Abstract
Background
Near-infrared spectroscopy is used in the assessment of regional splanchnic oxygen saturation (rsSO2), but solid reference values are scarce. We aimed to establish reference values of rsSO2 for preterm infants during the first week after birth, both crude and modeled based on predictors.
Methods
We included infants with gestational age (GA) <32 weeks and/or birth weight <1200 g. We excluded infants who developed necrotizing enterocolitis or sepsis or who died. In the first week after birth, we determined a daily 2-h mean of rsSO2 to assess its associations with sex, GA, postnatal age (PNA), small-for-gestational age (SGA) status, patent ductus arteriosus, hemoglobin, nutrition, and head circumference at birth and translated those into a prediction model.
Results
We included 220 infants. On day 1, the mean ± SD rsSO2 value was 48.2% ± 16.6. The nadir of rsSO2 was on day 4 (38.7% ± 16.6 smoothed line) to 5 (37.4%±17.3, actual data), after which rsSO2 increased to 44.2% ± 16.6 on day 7. The final model of the reference values of rsSO2 included the following coefficients: rsSO2 = 3.2 − 7.0 × PNA + 0.8 × PNA2 − 4.0 × SGA + 1.8 × GA.
Conclusions
We established reference values of rsSO2 for preterm infants during the first week after birth. GA, PNA, and SGA affect these values and need to be taken into account.
Impact
-
Regional splanchnic oxygen saturation is lower in infants with a lower gestational age and in small-for-gestational age infants.
-
Regional splanchnic oxygen saturation decreases with a higher postnatal age until day 4 after birth and then increases until day 7 after birth.
-
Gestational age, postnatal age, and small-for-gestational age status affect regional splanchnic oxygen saturation and need to be taken into account when interpreting regional splanchnic oxygen saturations using NIRS.
-
Reference values for infant regional splanchnic oxygen saturation can be computed with a formula based on these variables, as provided by this study.
Similar content being viewed by others
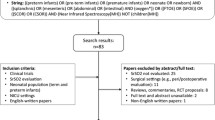
Introduction
At the neonatal intensive care unit (NICU), preterm infants are hospitalized for long periods with risk of severe complications and life-threatening events.1,2 To ensure successful treatment and early diagnosis of these complications, various monitoring tools have been introduced to assess individual physiological functioning at the NICU. Near-infrared spectroscopy (NIRS) is such a tool. This non-invasive technique uses near-infrared light to distinguish oxygenated from deoxygenated hemoglobin. Biological tissues are relatively transparent to near-infrared light and this light is absorbed by molecules such as hemoglobin. Based on this, NIRS measures the regional oxygen saturation of the underlying tissue.3 As NIRS measures a tissue vascular bed that mostly contains venous blood, NIRS values result from a balance between arterial oxygen supply and tissue oxygen use. NIRS can, therefore, be used to identify organs at risk of tissue hypoxia.4
In preterm infants, the intestines are frequently exposed to episodes of hypoxia, which has been associated with feeding intolerance and necrotizing enterocolitis.5,6,7,8 Therefore, a diagnostic and prognostic use of regional splanchnic oxygen saturation (rsSO2) monitoring has been suggested. RsSO2 has also been studied to guide nutritional management, to investigate the effect of anemia and blood transfusions, and to examine the effect of various medications such as ibuprofen on splanchnic oxygenation.9,10,11,12,13,14,15 However, the use of rsSO2 in clinical care is currently limited, in part due to a lack of solid reference values.16,17 Only a few studies in small samples are available as basis for the current reference values. These values suggest that rsSO2 is associated with gestational age (GA) and postnatal age (PNA). However, rsSO2 may differ between monitors. In order to optimize the use of rsSO2, we aimed to establish reference values of rsSO2 using INVOS 5100c monitors with neonatal sensors for preterm infants during the first week after birth, both crude and modeled based on predictors.
Methods
Patient population
In this observational cohort study, we included all infants with a GA <32 weeks and/or a birth weight (BW) <1200 g who were admitted to our NICU in the first week after birth between January 2016 and December 2017. Infants were excluded in case of chromosomal or congenital abnormalities and gastrointestinal diseases such as volvulus and spontaneous intestinal perforation. Infants with necrotizing enterocolitis (Bell’s stage ≥2)18 were also excluded because of the association of rsSO2 in the first week after birth and subsequent development of necrotizing enterocolitis.7,19 Data from infants who developed clinical sepsis (sepsis symptoms with a positive blood culture and/or C-reactive protein >20 mg/L) were excluded from 1 day prior to diagnosis and all subsequent days because intestinal perfusion has been shown to be different during these days in these infants.20 In case an infant died, data from that day were excluded. The medical ethical committee of the University Medical Center Groningen approved this study.
Data collection
As part of standard clinical care, we measured rsSO2 during the first week after birth for at least 2–3 times a day for a minimum of 2 h using an INVOS 5100c monitor (INVOS™ 5100C [Medtronic, Dublin, Ireland]). The neonatal INVOS™ SomaSensor was placed infraumbilically, on top of Mepitel® sheets (Mölnlycke, Sweden) to protect the skin. RsSO2 measurements were halted if infants had an umbilical catheter taped to the infraumbilical skin or during infraumbilical skin irritation. RsSO2 values were saved in an offline pseudonymized database every 5 s.
Sensor placement was checked by the attending nurse and verified every morning by a researcher. We calculated a daily 2-h mean of rsSO2 before or, when unavailable, closest to the sensor verification. We selected a 2-h period with at least 80% of available data. Artifacts were manually removed and defined as misplacement of the sensor, unexplained sudden non-physiological change of the values, or a lack of physiological variability of the values, again indicating sensor misplacement. Regional cerebral oxygen saturation (rcSO2) was simultaneously measured on the left or right frontoparietal side of the head and calculated in the same period and with an identical procedure as rsSO2. Peripheral arterial oxygen saturation (SpO2) was simultaneously measured using Nellcor® pulse oximeters (Medtronic, Dublin, Ireland), with target range 90–92% and alarm settings at 86 and 93% in case of oxygen therapy.
We further collected data on pregnancy, birth, and the early neonatal period from the hospital records. Data on pregnancy regarded multiple gestation, parity, and mode of delivery. Data on birth regarded BW, small-for-gestational age (SGA) status defined as <p10 according to Dutch reference values,21 GA, sex, head circumference at birth (converted to Z-scores according to Niklasson),22 and Apgar scores at 1 and 5 min. Data on the early neonatal period regarded SNAPPE-II score23; periventricular or intraventricular hemorrhage present in the first week after birth categorized according to the modified Papile classification24; patent ductus arteriosus (PDA) (categorized as no clinical signs of PDA/not assessed and no PDA after echocardiographic assessment, non-hemodynamic significant PDA, and hemodynamic significant PDA (hsPDA) that needed treatment); daily weight Z-scores according to Dutch reference values21; type of nutrition (categorized as ≥50% mother’s milk, <50% mother’s milk, or nil by mouth); and hemoglobin level for a period from 12 h before to 12 h after the rsSO2 measurement.
Data handling and statistical analyses
To allow making valid and efficient inferences, missing rsSO2 values were imputed.25 We imputed the missing rsSO2 values using predictive mean matching26 based on the following variables: GA, SNAPPE-II score as measure of disease severity, daily weight, SpO2, previous and future rsSO2 values, rcSO2, and hemoglobin levels. For the imputed values based on these variables, we further assumed no dependency on other variables, i.e., further missingness at random. Missing values were assessed by a repetitive total of 20 imputations, and all analyses were based on the pooled results of these imputations.
Mean rsSO2 values were graphically presented for the first week after birth and aberrant rsSO2 values were indicated as higher or lower than one standard deviation. We present the data of rsSO2 with a “smoothed” line. In this line, the measurement error per measurement is reduced by taking into account the adjacent measurements on the same child, enabling a more precise estimate of patterns. Because of this reduction in random errors, smoothed estimates may slightly vary from observed ones.
We also calculated the mean and SD of the actual measured rsSO2 values for each day and determined on which day the nadir occurred. Statistical differences of rsSO2 between various days were tested using Student’s t test and are presented in a Supplemental Table.
Next, we constructed a model to predict the course of the rsSO2 during the first week. To this end, we assessed associations of rsSO2 with PNA (in days after birth), sex (male/female), GA (weeks), SGA (yes/no), PDA (yes/no), hemoglobin level, nutrition (≥50% mother’s milk yes/no), and head circumference Z-score at birth according to GA, using multilevel models (due to the longitudinal study design). As first step, we determined the linear as well as quadratic effect of PNA to determine the best model using goodness-of-fit criteria.27 Next we assessed the associations of rsSO2 with all individual variables separately, only adjusting for PNA. Subsequently all variables with a P value < 0.1 were included into a multivariable model. We then reduced this multivariable model to a final prediction model using a backward elimination procedure, with variables eliminated based on P < 0.05.
To validate our final model, we performed a post hoc power analysis using a simulation-based approach with Monte Carlo facilities regarding the detection of the quadratic growth with covariates.28 This power analysis, performed over the 20 imputations, showed that the current study had a power of at least 90% to detect the type of growth, i.e., course of rsSO2 during the first 7 days, in analogy to growth curves (linear and quadratic) and the associations with GA and SGA. This shows that our study had adequate power to reject the null hypothesis of zero correlation.
We used IBM SPSS Statistics 23 (IBM Corp., Armonk, NY), SAS version 9.2 (SAS Institute INC., Cary, NC), and Mplus version 8.4 (Muthen and Muthen, Los Angeles, CA) for imputation, multilevel analysis, and power analysis, respectively. GraphPad Prism 7.02 (GraphPad Software Inc., La Jolla, CA) was used for graphical displays.
Results
Patient population
Out of 278 infants, we excluded 58 infants because of chromosomal abnormalities (n = 10), gastrointestinal diseases including necrotizing enterocolitis (n = 37), congenital heart defects (n = 3), sepsis (n = 7), death on day of birth (n = 1), or combinations of these. Patient characteristics are shown in Table 1. Of the 220 included infants, 50 infants had rsSO2 measurements on all 7 days, while for 41 infants we imputed rsSO2 values on all 7 days. For the remaining 129 infants, rsSO2 values were partly imputed. Reasons for missing values were presence of an infraumbilical taped umbilical catheter, the attending doctors’ preference to remove the sensor for unknown reason, death between day 2 and 7 after birth, sepsis between day 3 and day 7 after birth, discharge from NICU, no NIRS device available, artifacts and insufficient NIRS data, or an unknown cause (Supplemental Table S1).
Reference values for rsSO2
On the first day after birth, the mean (±SD) rsSO2 value was 48.2% (±16.6). Mean rsSO2 decreased during the first days after birth. For the smoothed line, the nadir of rsSO2 was on day 4 (38.7% ± 16.6), after which the mean rsSO2 increased to 44.2% (±16.6) on day 7 (Fig. 1). In Supplemental Fig. S1, we present the curve for the 50 infants with measurements on all days. Of note, mean SpO2 levels ranged between 93.6 and 95.3% during the first 7 days after birth (Table 1). In Supplemental Table S2, we present the mean (±SD) of the actual measured rsSO2 values for each day, including the statistical significance of differences between various days. The nadir was now on day 5 (37.4% ± 17.3).
For the prediction model, we first assessed the associations of GA, SGA, PDA, hemoglobin level, nutrition, and head circumference at birth with rsSO2, only adjusted for PNA and PNA-squared. This showed that PNA, GA, SGA, PDA, and head circumference at birth were associated with rsSO2 at P < 0.1 (Table 2). Next, we entered these variables in a multivariable model, which we reduced to a final prediction model by using a backward elimination procedure. This yielded that PNA, GA, and SGA remained significantly associated with rsSO2 (Table 3). This resulted in the formula: rsSO2 = 3.2 − 7.0 × PNA(i) + 0.8 × PNA2(i) − 4.0 × SGA + 1.8 × GA (i = day 1–7, GA: weeks, SGA: 0 = No, 1 = Yes). Additional information of all studied variables for the prediction model can be found in Supplemental Table S3. This formula represents that, up to the fourth day after birth, mean rsSO2 values decline and then increase toward day 7 after birth. Furthermore, mean rsSO2 values were lower for infants with a lower GA and for infants who were SGA (Fig. 2).
The course of mean regional splanchnic oxygen saturation of 4 subgroups of infants, illustrating differences between infants born at 24 and 32 weeks, with and without SGA. Regression coefficients of GA and SGA status are presented in Table 3. RsSO2 regional splanchnic oxygen saturation, GA gestational age, SGA small-for-gestational age.
Discussion
In this study, we established reference values of rsSO2 for preterm infants during the first week after birth. GA, PNA, and SGA affected these values and need to be taken into account when interpreting rsSO2 using NIRS.
In this study, rsSO2 decreased from day 1 after birth until day 4 after which rsSO2 increased with increasing PNA until day 7 after birth. These results mostly confirm the findings by McNeill et al. and Cortez et al. regarding the first week after birth16,17 but now in a considerably larger sample with more precise estimates. Our results contrast those of Patel et al. who found a different course of rsSO2, i.e., an increase of rsSO2 until day 3, after which rsSO2 decreased until day 7 after birth.7 This different course could be explained by the fact that Patel et al. used another site of placing their sensors, i.e., right lower abdomen, whereas we measured centrally in the infraumbilical region. Furthermore, they prospectively included infants only within the first 24 h after birth. This may have led to bias, by exclusion of the sickest infants as parents may not have been asked informed consent. Finally, they measured rsSO2 for only 5 min during a “steady state” without episodes of apnea, bradycardia, or arterial oxygen desaturation, which may occur frequently in the first days after birth and may have resulted in a lower rsSO2 in our study.7 Therefore, our results may better represent all preterm infants admitted to the NICU, resulting in a nadir on day 4.
We found several variables to be associated with rsSO2 for preterm infants in the first week after birth, modeled for predictors. Of these variables, PNA was associated with rsSO2 and a lower GA was associated with lower rsSO2 values as has been demonstrated before.16,17 This study adds that SGA was also associated with lower rsSO2 values. We did not find any associations between rsSO2 and sex and hemoglobin level as showed before.16,17 Similarly, this study demonstrated that confirmed hsPDA, nutrition, and head circumference Z-score at birth were not associated with rsSO2. In contrast, McNeill et al. reported a moderate-strength positive association between hemoglobin and rsSO2.16 As rsSO2 is expressed as the ratio of oxygenated to total hemoglobin, a positive association between rsSO2 and hemoglobin seems plausible. We may not have found this association in our multivariable models, because hemoglobin levels were strictly controlled and therefore did not vary enough to show a significant relation with rsSO2.
We found an initial decrease in rsSO2 in the first days after birth, followed by an increase from day 5 onwards. This course may be affected by several clinical variables in various degrees. Of these variables, it is known that PDA influences mesenteric hemodynamics of preterm infants.29,30 Ledo et al. reported the course of rsSO2 in the first week of life, stratified for PDA status, and showed an initial decrease until the third day after birth in all groups.30 A subsequent increase in rsSO2 was only observed in infants with closure of the ductus arteriosus, either spontaneously or following treatment with ibuprofen. Thus the initial decrease in rsSO2 suggests a maximal effect of the left-to-right ductal shunt after full transition after birth, resulting in decreased mesenteric blood flow.31,32,33 With ductal closure within 2–6 days after birth, mesenteric blood flow restores and rsSO2 increases.33 Based on the foregoing, an association between hsPDA and rsSO2 could be assumed. In our study, however, we found no association between hsPDA and rsSO2 when corrected for other variables. This association may have been suppressed by GA in the final model, as GA is associated with both rsSO2 and a higher risk of an hsPDA. Alternatively, hsPDA may have been underdiagnosed during the first week in our cohort.
The initial decrease in rsSO2 may also be explained by growth and intestinal maturation processes. Although there are no clinical studies available using simultaneous Doppler and NIRS measurements, an increase in mesenteric blood flow has been observed from birth onwards, while we demonstrated an initial decrease in rsSO2, suggesting that oxygen extraction increases to a greater extent than blood flow in the first days after birth. In the absence of PDA, this decrease in rsSO2 may be explained by increased intestinal activity, e.g., digestion–absorption in the presence of increasing volumes of enteral nutrition, immunological processes in the presence of the developing microbiome, and intestinal tissue growth.
The question remains why GA and SGA affect rsSO2. As NIRS predominantly measures venous hemoglobin, rsSO2 represents a balance between arterial oxygen supply and tissue oxygen consumption. The association of SGA with lower rsSO2 values may be explained by a lower splanchnic perfusion, as a result of a preferential blood flow to the brain, as seen during and after34 fetal growth restriction pregnancies often preceding prematurity and SGA.35 The association between lower GA and lower rsSO2 values suggests that the maturation of the mesenteric vasculature is GA dependent.16 This is supported by various studies in which abdominal blood flow was demonstrated to increase with increasing GA.36,37 However, as we did not measure blood flow in our study, we could not confirm this explanation. In tandem, SGA infants and infants with lower GA have the highest need for intestinal growth and development, potentially resulting in an increased oxygen demand. Nevertheless, we propose that the associations of lower GA and SGA with lower rsSO2 are mainly a result of reduced intestinal perfusion.
This study on reference values of rsSO2 for preterm infants has a number of major strengths, such as having the largest sample to date, collection of data in a clinical care setting, thus representing the actual rsSO2 found in preterm infants at the NICU, and the inclusion of various variables to assess associations with rsSO2. However, this study has also some limitations. First, we had to impute missing values in several infants on various days. Nevertheless, imputation of missing values, using other related variables to make an assumption of the missing value, is a frequently used and well-accepted method. Moreover, the course of rsSO2 was not different after we imputed data compared with only the non-imputed data (data not shown). Second, the broad range of values within one SD suggests to also investigate other aspects of the value, such as variability or relation to cerebral oxygenation. Finally, the reference values found in this study may be dependent on different factors such as the position and type of the sensor, duration of the measurement, type of device, and the SpO2 value.38 Nonetheless, although rsSO2 values could differ between different units, the associations with PNA, GA, and SGA will probably be consistent between various monitors and sensors.
Our findings imply that clinicians and researchers, when using splanchnic NIRS measurements, have to account for PNA, GA, and SGA status in order to correctly interpret rsSO2 values. Furthermore, this study shows that SGA infants and infants with lower GA have lower rsSO2 confirming a higher risk of hypoxia of the intestines. In conclusion, these reference values may facilitate identification of infants with aberrant rsSO2 values, advance the use of rsSO2 monitoring in clinical care, and increase the clinical implication of research results. A next step will be to investigate whether infants developing intestinal diseases such as necrotizing enterocolitis indeed have aberrant rsSO2 values compared to preterm infants during the first week after birth.
References
Patel, R. M. et al. Causes and timing of death in extremely premature infants from 2000 through 2011. N. Engl. J. Med. 372, 331–340 (2015).
Ancel, P. Y. et al. Survival and morbidity of preterm children born at 22 through 34 weeks’ gestation in France in 2011 results of the EPIPAGE-2 cohort study. JAMA Pediatr. 169, 230–238 (2015).
Martini, S. & Corvaglia, L. Splanchnic NIRS monitoring in neonatal care: rationale, current applications and future perspectives. J. Perinatol. 38, 431–443 (2018).
Watzman, H. M. et al. Arterial and venous contributions to near-infrared cerebral oximetry. Anesthesiology 93, 947–953 (2000).
Schat, T. E. et al. Near-infrared spectroscopy to predict the course of necrotizing enterocolitis. PLoS ONE 11, e0154710 (2016).
Schat, T. E. et al. Early cerebral and intestinal oxygenation in the risk assessment of necrotizing enterocolitis in preterm infants. Early Hum. Dev. 131, 75–80 (2019).
Patel, A. K. et al. Abdominal near-infrared spectroscopy measurements are lower in preterm infants at risk for necrotizing enterocolitis. Pediatr. Crit. Care Med. 15, 735–741 (2014).
Corvaglia, L. et al. Splanchnic oxygenation at first enteral feeding in preterm infants: correlation with feeding intolerance. J. Pediatr. Gastroenterol. Nutr. 64, 550–554 (2017).
Mintzer, J. P., Parvez, B., Chelala, M., Alpan, G. & Lagamma, E. F. Monitoring regional tissue oxygen extraction in neonates <1250 g helps identify transfusion thresholds independent of hematocrit. J. Neonatal Perinat. Med. 7, 89–100 (2014).
Banerjee, J., Leung, T. S. & Aladangady, N. Blood transfusion in preterm infants improves intestinal tissue oxygenation without alteration in blood flow. Vox Sang. 111, 399–408 (2016).
Braski, K. et al. Splanchnic-cerebral oxygenation ratio decreases during enteral feedings in anemic preterm infants: observations under near-infrared spectroscopy. Neonatology 113, 75–80 (2018).
Grometto, A., Pizzo, B., Strozzi, M. C., Gazzolo, F. & Gazzolo, D. Near-infrared spectroscopy is a promising noninvasive technique for monitoring the effects of feeding regimens on the cerebral and splanchnic regions. Acta Paediatr. Int. J. Paediatr. 107, 234–239 (2018).
Nowicki, P. T. Ischemia and necrotizing enterocolitis: where, when, and how. Semin. Pediatr. Surg. 14, 152–158 (2005).
Bhatt, M., Petrova, A. & Mehta, R. Does treatment of patent ductus arteriosus with cyclooxygenase inhibitors affect neonatal regional tissue oxygenation? Pediatr. Cardiol. 33, 1307–1314 (2012).
Seager, E., Longley, C., Aladangady, N. & Banerjee, J. Measurement of gut oxygenation in the neonatal population using near-infrared spectroscopy: a clinical tool? Arch. Dis. Child. Fetal Neonatal Ed. 105, 76–86 (2020).
McNeill, S., Gatenby, J. C., McElroy, S. & Engelhardt, B. Normal cerebral, renal and abdominal regional oxygen saturations using near-infrared spectroscopy in preterm infants. J. Perinatol. 31, 51–57 (2011).
Cortez, J. et al. Noninvasive evaluation of splanchnic tissue oxygenation using near-infrared spectroscopy in preterm neonates. J. Matern. Neonatal Med. 24, 574–582 (2011).
Bell, M. J. et al. Neonatal necrotizing enterocolitis. Therapeutic decisions based upon clinical staging. Ann. Surg. 187, 1–7 (1978).
Palleri, E., Wackernagel, D., Wester, T. & Bartocci, M. Low splanchnic oxygenation and risk for necrotizing enterocolitis in extremely preterm newborns. J. Pediatr. Gastroenterol. Nutr. 71, 401–406 (2020).
van der Laan, M. E. et al. Multisite tissue oxygenation monitoring indicates organ-specific flow distribution and oxygen delivery related to low cardiac output in preterm infants with clinical sepsis. Pediatr. Crit. Care Med. 17, 764–771 (2016).
Hoftiezer, L. et al. From population reference to national standard: new and improved birthweight charts. Am. J. Obstet. Gynecol. 220, 383.e1–383.e17 (2019).
Niklasson, A. & Albertsson-Wikland, K. Continuous growth reference from 24th week of gestation to 24 months by gender. BMC Pediatr. 8, 8 (2008).
Richardson, D. K., Corcoran, J. D., Escobar, G. J. & Lee, S. K. SNAP-II and SNAPPE-II: simplified newborn illness severity and mortality risk scores. J. Pediatr. 138, 92–100 (2001).
Papile, L. A., Burstein, J., Burstein, R. & Koffler, H. Incidence and evolution of subependymal and intraventricular hemorrhage: a study of infants with birth weights less than 1,500 gm. J. Pediatr. 92, 529–534 (1978).
Bhaskaran, K. & Smeeth, L. What is the difference between missing completely at random and missing at random. Int. J. Epidemiol. 43, 1336–1339 (2014).
Kleinke, K. Multiple imputation by predictive mean matching when sample size is small. Methodology 14, 3–15 (2018).
Hoyle, R. H. Handbook of Structural Equation Modeling (Guilford Press, New York, 2012).
Diallo, T. M. O., Morin, A. J. S. & Parker, P. D. Statistical power of latent growth curve models to detect quadratic growth. Behav. Res. 46, 357–371 (2014).
Clyman, R. I. Mechanisms regulating the ductus arteriosus. Biol. Neonate 89, 330–335 (2006).
Ledo, A., Aguar, M., Núñez-Ramiro, A., Saénz, P. & Vento, M. Abdominal near-infrared spectroscopy detects low mesenteric perfusion early in preterm infants with hemodynamic significant ductus arteriosus. Neonatology 112, 238–245 (2017).
Reller, M. D., Rice, M. J. & McDonald, R. W. Review of studies evaluating ductal patency in the premature infant. J. Pediatr. 122, S59–S62 (1993).
Freeman-Ladd, M., Cohen, J. B., Carver, J. D. & Huhta, J. C. The hemodynamic effects of neonatal patent ductus arteriosus shunting on superior mesenteric artery blood flow. J. Perinatol. 25, 459–462 (2005).
Koch, J. et al. Prevalence of spontaneous closure of the ductus arteriosus in neonates at a birth weight of 1000 grams or less. Pediatrics 117, 1113–1121 (2016).
Tanis, J. C. et al. Correlation between Doppler flow patterns in growth-restricted fetuses and neonatal circulation. Ultrasound Obstet. Gynecol. 48, 210–216 (2016).
Ley, D. & Maršál, K. Doppler velocimetry in cerebral vessels of small-for-gestational age infants. Early Hum. Dev. 31, 171–180 (1992).
Thompson, A., Silva, C. T., Gork, A. S., Wang, D. & Ehrenkranz, R. A. Intestinal blood flow by doppler ultrasound: the impact of gestational age and time from first enteral feeding in preterm neonates. Am. J. Perinatol. 31, 261–268 (2014).
Papacci, P. et al. Neonatal colour Doppler ultrasound study: normal values of abdominal blood flow velocities in the neonate during the first month of life. Pediatr. Radiol. 39, 328–335 (2009).
Dix, L. M. L., Van Bel, F., Baerts, W. & Lemmers, P. M. A. Comparing near-infrared spectroscopy devices and their sensors for monitoring regional cerebral oxygen saturation in the neonate. Pediatr. Res. 74, 557–563 (2013).
Acknowledgements
We thank the nurses, the medical staff of the neonatology department of the Beatrix Children’s Hospital in Groningen, and the medical students who participate in the NIRS team for their contribution in collecting data of this study. This study was part of the research program of the Graduate School of Medical Sciences, Research Institutes SHARE and BCN, University of Groningen. M.v.d.H. and B.M.D. were financially supported by a grant from the Junior Scientific Master Class of the University of Groningen.
Author information
Authors and Affiliations
Contributions
M.v.d.H., B.M.D., and E.M.W.K. were involved in the design and execution of the study. R.E.S. contributed to the analysis and interpretation of the data. All other authors were involved in the final consensus process of the protocol and revised the manuscript critically for important intellectual content. M.v.d.H. and B.M.D. drafted the manuscript and all other authors read, edited, and approved the final manuscript for publication.
Corresponding author
Ethics declarations
Competing interests
The authors declare no competing interests.
Consent statement
Due to the retrospective character of the study, no informed consent from the participant’s legal guardian was required. Nevertheless, none of the participant’s legal guardians objected to participate during admission when they were offered this option.
Additional information
Publisher’s note Springer Nature remains neutral with regard to jurisdictional claims in published maps and institutional affiliations.
Supplementary information
Rights and permissions
About this article
Cite this article
van der Heide, M., Dotinga, B.M., Stewart, R.E. et al. Regional splanchnic oxygen saturation for preterm infants in the first week after birth: reference values. Pediatr Res 90, 882–887 (2021). https://doi.org/10.1038/s41390-020-01323-3
Received:
Revised:
Accepted:
Published:
Issue Date:
DOI: https://doi.org/10.1038/s41390-020-01323-3
- Springer Nature America, Inc.
This article is cited by
-
The effect of drip versus intermittent feeding on splanchnic oxygenation in preterm infants with intrauterine growth restriction: a prospective randomized trial
European Journal of Pediatrics (2023)
-
Early brain and abdominal oxygenation in extremely low birth weight infants
Pediatric Research (2022)
-
Normal regional tissue oxygen saturation in neonates: a systematic qualitative review
Pediatric Research (2021)