the original author(s) and the source, provide a link to the Creative Commons licence, and indicate if changes were made. The images or other third party material in this article are included in the article's Creative Commons licence, unless indicated otherwise in a credit line to the material. If material is not included in the article's Creative Commons licence and your intended use is not permitted by statutory regulation or exceeds the permitted use, you will need to obtain permission directly from the copyright holder. To view a copy of this licence, visit http://creativecommons.org/licenses/by/4.0/.
About this article
Cite this article
Chiangchin, S., Thongyim, S., Pandith, H. et al. Clinacanthus nutans genetic diversity and its association with anti-apoptotic, antioxidant, and anti-bacterial activities. Sci Rep 13, 19566 (2023). https://doi.org/10.1038/s41598-023-46105-z
Received:
Accepted:
Published:
DOI: https://doi.org/10.1038/s41598-023-46105-z
- Springer Nature Limited