Abstract
To explore the function of fibulin-3 in cervical carcinoma malignant cell growth and metastasis, fibulin-3 expression in normal cervical tissue, cervical intraepithelial neoplasia (CIN), and cervical carcinoma were evaluated by immunohistochemistry. Quantitative real-time-polymerase chain reaction, western blotting, and immunocytochemistry were performed to assess the expression of fibulin-3 at mRNA and protein levels in different invasive clone sublines. Fibulin-3 shRNA and fibulin-3 cDNA were used to transfect the strongly and weakly invasive clone sublines. Using in vitro and in vivo functional assays, we investigated the effects of down-regulating and up-regulating fibulin-3 expression on the proliferation and invasion of different clone sublines. Epithelial mesenchymal transition (EMT) and its signaling pathways PI3K/AKT and ERK were studied carefully in lentiviral transfection systems. Fibulin-3 was upregulated in cervical carcinoma, and its overexpression was significantly related with malignant phenotype and poor prognosis of cervical carcinoma. Fibulin-3 promoted cervical cancer cell invasive capabilities by eliciting EMT and activating the PI3K-Akt-mTOR signal transduction pathway. Fibulin-3 could facilitate the process of cervical cancer development. The results presented here will help develop novel prognostic factors and possible therapeutic options for patients with cervical cancer.
Similar content being viewed by others
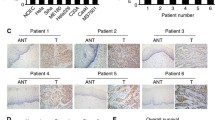
Introduction
Cervical cancer is the fourth most common malignancy in women worldwide, with an estimated global incidence of more than 500,000 new cases and approximately 233,000 deaths per year1. The incidence and mortality of cervical cancer is higher in developing countries than in developed countries2. Presently, although the combined treatments, including surgery, radiotherapy, and chemotherapy, have been attempted to treat cervical cancer, recurrence and metastasis in patients at an advanced stage still result in 5-year survival rates lower than 50%3. Therefore, studying the underlying functional molecular and regulation mechanisms of tumor invasion and metastasis will provide further insight into the tumorigenesis and development of cervical cancer.
Human fibulin-3 (FBLN-3), encoded by the epidermal growth factor-containing fibulin-like extracellular matrix protein-1 (EFEMP-1) gene on chromosome 2p16, contains 11 exons, and encodes a 493-amino acid protein with a molecular mass of 54 kDa4,5. Fibulin-3 is a member of the secreted extracellular glycoprotein family and it is widely expressed in basal membranes, stroma, and extracellular matrix fibers. Seven extracellular matrix proteins form the fibulin family and mediate cell-to-cell and cell-to-matrix conduction6. Recent studies have yielded conflicting results about the function of fibulin-3 in tumor biology, showing that affinity or anti-tumor biological activity upon up- or downregulated expression depends on the type of cancer. In ovarian cancer7,8, osteosarcoma9, pancreatic cancer10, and glioblastoma11, fibulin-3 up-regulation was associated with poor prognosis because of malignant cell growth and invasion. On the other hand, downregulation of fibulin-3 in liver cancer12,13, gastric carcinoma14, lung carcinoma15,16, endometrial cancer17, and nasopharyngeal cancer18, was able to suppress cancer cell growth and metastasis. In our present research, our purpose was to investigate the prognostic effect of fibulin-3 in cervical cancer, and to determine the role of fibulin-3 in cervical cancer cell proliferation and metastasis in vitro and in vivo.
Materials and Methods
Cell culture
Using the limited dilution method, strongly invasive clone sublines and the weakly invasive clone sublines were derived from the human cervical cancer cell lines, HeLa and SiHa (Shanghai Institute for Biological Sciences, Chinese Academy of Sciences). The clone sublines had different invasive potential and were suitable for analyzing the differences in gene expression, as described in a previous report19. Cells were cultured in Dulbecco’s modified Eagle medium (DMEM, Gibco BRL, Rockville, MD) with 10% fetal bovine serum (FBS, Gibco BRL, Rockville, MD).
Tissue specimens
Following informed consent from patients, a total of 285 human cervical tissue specimens were collected at the Pathology Department, Qilu Hospital and Provincial Hospital of Shandong, between 2006 and 2016. All patients received standard follow-up, with the exception of 16 patients who lost contact and 35 patients who died. This study was approved by the Medical Ethics Committee of Shandong University. All methods were performed in accordance with all relevant guidelines and regulations.
Immunohistochemistry and immunocytochemistry
Immunohistochemistry (IHC) and immunocytochemistry (ICC) were performed according to standard streptavidin-biotin-peroxidase complex procedures. Paraffin-embedded sections (5 μm thick) and cell coverslips fixed in 4% paraformaldehyde were used in these experiments. For paraffin-embedded sections, dewaxing, rehydration, and heat-induced retrieval were performed. Then, the tissue sections and the cell coverslips were incubated with mouse anti-human fibulin-3 monoclonal antibody (sc-33722, Santa Cruz Biotechnology, Inc.) at a 1:100 dilution overnight at 4 °C. Ovarian cancer tissue sections (fibulin-3-positive) were applied as a positive control7, and phosphate-buffered saline (PBS) was used to replace the original antibody as negative control. Brown granules in the cytoplasm or stroma were regarded as positive expression of fibulin-3. IHC and ICC experiments for every slide were duplicated in triplicate.
Analysis for IHC and ICC experiments
A semiquantitative scoring system was performed to evaluate the expression of fibulin-3 in the tissue sections and cell coverslips, and intensity of staining and distribution of positive cells were measured. The staining intensity was classified as 0–3 (0 = negative staining, 1 = low staining intensity, 2 = moderate staining intensity, and 3 = high staining intensity), whereas the percentage of staining-positive cells was scored as 0 (0% cells stained), 1 (1–25%), 2 (26–50%), 3 (51–75%), or 4 (76–100%)20. The sum of the staining intensity and percentage of staining-positive cell scores constituted the final staining score (0–7), and the sum-indexes −, +, ++, and +++ representing scores of 0, 1–3, 4–5, and 6–7, respectively. For statistical analysis, − and + were regarded as low fibulin-3 expression, while ++ and +++ were regarded as high fibulin-3 expression. Every section slide was independently scored by two pathologists. If a discrepancy occurred, a third pathologist was consulted to achieve consensus.
Lentivirus transfection
The lentivirus vector for fibulin-3 overexpression was achieved by recombining pGC-LV-GV287-GFP vector with the EFEMP-1 (NM_001039348) gene, described as pLVX-fibulin-3; while the lentivirus vector for fibulin-3 knockdown was achieved by cloning small hairpin RNAs (shRNAs) using a self-inactivating lentivirus vector containing a CMV-driven GFP reporter and a U6 promoter (GeneChem, Shanghai, China), described as fibulin-3 shRNA. The target sequence for fibulin-3 was 5′-TGTGAGACAGCAATGCAAA-3′. According to the specifications, in a 24-well plate, 60% confluent cells were transfected with the lentivirus vector at a multiplicity of infection (MOI) of 100. After overnight culture, the transfection mixture was replaced with normal complete growth medium to avoid cell toxicity. After 48 h or 72 h, the transfection efficiency was monitored using fluorescence microscopy and confirmed by qPCR and western blot analyses.
Western blotting
Target cells were lysed on ice using radioimmunoprecipitation assay (RIPA) lysis buffer with phenylmethylsulfonyl fluoride (PMSF) as a serine protease inhibitor (RIPA:PMSF = 100:1). After determining the protein concentration by the Bicinchoninic Acid (BCA) method, protein samples (40 µg) were separated using 10% sodium dodecyl sulfate polyacrylamide gel electrophoresis (SDS-PAGE), transferred to polyvinylidene difluoride (PVDF) membranes, and blocked for 1 h with Tris-buffered saline containing Tween 20 (TBST) and 5% bovine serum albumin (BSA). The membranes were then incubated on a shaking bed with primary antibodies (fibulin-3 sc-33722, E-cadherin sc-8426, N-cadherin sc-59987, Vimentin sc-6260, Santa Cruz; Snail ab167609, Slug ab106077, Twist ab50887, Zeb 2 ab138222, PI3K ab86714, p-PI3K ab182651, AKT ab8805, P-AKT ab38449, mTOR ab32028, p-mTOR ab109268, Abcam; p-ERK (Thr202/Tyr204) #4370, ERK #9102, Cell Signaling), at a 1:2,000 dilution overnight at 4 °C. The next day, the membranes were washed three times with TBST and then incubated with the corresponding horseradish peroxidase (HRP)-conjugated secondary antibody at room temperature for 1 h. Finally, positive labeling of the proteins on the membranes was visualized by enhanced chemiluminescence (ECL) using a Bio-Rad ECL kit (Solarbio).
Total RNA extraction and quantitative real time polymerase chain reaction
Total RNA was extracted from cervical cancer cells using the RNAiso Plus extraction reagent (TaKaRa), and complementary DNA (cDNA) was reverse-transcribed from 2 μg of the total RNA used as template in a reaction using a PrimeScript RT reagent Kit with gDNA Eraser (TaKaRa). The qPCR experiments were carried out using a Lightcycler 480 System. According to the specifications, the qPCR reaction mixture volume was 20 μl and included 10 μl of TB Green Premix Ex Taq II (TaKaRa), 2 μl of cDNA template, 0.8 μl of forward primer (10 μM), 0.8 μl of reverse primer (10 μM), and 6.4 μl of sterile water. The specific primer sequences designed by TaKaRa were as follows: EFEMP1-F: 5′-ACCCTTCCCACCGTATCCA-3′, EFEMP1-R: 5′-TCTGCTCTACAGTTGTGCGTCC-3′; CDH1-F: 5′-GGATTGCAAATTCCTGCCATTC-3′, CDH1-R: 5′-AACGTTGTCCCGGGTGTCA-3′; CDH2-F: 5′-CGAATGGATGAAAGACCCATCC-3′, CDH2-R: 5′-GCCACTGCCTTCATAGTCAAACACT-3′; VIM-F: 5′-AACCTGGCCGAGGACATCA-3′, VIM-R: 5′-TCAAGGTCAAGACGTGCCAGA-3′; SNAIL1-F: 5′-GCTCCCTCTTCCTCTCCATACC-3′, SNAIL1-R: 5′-AAGTCCTGTGGGGCTGATGT-3′; SNAIL2-F: 5′-GAAGCATTTCAACGCCTCCAA-3′, SNAIL2-R: 5′-GTTGTGGTATGACAGGCATGGAGTA-3′; TWIST-F: 5′-AGCAAGATTCAGACCCTCAAGCT-3′, TWIST-R: 5′-CCTGGTAGAGGAAGTCGATGTACCT-3′; ZEB2-F:5′-AAATGCACAGAGTGTGGCAAGG-3′, ZEB2-R:5′-CTGCTGATGTGCGAACTGTAGGA-3′; ACTB-F: 5′-TGGCACCCAGCACAATGAA-3′, ACTB-R: 5′-CTAAGTCATAGTCCGCCTAGAAGCA-3′. The relative gene expression levels were analyzed using the ΔΔCt method.
Cell proliferation assay
Cell proliferation capacities were determined by growth curves and anchorage-independent soft agar colony formation assays. For growth curves, cells in the logarithmic growth phase were seeded into 24-well plates and cultured for seven consecutive days. Every day, three random wells were selected, digested by trypsin, and counted by an Automated Cell Counter (Thermo Fisher Scientific, Inc.). Growth curves were generated according to the number of cells counted for each day. For the colony formation assay, 1.5 ml of 1.2% agar was mixed with 1.5 ml of 2× DMEM supplemented with 20% FBS and solidified in 3.5-cm dishes to form the base layer. For the top layer, 200 µl of cells suspended in medium (3 × 103/ml) were mixed with 1.5 ml of 0.7% agar and 1.5 ml of 2× DMEM, and quickly added to the solidified base agar layer. All the dishes were cultured in an incubator at 37 °C for 2 weeks. The experiment was repeated three times for each group. The colony formation capacities were monitored through a microscope. A cluster with a diameter more than 2 mm was defined as a colony. Then colonies were counted in five random fields in each quadrant and the average was calculated.
Boyden Chamber migration and invasion assay
Boyden Chamber migration and invasion assays were performed as described by Albini21. Polyvinylpyrrolidone-free polycarbonate (PVPF) filters with an 8.0 μm pore size divided the chambers into two segments. Matrigel (BD Biosciences) was usually used as the basement membrane matrix. For the invasion assay, the PVPF membranes were coated with 50 µl of a 1:5 dilution of Matrigel. There was no Matrigel coating in the migration assays. The remaining steps were the same for the both assays. First, 600 µl of serum-free NIH3T3-conditioned medium was filtered and added to the bottom chamber as a chemotactic factor. Second, 2 × 105 cells suspended in 200 µl medium were added into the upper chamber; finally, after 24 h of culture at 37 °C and 5% CO2, the non-migrated or non-invaded cells in the upper membrane chamber were removed, and the migrated or invaded cells in the lower chamber were fixed with 4% paraformaldehyde, stained with crystal violet, and quantitated by counting the cells from five random fields in each quadrant. Each experiment was performed in triplicate.
Tumor growth assay
Female 5-week-old BALB/C-nu/nu nude mice were purchased from the National Resource Center for Rodent Laboratory Animal of China for in vivo experiments. Each group contained five nude mice; each was injected subcutaneously with 5.0 × 106 cells and maintained in a sterile animal facility. Tumor volumes were measured by Vernier calipers at the indicated times. After 2 months, the mice were sacrificed and the tumors were dissected. This animal experiment was approved by the Institutional Animal Care and Use Committee of Shandong University and was in compliance with all regulatory guidelines.
Statistical analysis
Statistical analyses were performed using SPSS 17.0 software. Pearson chi-square or Fisher’s exact tests were used to compare differences in the proportions of fibulin-3 expression levels between groups. The quantitative data were assessed by ANOVA. A p-value < 0.05 was considered statistically significant. The correlation analyses were performed by Pearson’s product-moment correlation coefficient. The differences in survival between the groups were estimated by the Kaplan-Meier method and the log-rank test.
Results
Expression of fibulin-3 in human cervical tissue specimens
By IHC, the staining of fibulin-3 was mainly concentrated in the cytoplasm and cell membranes of cervical cancer tissues. Fibulin-3 expression was statistically lower in cervical cancer samples than that in most cervical normal and cervical intraepithelial neoplasia (CIN) tissues (Fig. 1). Fibulin-3 expression demonstrated a significant positive correlation with TNM (p < 0.01), histological grade (p < 0.01), and lymph node involvement (p < 0.01) (Table 1). Using Kaplan-Meier analysis, the prognostic value of fibulin-3 in cervical cancer was evaluated. The outcome revealed that the prognosis of patients with cervical cancer with low fibulin-3 expression was better than that in patients with high expression (Fig. 1E).
Expression of fibulin-3 in human cervical tissues. Fibulin-3 expression in (A) normal human cervical tissue, (B) cervical intraepithelial neoplasia (CIN), (C) stage I and stage II of cervical carcinoma, and (D) stage III and stage IV of cervical carcinoma were measured by IHC. (E) Cervical cancer patients with high fibulin-3 expression (blue line) had a worse prognosis than those with low fibulin-3 expression (green line). (Magnification x200).
Expression of fibulin-3 in high invasive clone sublines and low invasive clone sublines
Fibulin-3 was highly expressed in the highly invasive clone sublines, compared to the low invasive clone sublines as measured by levels of mRNA and protein determined by ICC, western blotting, and real time qRT-PCR experiments (Fig. 2). The corresponding results showed that there was a positive correlation between high fibulin-3 expression and proliferation capacity and invasion abilities of cervical cancer cells. For further confirmation, more studies were performed in the following experiments.
Fibulin-3 expression in cervical cancer cell clone sublines with different invasive ability. Fibulin-3 expression in highly invasive clone sublines and low invasive clone sublines were measured by (A) western blotting (cropped blot), (B) real time q-RT-PCR, and (C) ICC staining. (Magnification x200). *P < 0.05.
Determination of fibulin-3 transfection efficiency
Transfections using lentiviruses were performed to increase fibulin-3 expression in the low invasive clone subline HeLa-25 and to decrease fibulin-3 expression in the highly invasive clone subline HeLa-1. Then, ICC, western blotting, and qPCR analyses confirmed that HeLa-1 cells transfected with fibulin-3 shRNA had significantly lower expression levels of fibulin-3 compared the negative controls, and that fibulin-3 cDNA-transfected HeLa-25 cells had significantly higher expression levels of fibulin-3 than negative controls at both the transcription and protein levels (Fig. 3).
Identification of downregulated and upregulated fibulin-3 expression in lentivirus transfection systems. Fibulin-3 expression in control shRNA-infected cells and control cDNA-infected cells, as well as fibulin-3 shRNA-infected cells and fibulin-3 cDNA-infected cells as measured by (A) ICC staining, (B) real time q-RT-PCR, and (C) western blotting (cropped blot) (Magnification x200). *P < 0.05.
Effects of fibulin-3 on the cell proliferative capacities of cervical carcinoma
Increased expression of fibulin-3 in the low invasive clone subline HeLa-25 promoted cell proliferation capacities and clone-forming abilities, and at the same time, decreased expression of fibulin-3 in the high invasive clone subline HeLa-1 inhibited cell proliferative activities and clone-forming abilities (Fig. 4). There was a positive correlation between the expression of fibulin-3 and the proliferative capacities of cervical cancer cells.
Effects of fibulin-3 knockdown and overexpression on the proliferation and colony formation abilities of cervical cancer cells. (A) Downregulated fibulin-3 markedly inhibited cell proliferative abilities of the highly invasive clone subline cells, whereas upregulated fibulin-3 significantly promoted cell proliferative abilities of the low invasive clone subline cells. (B) The colony-forming abilities of the highly invasive clone subline cells were inhibited by fibulin-3 knockdown; at the same time, fibulin-3 upregulation promoted the colony forming abilities of the low invasive clone subline cells. (C) The colony images of control shRNA-infected cells and fibulin-3 shRNA-infected cells from a soft agar colony formation assay. (D) The colony images of control cDNA-infected cells and fibulin-3 cDNA-infected cells were examined from a soft agar colony formation assay (Magnification x200). *P < 0.05.
Effects of fibulin-3 on the cell migrating and invasive activities of cervical carcinoma
HeLa-25 cells transfected with fibulin-3 cDNA exhibited significantly enhanced migrating and invasive activities, with more migrating and invading cells through PVPF or Matrigel compared with those of control shRNA-infected cells. At the same time, HeLa-1 cells transfected with fibulin-3 shRNA exhibited markedly decreased migrating and invasive capacities, with fewer migrating and invading cells through PVPF or Matrigel compared to those of control cDNA-infected cells (Fig. 5).
Effects of fibulin-3 knockdown and overexpression on cervical cancer cell migration and invasion abilities. (A) Cell migration images of control shRNA-infected cells and fibulin-3 shRNA-infected cells, as well as control cDNA-infected cells and fibulin-3 cDNA-infected cells were measured by cell migration assays using Boyden chambers without Matrigel. (B) Cell invasion images of control shRNA-infected cells and fibulin-3 shRNA-infected cells, as well as control cDNA-infected cells and fibulin-3 cDNA-infected cells were measured by cell invasion assays using Boyden chambers coated with Matrigel. (C) The average counts of migrating and invading fibulin-3 shRNA infected cells were much lower than those of controls; meanwhile, the average counts of migrating and invading fibulin-3 cDNA infected cells were much higher than those of controls (Magnification x200). *P < 0.05.
Effects of fibulin-3 on tumor growth in vivo assay
To validate the in vitro results, in vivo tumor growth assays were performed in cells with fibulin-3 knockdown or overexpression. As shown in Fig. 6, fibulin-3 knockdown inhibited tumor formation in vivo, while fibulin-3 overexpression promoted tumor growth in vivo.
Effects of fibulin-3 knockdown and overexpression on tumor growth in vivo. (A) Knockdown of fibulin-3 inhibited tumor growth, while up-regulation of fibulin-3 promoted tumor growth. Images of xenografts in nude mice 2 months after subcutaneous inoculation of (B) control shRNA-infected cells and fibulin-3 shRNA-infected cells and (C) control cDNA-infected cells and fibulin-3 cDNA-infected cells. *P < 0.05.
Effects of fibulin-3 on the major EMT-related genes
The activation of the EMT program promotes tumor-cell invasion and metastasis. Therefore, we tested if gain or loss of fibulin-3 function could affect several major EMT-related genes, such as E-cadherin, N-cadherin, vimentin, Snail, Slug, Zeb2, and Twist. Analysis using qPCR and western blotting indicated that increased expression of fibulin-3 in the low invasive clone subline HeLa-25 exhibited significantly decreased the mRNA and protein levels of E-cadherin, the epithelial hallmark of EMT that mediates cell-cell interactions, and the mRNA and protein levels of N-cadherin and vimentin were increased. N-cadherin is commonly found in cancer cells and provides a mechanism for transendothelial migration. Vimentin was used as a tumor marker to identify mesenchyme. Snail, Slug, Zeb2, and Twist belong to the family of transcription factors that bind to E-box motifs to repress E-cadherin transcription and regulate EMT. Upon fibulin-3 knockdown, levels of Snail, Slug, Zeb2, and Twist all decreased, at the same time, upon fibulin-3 overexpression, they were all upregulated. As a result, gain or loss of fibulin-3 function could affect several major EMT-related genes, fibulin-3 knockdown could inhibit the process of EMT, and conversely, fibulin-3 overexpression could promote the process of EMT (Fig. 7). To further determine the correlations between fibulin-3 and the studied markers E-cadherin, N-cadherin, and Vimentin, we performed IHC experiments in clinical samples (Fig. 8) and revealed that fibulin-3 was significantly positively correlated with N-cadherin and Vimentin, but negatively correlated with E-cadherin. Next, we studied the association of fibulin-3 with the transcription factors closely related to EMT using transcriptomics data from the TCGA repositories. Fibulin-3 showed a significant positive correlation with the transcription factors that were assayed in Fig. 9.
Effects of fibulin-3 knockdown and overexpression on key EMT hallmarks. After lentivirus transfection, EMT markers including E-cadherin, N-cadherin, vimentin, Snail, Slug, Zeb2, and Twist were measured by (A) western blotting (cropped blot) and (B) real-time q-RT-PCR in the lentivirus transfection systems. *P < 0.05.
The correlations between fibulin-3 and the studied EMT markers E-cadherin, N-cadherin and Vimentin. E-cadherin (A), N-cadherin (B), and Vimentin (C) expression in normal human cervical tissues and cervical carcinoma tissues were measured by IHC. (D) Pearson’s product-moment correlation coefficient analysis showed that fibulin-3 was significantly positively correlated with N-cadherin and Vimentin, but negatively correlated with E-cadherin.
The correlations between fibulin-3 and the transcription factors closely related to EMT. Using transcriptomics data from the TCGA repositories, (A) fibulin-3 was significantly positively correlation with the transcription factors Snail 1 and Snail 2, (B) the transcription factors Twist1 and Twist2, and (C) the transcription factors Zeb1 and Zeb2.
Effects of fibulin-3 on the PI3K/AKT and ERK pathway
Using pharmacological signal pathway inhibitors, we studied the PI3K/AKT and ERK signaling pathways, which are tightly associated with EMT22. Fibulin-3 cDNA- and shRNA-infected cells were serum-starved and treated with DMSO and the inhibitors PD98059 (20 μM, ERK inhibitor) or LY294002 (20 μM, PI3K/AKT inhibitor), for 48 h. Then, Boyden Chamber migration and invasion assays were performed. In fibulin-3 overexpression groups, cell migration and invasion abilities were strongly decreased by incubation with LY294002, but not with PD98059; nevertheless, in fibulin-3 downregulation groups, cell migration and invasion abilities had no significant differences upon treatment with DMSO, LY294002, and PD98059 (Fig. 10). LY294002 remarkably reduced the levels of PI3K, AKT, and mTOR phosphorylation, and accordingly changed the expression of EMT hallmarks, but had no influence on fibulin-3 expression, compared to the DMSO control (Fig. 11A). Thus, the expression of fibulin-3 was not regulated by PI3K/AKT signaling, but the effect of fibulin-3 on EMT was inhibited by the phosphorylation pathway inhibitor LY294002. Regarding the ERK pathway, PD98059 treatment decreased ERK phosphorylation, but had no effect on fibulin-3 expression and could not block fibulin-3-mediated EMT promotion (Fig. 11B). Further experiments revealed that fibulin-3 upregulation increased the PI3K, AKT, and mTOR phosphorylation levels, but had no effect on the ERK phosphorylation level; at the same time, fibulin-3 knockdown reduced their phosphorylation levels, but also had no effect on the ERK phosphorylation level (Fig. 11C). In summary, fibulin-3 could facilitate cervical cancer cell development and metastasis by the PI3K/AKT/mTOR signaling pathway.
Effects of fibulin-3 knockdown and overexpression on the PI3K/AKT/mTOR and ERK pathway as assessed by Boyden chambers. Fibulin-3 cDNA-infected cells, which were serum-starved and treated with DMSO or the indicated inhibitors PD98059 and LY294002 for 48 h, were measured by (A) cell migration assays without Matrigel and (B) cell invasion assays with Matrigel. Fibulin-3 shRNA-infected cells, which were serum-starved and treated with DMSO and the indicated inhibitors PD98059 and LY294002 for 48 h, were subjected to (C) cell migration assays without Matrigel and (D) cell invasion assays with Matrigel. (E) The average counts of migrating and invading fibulin-3 cDNA-infected cells were strongly decreased upon incubation with LY294002, but not with PD98059. (F) The average counts of migrating and invading fibulin-3 shRNA-infected cells were not significantly different among cells treated with DMSO, LY294002, and PD98059.
Effects of fibulin-3 knockdown and overexpression on the PI3K/AKT/mTOR and ERK pathway as determined by western blotting (cropped blot). (A) LY294002 treatment remarkably reduced the levels of PI3K, AKT, and mTOR phosphorylation, and accordingly changed the expression of EMT markers, but had no influence on fibulin-3 expression compared to the DMSO control. (B) PD98059 decreased ERK phosphorylation level but had no effect on fibulin-3 expression and could not block fibulin-3-mediated promotion of EMT. (C) Fibulin-3 knockdown reduced the PI3K, AKT, and mTOR phosphorylation levels to deactivate the PI3K/AKT/mTOR pathway but had no effect on the ERK phosphorylation levels; in contrast, fibulin-3 upregulation increased the PI3K, AKT, and mTOR phosphorylation levels to activate the PI3K/AKT/mTOR pathway, but also had no effect on the ERK phosphorylation level.
Correlations between fibulin-3 and p-PI3K, p-AKT, and p-mTOR
The representative immunohistochemical staining of p-PI3K, p-AKT, and p-mTOR in cervical cancer and normal tissues were determined by IHC. Like fibulin-3 expression, the expressions of p-PI3K, p-AKT, and p-mTOR in cervical cancer tissues were much higher than that in most cervical normal tissues (Fig. 12A–C). To assess the correlations between fibulin-3 and p-PI3K, p-AKT, and p-mTOR, Pearson correlation coefficients were calculated and showed significantly positive correlations between fibulin-3 and p-PI3K, p-AKT, and p-mTOR (Fig. 12D).
Expression of p-PI3K, p-AKT, and p-mTOR in human cervical tissues and the correlations between these proteins and fibulin-3. The expressions of p-PI3K (A), p-AKT (B), and p-mTOR (C) in normal cervical tissues and cervical carcinoma tissues were measured by IHC. (D) Pearson’s correlation coefficient analysis was used to separately analyze the correlations between p-PI3K, p-AKT, and p-mTOR and fibulin-3, and significant positive correlations were observed.
Discussion
In this study, fibulin-3 overexpression was significantly related with malignant phenotype and poor prognosis of cervical carcinoma in clinical samples, and high fibulin-3 expression was positively correlated with proliferation capacity and invasion ability of cervical cancer cells. Fibulin-3 could facilitate cervical cancer cell development and metastasis by eliciting EMT and activating the PI3K-Akt-mTOR signal transduction pathway.
Higher fibulin-3 expression was detected in cervical cancer tissues and the highly invasive clone sublines than in the normal cervical tissues and low invasive clone sublines. Moreover, high fibulin-3 expression was significantly related to poor differentiation, positive lymph node metastasis, and high clinicopathologic staging of cervical cancer. Similar outcomes were reported by En-lin S et al.23 that fibulin-3 up-regulation was markedly related to positive lymph node metastasis, vascular invasion, and poor prognosis in patients with cervical carcinoma. They also found that fibulin-3 overexpression promoted angiogenesis, which is mediated by VEGF upregulation24. For our study, the experiments revealed that fibulin-3 facilitated cervical cancer cell development and metastasis by eliciting EMT and activating the PI3K-Akt-mTOR signal transduction pathway. In ovarian cancer, fibulin-3 expression was upregulated, and its overexpression was significantly associated with high clinicopathologic stage, low degree of tissue differentiation, positive lymph node metastasis, and poor prognosis of ovarian cancer patients7. Fibulin-3 also acts as a positive regulator to contribute to ovarian cancer invasion and metastasis by activating AKT signaling8. In contrast, in breast cancer, fibulin-3 expression is reduced or even abolished in 57–62.5% of these tumors, and its reduced expression was significantly correlated with poor disease-free and overall survival in patients with breast cancer25. Functionally, high fibulin-3 levels inhibit TGF-β-induced EMT, migration, invasion, and endothelial permeability in breast cancer26. In human endometrial carcinoma, fibulin-3 was thought to be a tumor suppressor, decreased the secretion of MMPs, and thwarted endometrial cancer cell proliferative and invasive capacities17. Furthermore, fibulin-3 could suppress EMT in EC (endometrial cancer) cells in vitro and in vivo through Wnt/β-catenin signaling27. Apparently, the function of fibulin-3 in carcinogenesis was determined by the tumor type. The role of fibulin-3 in tumor development is tissue specific, which may be due to the fact that the paradoxical roles of the tumor microenvironment during specific cancer progression determine the different functions of tumor-related genes28.
In the 1980s, the EMT was first recognized by Betty Hay and was termed as a feature of embryogenesis. Later, EMT was also found to take place in during wound healing, organ fibrosis, and cancer invasion. Cancer cells in a primary tumor lose cell-cell adhesion mediated by E-cadherin repression, break through the basement membrane with increased invasive properties, and enter the bloodstream through intravasation. Cells undergoing EMT gain stem cell-like properties, which increase tumorigenic and proliferative potential29,30. In our study, we found that fibulin-3 overexpression promoted EMT and enabled cervical cancer cells to acquire more mesenchymal cells properties with more invasive and metastatic capacities. Similar results were previously found in ovarian carcinoma8. The RT2 Profiler EMT PCR array was performed in a fibulin-3 RNA interference system in ovarian cancer cells and showed that fibulin-3 downregulation hindered the process of EMT and reduced the proliferative, invasive, and metastatic capacities of ovarian cancer cells. In human osteosarcoma9, fibulin-3 could facilitate osteosarcoma cells to further invade and disseminate, leading to the occurrence of EMT by regulating and controlling H3K4me3 methylation modifications. In contrast, in endometrial carcinoma cells27 and lung cancer stem cells16, the opposite conclusion was made: that fibulin-3 overexpression blocked the EMT and abrogated the invasive and metastatic abilities of tumor cells. In summary, the functions of fibulin-3 during EMT of specific tumor types may also be affected by the specific tumor microenvironment, with the dual paradoxical role of either promoting or restraining EMT depending on the cancer type.
The PI3K/Akt/mTOR pathway is overactive in many cancers, playing a crucial role in reducing apoptosis and allowing proliferation, ultimately influencing the invasion, metastasis, and aggressiveness of cancer cells31. In our study, LY294002, an inhibitor of the PI3K/AKT pathway, remarkably hampered the increased cell migration and invasion abilities caused by fibulin-3 overexpression, and inactivating this signaling pathway blocked the process of EMT. Correspondingly, fibulin-3 upregulation could activate the PI3K/Akt/mTOR pathway, promote EMT, and increase the migration and invasion abilities of cervical cancer cells; at the same time, fibulin-3 knockdown could inactivate the PI3K/Akt/mTOR pathway, inhibit EMT, and decrease the migration and invasion abilities of cervical cancer cells. In ovarian cancer, fibulin-3, as a positive regulator, was found to boost cancer cell proliferation, invasion, and diffusion by activating AKT signaling8. In lung cancer, fibulin-3 suppresses both the EMT process and the ability of lung cancer stem cells to self-renew by modulating the IGF1R/PI3K/AKT/GSK3β pathway16. In nasopharyngeal carcinomas, fibulin-3 suppressed cell migration and invasion by blocking the PI3K/AKT pathway18. Collectively, regardless of the role of fibulin-3 in the development of a specific cancer, the PI3K/AKT pathway was always involved, and fibulin-3 was one of the regulators of the PI3K/AKT pathway.
In conclusion, there was a significantly positive correlation between fibulin-3 overexpression and malignant phenotype and poor prognosis of cervical carcinoma. Fibulin-3 could facilitate cervical cancer cell growth, invasion, and spread, by stimulating the process of EMT and triggering the PI3K-Akt-mTOR signal transduction pathway. Additional research on fibulin-3 may help in designing treatments for cervical cancer and to improve patient prognosis.
Change history
10 July 2023
This article has been retracted. Please see the Retraction Notice for more detail: https://doi.org/10.1038/s41598-023-38389-y
References
Bobdey, S., Sathwara, J., Jain, A. & Balasubramaniam, G. Burden of cervical cancer and role of screening in India. Indian J Med Paediatr Oncol. 37, 278–285 (2016).
Suneja, G. et al. American Brachytherapy Society: Brachytherapy treatment recommendations for locally advanced cervix cancer for low-income and middle-income countries. Brachytherapy. 16, 85–94 (2017).
Mezei, A. K. et al. Cost-effectiveness of cervical cancer screening methods in low- and middle-income countries: A systematic review. Int J Cancer. 141, 437–446 (2017).
Zhang, Y. & Marmorstein, L. Y. Focus on molecules: fibulin-3 (EFEMP1). Exp Eye Res. 90(3), 374–375 (2010).
Timpl, R., Sasaki, T., Kostka, G. & Chu, M. L. Fibulins: a versatile family of extracellular matrix proteins. Nat Rev Mol Cell Biol. 4, 479–489 (2003).
Kobayashi, N. et al. A comparative analysis of the fibulin protein family. Biochemical characterization, binding interactions, and tissue localization. J Biol Chem. 282, 11805–11816 (2007).
Chen, J., Wei, D., Zhao, Y., Liu, X. & Zhang, J. Overexpression of EFEMP1 Correlates with Tumor Progression and Poor Prognosis in Human Ovarian Carcinoma. PLoS One. 8(11), e78783 (2013).
Yin, X. et al. EFEMP1 promotes ovarian cancer cell growth, invasion and metastasis via activated the AKT pathway. Oncotarget. 7(30), 47938–47953 (2016).
Wang, S. et al. Fibulin-3 promotes osteosarcoma invasion and metastasis by inducing epithelial to mesenchymal transition and activating the Wnt/β-catenin signaling pathway. Sci Rep. 7(1), 6215 (2017).
Seeliger, H. et al. EFEMP1 expression promotes in vivo tumor growth in human pancreatic adenocarcinoma. Mol Cancer Res. 7(2), 189–198 (2009).
Hu, B., Thirtamara-Raiamani, K. K., Sim, H. & Viapiano, M. S. Fibulin-3 is uniquely upregulated in malignant gliomas and promotes tumor cell motility and invasion. Mol Cancer Res. 7(11), 1756–1770 (2009).
Dou, C. Y. et al. EFEMP1 inhibits migration of hepatocellular carcinoma by regulating MMP2 and MMP9 via ERK1/2 activity. Oncol Rep. 35(6), 3489–3495 (2016).
Luo, R. et al. Decrease of fibulin-3 in hepatocellular carcinoma indicates poor prognosis. PLoS One. 8(8), e70511 (2013).
Zhu, X. J., Liu, J., Xu, X. Y., Zhang, C. D. & Dai, D. Q. Novel tumor-suppressor gene epidermal growth factor-containing fibulin-like extracellular matrix protein 1 is epigenetically silenced and associated with invasion and metastasis in human gastric cancer. Mol Med Rep. 9(6), 2283–2292 (2014).
Chen, X. et al. Fibulin-3 suppresses Wnt/β-catenin signaling and lung cancer invasion. Carcinogenesis. 35(8), 1707–1716 (2014).
Kim, I. G. et al. Fibulin-3-mediated inhibition of epithelial-to-mesenchymal transition and self-renewal of ALDH+ lung cancer stem cells through IGF1R signaling. Oncogene. 33(30), 3908–3917 (2014).
Yang, T. et al. Epigenetic inactivation of EFEMP1 is associated with tumor suppressive function in endometrial carcinoma. PLoS One. 8(6), e67458 (2013).
Hwang, C. F. et al. Fibulin-3 is associated with tumour progression and a poor prognosis in nasopharyngeal carcinomas and inhibits cell migration and invasion via suppressed AKT activity. J Pathol. 222(4), 367–379 (2010).
Chen, J. et al. Targeting SPARC by lentivirus-mediated RNA interference inhibits cervical cancer cell growth and metastasis. BMC Cancer. 12(1), 464 (2012).
Yang, X. S. et al. The correlation of bone morphogenetic protein 2 with poor prognosis in glioma patients. Tumor Biol. 35, 11091–11095 (2014).
Albini, A. Extracellular Matrix Invasion in Metastases and Angiogenesis: Commentary on the Matrigel “Chemoinvasion Assay”. Cancer Res. 76(16), 4595–4597 (2016).
Pearlman, R. L., Montes de Oca, M. K., Pal, H. C. & Afaq, F. Potential therapeutic targets of epithelial-mesenchymal transition in melanoma. Cancer Lett. 391, 125–140 (2017).
En-lin, S., Sheng-guo, C. & Hua-qiao, W. The expression of EFEMP1 in cervical carcinoma and its relationship with prognosis. Gynecol Oncol. 117(3), 417–422 (2010).
Song, E. L. et al. EFEMP1 expression promotes angiogenesis and accelerates the growth of cervical cancer in vivo. Gynecol Oncol. 121(1), 174–180 (2011).
Sadr-Nabavi, A. et al. Decreased expression of angiogenesis antagonist EFEMP1 in sporadic breast cancer is caused by aberrant promoter methylation and points to an impact of EFEMP1 as molecular biomarker. Int J Cancer. 124(7), 1727–1735 (2009).
Tian, H., Liu, J., Chen, J., Gatza, M. L. & Blobe, G. C. Fibulin-3 is a novel TGF-β pathway inhibitor in the breast cancer. Oncogene. 34(45), 5635–5647 (2015).
Yang, T. et al. EFEMP1 is repressed by estrogen and inhibits the epithelial-mesenchymal transition via Wnt/β-catenin signaling in endometrial carcinoma. Oncotarget. 7(18), 25712–25725 (2016).
Quail, D. F. & Joyce, J. A. Microenvironmental regulation of tumor progression and metastasis. Nat Med. 19(11), 1423–1437 (2013).
Lamouille, S., Xu, J. & Derynck, R. Molecular mechanisms of epithelial-mesenchymal transition. Nat Rev Mol Cell Biol. 15(3), 178–196 (2014).
Kong, D., Li, Y., Wang, Z. & Sarkar, F. H. Cancer Stem Cells and Epithelial-to-Mesenchymal Transition (EMT)-Phenotypic Cells: Are They Cousins or Twins? Cancers (Basel). 3(1), 716–729 (2011).
Ahmad, A. et al. Targeted regulation of PI3K/Akt/mTOR/NF-κB signaling by indole compounds and their derivatives: mechanistic details and biological implications for cancer therapy. Anticancer Agents Med Chem. 13(7), 1002–1013 (2013).
Acknowledgements
This study was supported by National Nature Science Foundation of China (81671434 and 81503298) and Shandong Natural Science Foundation (2R2013HM060). The fonders had no role in study design, data collection and analysis, decision to publish, or preparation of the manuscript.
Author information
Authors and Affiliations
Contributions
Conceived and designed the experiments: J.C. M.S. Performed the experiments: J.L. C.Q. X.L. C.L. Analyzed the data: J.L. C.L. X.L. M.S. Wrote the paper: J.C. C.Q. M.S.
Corresponding author
Ethics declarations
Competing Interests
The authors declare no competing interests.
Additional information
Publisher's note: Springer Nature remains neutral with regard to jurisdictional claims in published maps and institutional affiliations.
This article has been retracted. Please see the retraction notice for more detail: https://doi.org/10.1038/s41598-023-38389-y
Rights and permissions
Open Access This article is licensed under a Creative Commons Attribution 4.0 International License, which permits use, sharing, adaptation, distribution and reproduction in any medium or format, as long as you give appropriate credit to the original author(s) and the source, provide a link to the Creative Commons license, and indicate if changes were made. The images or other third party material in this article are included in the article’s Creative Commons license, unless indicated otherwise in a credit line to the material. If material is not included in the article’s Creative Commons license and your intended use is not permitted by statutory regulation or exceeds the permitted use, you will need to obtain permission directly from the copyright holder. To view a copy of this license, visit http://creativecommons.org/licenses/by/4.0/.
About this article
Cite this article
Li, J., Qi, C., Liu, X. et al. RETRACTED ARTICLE: Fibulin-3 knockdown inhibits cervical cancer cell growth and metastasis in vitro and in vivo. Sci Rep 8, 10594 (2018). https://doi.org/10.1038/s41598-018-28906-9
Received:
Accepted:
Published:
DOI: https://doi.org/10.1038/s41598-018-28906-9
- Springer Nature Limited
This article is cited by
-
Multidimensional outlook on the pathophysiology of cervical cancer invasion and metastasis
Molecular and Cellular Biochemistry (2023)
-
Fibulin-3 knockout mice demonstrate corneal dysfunction but maintain normal retinal integrity
Journal of Molecular Medicine (2020)