Abstract
Agroforestry plays a pivotal role in mitigating greenhouse gas (GHG) emissions and addressing the challenges posed by climate change. While carbon sequestration efforts have primarily centred on forests, it is imperative to acknowledge the contribution that non-forest ecosystems, such as agroforestry, can offer. This study investigated the influence of agroforestry systems on the variation of biomass carbon stocks in the Sahelo-Sudanian and Sudanian regions of the Senegalese Groundnut basin. Three primary agroforestry systems were studied: silvopastoral, agrisilvicultural, and agrosilvopastoral. Forty-six (46) 100 m × 100 m plots were sampled, within which 1 × 1 m2 subplots were used to sample understory biomass across three agroforestry systems in the two climatic zones. Analysis of variance was performed to assess the influence of agroforestry systems and climatic zones on biomass carbon stocks. The findings showed that in the Sahelo-Sudanian region, the agrisilvicultural system exhibited the highest AGC + BGC stocks, averaging 43.42 ± 21.61 tCha−1. In contrast, the silvopastoral system showed significantly higher AGC + BGC stocks, which amounted to 36.33 ± 12.27 tCha−1 in the Sudanian region. On the other hand, understory carbon stocks were significantly higher (p < 0.01) in the agrisilvicultural and agrosilvopastoral systems than in the silvopastoral system in both climatic zones. Agroforestry systems had a significant effect on AGC + BGC stocks within climatic zones. Nevertheless, the effect was less pronounced when comparing across climatic regions. These results underscore the importance of specific land management practices interacting with local climatic conditions to influence AGC + BGC stocks. Therefore, policy makers should carefully consider the interaction of these factors when implementing carbon management practices and planning mitigation strategies in West Africa.
Similar content being viewed by others
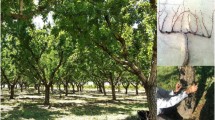
Avoid common mistakes on your manuscript.
1 Introduction
The expansion of agriculture has been recognised as a major driver of deforestation and forest degradation, leading to the conversion of forests to agricultural and pasture lands and resulting in significant losses of soil organic carbon stock [1]. The Agriculture, Forestry, and Other Land Use (AFOLU) sector accounted for an average of 11.9 ± 4.4 GtCO2-eq year−1, or approximately 21% of global greenhouse gas emissions, between 2010 and 2019 [2]. The primary contributors to global climate change are greenhouse gases (GHG) like carbon dioxide, nitrogen dioxide, and sulfur dioxide [3, 4], with carbon dioxide being the most prevalent GHG [4]. As a result, there is a growing emphasis on finding mitigation strategies to reduce carbon dioxide emissions [4, 5].
Agroforestry, a practice that involves integrating woody plants with crops and animals within a single land management unit, either in spatial or temporal sequence [6], can play a crucial role in addressing these challenges. It has emerged as a globally recognised approach to achieving sustainable land use objectives. This implies the simultaneous management of agriculture and natural resources to achieve a range of goals, including food and fibre production, biodiversity conservation, ecosystem service provision, and the enhancement of human well-being [7]. Agroforestry promotes carbon sequestration, thereby significantly influencing carbon dynamics and mitigating GHG emissions [8,9,10,11]. Its ability to enhance resilience to climate change and mitigate its impacts by capturing carbon from the atmosphere and storing it in biomass or soil reservoirs [12] positions agroforestry as a promising land use strategy for the future. Carbon storage in agroforestry systems occurs in both aboveground and belowground pools [13]. The aboveground pool includes living organisms such as trees, shrubs, grasses and herbs, while the belowground pool comprises soil, roots, and rhizomes [14]. Although estimating belowground biomass introduces greater uncertainty than aboveground biomass, it is an important reservoir of carbon in various vegetation types [15]. Agroforestry systems store more carbon in their aboveground and belowground biomass than conventional cropping and pasture systems [1]. Since the adoption of the Kyoto Protocol in 2005, agroforestry has been recognised as an afforestation activity contributing to biodiversity conservation and carbon dioxide (CO2) storage [1].
Agroforestry systems in Africa, especially in Senegal, come in various forms, such as fallow, parkland, rangeland, multi-storied home garden and woodlots [16, 17]. These different systems can be broadly classified into three main land use types based on their major components and interactions: silvopastoral, agrisilvicultural and agrosilvopastoral systems [18]. An agrisilvicultural system combines crops and trees, while the agrosilvopastoral system integrates crops, animals, and trees. On the other hand, the silvopastoral system includes pastures, animals, and trees [18]. The Senegalese Groundnut basin encompasses the three primary agroforestry systems. These systems rely on different land management techniques that affect their diversity, structure, and carbon storage potential. However, despite significant advances in agroforestry research, our understanding of how both agroforestry systems and climate affect carbon stocks is limited. Furthermore, these systems are yet to be thoroughly investigated to evaluate the effects of their management practices on carbon storage.
This study provides valuable insights from semi-arid regions, which have often witnessed underestimations of carbon stocks, as shown by recent research [19, 20]. It sheds light on the understudied effect of agroforestry systems and climate on carbon stocks variation within the Senegalese Groundnut basin. The study aimed to assess the biomass carbon stocks in both aboveground and belowground components within the agrisilvicultural, agrosilvopastoral, and silvopastoral systems, while investigating their relationships with species diversity and dendrometric parameters. Furthermore, the research highlights the contribution of understory biomass to the overall carbon stock potential and underscores the pivotal role of dominant species in driving variation in biomass carbon stocks across systems. These findings have implications for understanding carbon dynamics in agroforestry systems and contribute to the growing importance of these systems in mitigating climate change and promoting sustainable land management practices.
2 Material and methods
2.1 Presentation of the study area
The research was carried out in the Senegalese Groundnut basin. It focused on the sites of Keur Macodou, Newrane and Ndangalma in the Sahelo-Sudanian region and Lamarame, Missira, Mbotil-Done and Tobene representing the Sudanian zone (Supplementary Information, Table 1). The Senegalese Groundnut basin is the largest agricultural landscape in the country [21]. It accounted for 65% of the country’s rural population and covered a significant part of Senegal’s land mass (33% of the surface area) in the early 1980s [22]. Between 2009 and 2018, the agricultural area increased by 14.53%, with a dynamic index of 1.45 [23]. The Groundnut basin plays an important role in the agricultural sector, contributing to 80% of the country’s exportable groundnut production and 70% of cereal production [23]. Throughout the Groundnut basin, the dominant cropping systems revolve around cereals- leguminous rotations [24], focusing on millet and groundnut production [25]. The Sahelo-Sudanian region of the Groundnut basin receives an annual mean rainfall of 400 to 500 mm, while the Sudanian region receives an average rainfall of 800 to 900 mm. Average temperatures range from 20 to 33 °C in the Sahelo-Sudanian region and from 22 to 36 °C in the Sudanian region. The Sahelo-Sudanian region is characterised by shrub savanna, where typical Sahelian species, such as Guiera senegalensis J.F.Gmel., Combretum glutinosum Perr. ex DC., Balanites aegyptiaca (L.) Delile, Bauhinia rufescens Lam., and Faidherbia albida (Delile) A.Chev are found. On the other hand, the vegetation of the Sudanian region is dominated by Sudanian species such as Cordyla pinnata (A.Rich.) Milne-Redh., Pterocarpus erinaceus Poir., Daniellia oliveri Hutch. & Dalziel, and Parkia biglobosa (Jacq.) R.Br. ex G.Don. Both areas have a unimodal rainfall regime with a rainy season from June to September, the main growing season (Fig. 1). The area experienced a prolonged severe drought between 1968 and 1974 [21], which led to a reduction in plant cover and soil erosion [26, 27].
2.2 Sampling design and data collection
2.2.1 Field inventory
Data collection was based on stratification [28] within the Sahelo-Sudanian and Sudanian areas. The main strata corresponded to the agroforestry systems: agrosilvopastoral, agrisilvicultural and silvopastoral (Table 1). Within each climatic zone, sites were selected on the basis of biophysical aspects, particularly the type of agroforestry practiced. Key informants were involved in the inventory process to facilitate data collection from farmers. Forty-six (46) 100 × 100m2 plots were established in the Sahelo-Sudanian (23) and Sudanian (23) zones. We sampled 10 plots in the agrisilvicultural system and 10 in the agrosilvopastoral system within each zone. However, six plots were sampled in the silvopastoral systems due to their conversion to cropland. In the agrisilvicultural and agrosilvopastoral systems, the plots were randomly distributed within the main crops, such as millet and groundnut. Conversely, due to the absence of crops, no distinct strata were considered in the silvopastoral systems. A tape measure and Blume-Leiss were used to measure both the diameter at breast height (DBH) and height. The inventory included individuals ≥ 5 cm in diameter, contributing significantly to overall biomass in a typical savanna environment [29]. For multi-stemmed individuals with n main stems (i = 1…, n), the quadratic sum of the diameters (dsi) was applied to ensure that values were neither overestimated nor underestimated [29,30,31,32].
2.2.2 Understory biomass measurement
The composition of the understory biomass differs between agroforestry systems. In the agrisilvicultural and agrosilvopastoral systems, the understory biomass consists mainly of crops and weeds, while in the silvopastoral system, it consists mainly of herbaceous species. Depending on the system, herbs or crops were sampled within the 100 m × 100 m sampling unit used for the woody vegetation inventory. Within each sampling unit, five 1 × 1m2 subplots [33] were established at the centroid and the four corners of each plot between October and November (Supplementary Information, Figs. 1, 2). All aboveground herbaceous and crop biomass within these subplots was harvested [33]. In the agrisilvicultural and agrosilvopastoral systems, the biomass sampling was stratified by crop type, such as millet and groundnut. The harvested samples from each 1 × 1 m2 subplot were mixed and weighed in situ to determine their fresh weight [34]. These fresh samples were then transported to the laboratory, where they underwent oven-drying at 70 °C for 24 h to determine their dry weight and estimate their biomass.
2.3 Data treatment and analysis
2.3.1 Diversity and dendrometric parameters measurement
Various ecological indices and parameters were considered to calculate species diversity and dendrometric parameters within agroforestry systems, as outlined in Table 2.
2.3.2 Estimation of biomass
A non-destructive approach was carried out to estimate biomass within each agroforestry system using allometric equations [8, 39,40,41]. Different allometric equations were used to estimate aboveground and belowground biomass, as shown in Table 3. Palm tree biomass was estimated using the equation of [42], while other woody perennials were estimated using the generic model [43] and the Sudanian-based model [29]. Belowground biomass was estimated using the equation proposed by [44]. The biomass of herbs, crops, shrubs, and trees was summed to determine the total biomass. First, the biomass of each component was converted to the same unit (tha−1). Then, the biomass was estimated for each plot and averaged over each agroforestry system.
2.3.3 Estimation of carbon stocks
Carbon stocks were determined by multiplying the biomass of plants such as trees, shrubs, herbs, or crops by a commonly used conversion factor of 0.50, representing the biomass’s average carbon content [45,46,47,48].
3 Statistical analysis
Statistical analysis was conducted using R software, version 4.2.2 (R Core Team, 2022). The Shapiro–Wilk test was performed to ensure normality of the data. For normally distributed data, analysis of variance (ANOVA) was applied, and significant mean differences were determined using Tukey’s test (α ≤ 0.05). In cases where normality assumptions were not met, the Kruskal-Walli’s test was utilized. Principal component analysis (PCA) was used to determine the relationship between carbon stocks, species diversity and relative abundance. Data accuracy was assessed through the Kaiser–Meyer–Olkin (KMO) test (threshold > 0.5), and correlation between variables was evaluated with Bartlett’s test (significant p < 0.001) prior to PCA [49]. In addition, pairwise product-moment correlation (r) was performed between AGC + BGC stocks, species diversity, and abundance. The 'BiodiversityR' package in R was used to compute diversity indices [28], while PCA was performed using the 'FactoMineR’ package in R [50]. A Generalised Linear Model (GLM) and two-way ANOVA were used to evaluate the effects of agroforestry systems and climate on AGC + BGC stocks and their interactions. Furthermore, a linear regression model assessed the correlation between AGC + BGC stocks and dendrometric parameters.
4 Results
4.1 Species diversity and abundance
The study revealed differences in species richness across climatic zones. Specifically, the Sahelo-Sudanian region exhibited 27 species, while the Sudanian region had a higher species number (36). Within both climatic zones, the agrosilvopastoral systems included 33 species, the agrisilvicultural systems comprised 36 species and the silvopastoral systems recorded 16 species (Supplementary Information, Table 2). In the Sahelo-Sudanian zone, both agrisilvicultural and agrosilvopastoral systems exhibited significantly higher species richness and diversity compared to the silvopastoral systems, with statistical significance at P ≤ 0.05, as depicted in Fig. 2A. However, no significant contrasts (P > 0.05) were observed in terms of species richness, diversity and evenness among the systems in the Sudanian zone (Fig. 2B).
Regarding relative abundance, Table 4 shows that the silvopastoral systems are more favourable in supporting a higher species relative abundance in the studied areas. In both climatic zones, these systems displayed a significantly higher species relative abundance (530 ± 232.42; 654.67 ± 96, respectively) than the agrisilvicultural (146.8 ± 106; 229.1 ± 191, respectively) and agrosilvopastoral (192 ± 89.17; 127.1 ± 114, respectively) systems.
4.2 Variation of dendrometric parameters within agroforestry systems and climatic zones
Table 5 shows significant variations in dendrometric parameters between the agroforestry systems. In the Sahelo-Sudanian area, the agrisilvicultural system had the highest mean diameter (73.38 ± 1.27 cm), followed by the agrosilvopastoral system (49.48 ± 5.79 cm) and the silvopastoral system (30.46 ± 1.17 cm). The basal area also showed significant differences, ranging from 6.26 ± 3.27 m2ha−1 in the agrisilvicultural system to 2.7 ± 1 m2ha−1 in the silvopastoral system. The mean height varied significantly among the systems, with the agrisilvicultural system having the highest value (18.68 ± 1.28 m) and the silvopastoral system the lowest (11.19 ± 0.24 m). However, the tree density was much higher in the silvopastoral system (32.33 ± 8.32 trees. ha−1) than in the agrisilvicultural (14.9 ± 4.77 trees. ha−1) and agrosilvopastoral (17.9 ± 3.72 trees. ha−1) systems. On the other hand, the agrisilvicultural system exhibited the highest canopy cover (24.64 ± 7.31%) than the silvopastoral (17.15 ± 7.39%) and agrosilvopastoral (15.75 ± 3.7%) systems. In the Sudanian area, the canopy cover was much higher in the silvopastoral system (32.63 ± 2.37%) than in the agrosilvopastoral system (13.18 ± 4.8%) and the agrisilvicultural system (8.6 ± 3.19%). The highest tree density was also observed in the silvopastoral system (40.66 ± 14.15 trees. ha−1), while the lowest was found in the agrisilvicultural system (4.7 ± 1.49 trees. ha−1). In contrast, the mean height and diameter were significantly higher in the agrosilvopastoral (22.75 ± 3.5 m and 70.69 ± 14.51 cm, respectively) and agrisilvicultural (18.79 ± 2.39 m and 70.58 ± 10.19 cm, respectively) systems than in the silvopastoral system (15.23 ± 2.99 m and 37.63 ± 6.55 cm, respectively). Furthermore, the basal area ranged from 4.03 ± 0.65 m2 ha−1 in the silvopastoral system to 2 ± 0.69 m2ha−1 in the agrisilvicultural system, with a significant difference (p < 0.01).
4.3 Aboveground and belowground carbon stocks within agroforestry systems and climatic zones
The study found significant variation in mean aboveground and belowground biomass carbon (AGC + BGC) stocks among the agroforestry systems, with different patterns observed in both climatic zones, as illustrated in Fig. 3A, B. In the Sahelo-Sudanian area (Fig. 3A), the agrisilvicultural system exhibited significantly higher AGC + BGC stocks (43.42 ± 21.61 tCha−1) than the agrosilvopastoral (21.4 ± 7.22 tCha−1) and the silvopastoral (10.24 ± 3.85 tCha−1) systems. Conversely, in the Sudanian area (Fig. 3B), the mean AGC + BGC stocks were significantly higher in the silvopastoral system (36.33 ± 12.27 tCha−1), with the agrisilvicultural system recording the lowest amount of 14.69 ± 6.4 tCha−1. In the Sahelo-Sudanian region, the three agroforestry systems stored an average of 29.52 ± 19 tCha−1, while in the Sudanian region, they stored 21.43 ± 11.86 tCha−1.
Further analysis using GLM and the two-way ANOVA confirmed the significant effect of agroforestry systems on AGC + BGC stocks within climatic zones (Tables 6, 7). However, when considering both climatic zones, no significant variation in AGC + BGC stocks was observed between agroforestry systems (Table 7). The findings emphasise that AGC + BGC stocks in agroforestry systems depend on climatic zones. Therefore, it is important to account for the interaction between agroforestry systems and climatic zones (P < 0.001) while devising carbon management strategies.
4.4 Variation in AGC + BGC stocks of dominant species within agroforestry systems and climatic zones
Figure 4 shows that in the Sahelo-Sudanian zone, the AGC + BGC stocks of the dominant tree species were significantly influenced by agroforestry systems. Faidherbia albida stored significantly more carbon in the agrisilvicultural system (39.18 ± 19.75 tCha−1) than in the agrosilvopastoral system (19.04 ± 6.97 tCha−1). In contrast, Balanites aegyptiaca stored significantly more carbon in the silvopastoral system (9.89 ± 3.58 tCha−1) than in the agrisilvicultural (1.73 ± 1.01 tCha−1) and agrosilvopastoral (2.26 ± 2.46 tCha−1) systems. A similar pattern was observed in the Sudanian area, where Balanites aegyptiaca stored significantly more carbon in the silvopastoral system (7.76 ± 4.95 tCha−1) than in the agrosilvopastoral system (0.05 ± 0.07 tCha−1). On the other hand, Faidherbia albida showed significantly higher carbon stocks in the agrosilvopastoral (19.46 ± 10.64 tCha−1) and silvopastoral (16.04 ± 5.89 tCha−1) systems than in the agrisilvicultural system (2.65 ± 2.16 tCha−1). Cordyla pinnata showed a higher carbon storage in the agrisilvicultural system (12.06 ± 5.13 tCha−1) than in the agrosilvopastoral system (5.5 ± 4.89 tCha−1). Individual species size and population density are important factors in the variation of carbon stocks. The mean diameter of Faidherbia albida was 64.09 ± 9.19 cm, while Cordyla pinnata and Balanites aegyptica had a mean diameter of 66.12 ± 8.17 and 28.51 ± 11.54 cm, respectively. Thus, the three species have distinct carbon storage potentials. Furthermore, the density of Balanites aegyptiaca varied considerably, with mean values ranging from 3.47 ± 1.87 trees. ha−1 in the farm-based systems (agrisilvicultural and agrosilvopastoral) to 31.66 ± 7.76 trees. ha−1 in the forest-based system (silvopastoral) within the Sahelo-Sudanian zone. In the Sudanian zone, the density ranged from 1.2 ± 0.44 (agrosilvopastoral system) to 28.66 ± 18.44 (silvopastoral system) trees. ha−1. The density of Faidherbia albida in the Sahelo-Sudanian zone ranged from 12.8 ± 5.11 (agrisilvicultural system) to 13 ± 3.01 (agrosilvopastoral system) trees. ha−1. In the Sudanian zone, it varied from 1 ± 1.02 (agrisilvicultural), 5.4 ± 3.2 (agrosilvopastoral) to 6 ± 1 (silvopastoral system) trees. ha−1. The density of Cordyla pinnata ranged from 3.55 ± 1.54 trees. ha−1 in the agrisilvicultural system to 2.17 ± 1.47 trees. ha−1 in the agrosilvopastoral system in the Sudanian zone. Across climatic zones, Faidherbia albida had significantly higher AGC + BGC stocks in the Sahelo-Sudanian zone (29.12 ± 17.74 tCha−1) than in the Sudanian zone (14.22 ± 11.02 tCha−1). For Balanites aegyptiaca, there was no significant variation, with mean AGC + BGC stocks of 3.27 ± 3.63 tCha−1 in the Sahelo-Sudanian zone and 2.94 ± 4.79 tCha−1 in the Sudanian zone. Cordyla pinnata stored an average of 9.88 ± 5.85 tCha−1 in the Sudanian area where it occurs.
4.5 Understory biomass carbon stocks within agroforestry systems and climatic zones
Understory biomass also contributes to the overall carbon storage potential of agroforestry systems. In the Sahelo-Sudanian area (Fig. 5A), the agrisilvicultural and agrosilvopastoral systems displayed a significantly higher understory carbon stocks (4.54 ± 3.5 tCha−1and 3.81 ± 2.2 tCha−1, respectively) compared to the silvopastoral system (2 ± 1.45 tCha−1). Conversely, in the Sudanian area (Fig. 5B), the agrosilvopastoral system stood out with a significantly higher understory carbon stock (5.52 ± 2.50 tCha−1) than the agrisilvicultural (3.44 ± 1.43 tCha−1) and silvopastoral (2.38 ± 1.35 tCha−1) systems. Furthermore, millet contributed substantially to the total carbon stock of the understory biomass, accounting for up to 82.96% and 75.11% in the agrisilvicultural and agrosilvopastoral systems, respectively, in the Sahelo-Sudanian area. In the Sudanian area, the contribution of millet ranged from 73.55% (agrosilvopastoral system) to 51% (agrisilvicultural system).
Adding up the AGC + BGC and understory carbon stocks (Fig. 5A, B), the mean biomass carbon stock potential of the agroforestry systems in the Sahelo-Sudanian area was 28.47 tCha−1, distributed as 47.96 tCha−1 in the agrisilvicultural system, 25.21 tCha−1 in the agrosilvopastoral system, and 12.24 tCha−1 in the silvopastoral system. In the Sudanian area, the agroforestry systems studied had an average biomass carbon stock of 28.96 tCha−1, with 18.96 tCha−1 in the agrisilvicultural system, 29.23 tCha−1 in the agrosilvopastoral system, and 38.71 tCha−1 in the silvopastoral system.
4.6 Relationship between AGC + BGC stocks and diversity parameters
Figure 6 depicts the relationship between species richness, diversity, relative abundance and AGC + BGC stocks within systems. In the Sahelo-Sudanian area (Fig. 6A), the two axes (PC#1 and PC#2) together explained 85.3% of the total variance in the dataset. The results revealed that species richness, diversity indices (Shannon, N1, N2 and evenness) and AGC + BGC stocks were positively correlated with PC#1. Conversely, these variables showed a negative relationship with relative abundance.
In the Sudanian area (Fig. 6B), PC#1 and PC#2 accounted for 82.5% of the total variance between systems. The findings revealed that AGC + BGC stocks and relative abundance were positively correlated. However, these variables were negatively correlated with the diversity indices.
Furthermore, the Pearson correlation coefficient (r) revealed a significant and positive correlation between species richness and AGC + BGC stocks (r = 0.47; p < 0.01) in the Sahelo-Sudanian area (Table 8). A positive relationship was also observed between N1 and AGC + BGC stocks (r = 0.41; p ≤0.05). However, there was no significant correlation between AGC + BGC stocks and Shannon, evenness and N2. Surprisingly, AGC + BGC stocks and relative abundance were strongly and negatively correlated (r = −0.48; p < 0.01).
In contrast, in the Sudanian area (Table 8), no significant correlation was observed between AGC + BGC stocks and species richness or between Shannon and AGC + BGC stocks. Similarly, there was a weak relationship between AGC + BGC stocks and species evenness, N1 and N2. However, the findings showed a strong and positive correlation between relative abundance and AGC + BGC stocks (r = 0.52 ; p < 0.01). These results shed light on the AGC + BGC stocks, which may depend on woody species richness in the Sahelo-Sudanian area. In contrast, relative abundance may be more influential in controlling AGC + BGC stocks in the Sudanian area.
4.7 Relationship between AGC + BGC stocks and dendrometric parameters
Figures 7 and 8 show a strong linear correlation between dendrometric parameters and AGC + BGC stocks, with a significant positive effect (P < 0.001) in both climatic zones. Height, diameter, canopy cover, and basal area contributed significantly to AGC + BGC stocks. In the Sahelo-Sudanian zone, height explained 43% (Fig. 7B), canopy cover 54% (Fig. 7A), diameter 77% (Fig. 7C), and basal area 95% (Fig. 7D) of the variation in AGC + BGC stocks. Similarly, in the Sudanian region, height, canopy cover, DBH, and basal area accounted for 61%, 62%, 80% and 96% of the variation in AGC + BGC stocks, respectively (Fig. 8B, A, C, D). These results highlight the importance of dendrometric parameters in the variation of AGC + BGC stocks within agroforestry systems.
5 Discussion
5.1 Slight grazing enhances species richness and diversity
The total number of species observed in the two climatic regions was higher in agrisilvicultural systems compared to agrosilvopastoral and silvopastoral systems. This difference may be related to factors such as grazing intensity. Our study highlighted that high grazing intensity systems (agrosilvopastoral and silvopastoral) had lower species richness than light grazing intensity systems (agrisilvicultural). Indeed, the silvopastoral systems investigated serve as permanent village pastures shared by several villages. Similarly, agrosilvopastoral systems are used by transhumant pastoralists from northern Senegal during the dry season when fodder becomes scarce. Intensive grazing in these systems has affected plant species richness. The results are consistent with similar studies conducted in pasture and shrub lands [51, 52]. Furthermore, at the scale of system types, the agrisilvicultural and agrosilvopastoral systems, which include activities such as cropping, thinning, and weeding, showed greater species diversity compared to the silvopastoral system in the Sahelo-Sudanian zone. Indeed, thinning activities contribute to increased species diversity by modifying the light environment and promoting the growth and survival of understory vegetation [53]. In addition, in agrisilvicultural and agrosilvopastoral systems, farmers select and manage species diversity with more protection than in communal areas such as silvopastoral systems. It is worth noting that the silvopastoral system in the Sudanian area is managed through a well-organised participatory community approach, distinguishing it from the silvopastoral system in the Sahelo-Sudanian area. The differences in management practices could account for the variation in species richness and diversity levels observed in the two silvopastoral systems. This distinction may contribute to the similarity observed between the silvopastoral, agrisilvicultural, and agrosilvopastoral systems in the Sudanian zone.
5.2 Cropping and related activities influence the dendrometric parameters of agroforestry systems
Cropping and related activities are important factors inducing variation in the dendrometric parameters of agroforestry systems. In the Sahelo-Sudanian zone, Faidherbia albida was the most dominant species in the agrosilvopastoral and agrisilvicultural systems, accounting for 77.57% and 84.37% of the species, respectively (Supplementary Information, Table 2). In the Sudanian zone, Cordyla pinnata was the most common species in the agrisilvicultural system, accounting for 62.96% of the species, while Faidherbia albida dominated the agrosilvopastoral system, accounting for 73.92% of the species. Both species in these systems showed individuals with large heights and diameters, but with lower densities. On the other hand, the silvopastoral systems, which were dominated by Balanites aegyptiaca in the Sahelo-Sudanian zone (85.97%) and by both Faidherbia albida (45.65%) and Balanites aegyptiaca (34.41%) in the Sudanian zone, were characterised by individuals with small to medium diameters and heights, but with higher densities. Thus, our results suggest that tree density affects height and diameter, with higher density being associated with lower height and diameter and vice versa. These findings align with the research of [54], who demonstrated that changes in tree density significantly affected diameter in Leucaena-based agroforestry systems. Furthermore, management practices such as harvesting, thinning, and weeding did not only affect species richness. They also appeared to influence individual density, with agrisilvicultural and agrosilvopastoral systems having lower tree densities than silvopastoral systems. Similar results were reported by [55], who suggested that the tree density in the Sudanian savanna may be influenced by logging, annual shrub removal, and fire, which promote tree growth by reducing competition [56].
5.3 The different factors contributing to biomass carbon stocks
Our research highlights the significant influence of agroforestry systems on the variation of biomass carbon stocks within climatic zones. The AGC + BGC stored in agroforestry systems in the Senegalese Groundnut basin exceeded that reported for similar systems in India [57]. The authors found higher AGC + BGC stocks in agrosilvopastoral systems (73.4 MgCha−1) compared to agrisilvicultural (42.6 MgCha−1) and silvopastoral (42.7 MgCha−1) systems. However, our results contradict the opinion that the agrosilvopastoral system stores more carbon than the other two systems, indicating a higher carbon storage potential in the silvopastoral and agrisilvicultural systems in the Sudanian and Sahelo-Sudanian regions, respectively. This difference can be attributed to the pruning practices employed in the agrosilvopastoral systems, which affected the diameter growth of individuals [58].
The variation in mean AGC + BGC stocks was significantly influenced by the interaction between agroforestry systems and climatic zones, as reported by [59] in the forest ecosystem. Indeed, the intensive logging pressure experienced in the Sudanian region affected tree density (especially the agrisilvicultural system), which emerged as a critical factor influencing the variation of AGC + BGC stocks in the Sudanian area, as reported by [60] in homestead forests. In the Sahelo-Sudanian area, differences in tree diameter size and the dominance of specific species are likely to be key factors contributing to the variation in AGC + BGC stocks. Indeed, among the dominant species, Faidherbia albida showed the highest AGC + BGC storage potential, followed by Cordyla pinnata and Balanites aegyptiaca. This finding is consistent with the results reported by [17], who revealed that Faidherbia albida had the highest carbon storage potential among five woody species in the Niaye zone of Senegal. It is worth noting that Faidherbia albida also provides additional benefits, such as improving crop yield, which is attributed to its ability to enhance soil nitrogen content, improve water use efficiency, and mitigate heat stress [61]. Consequently, the silvopastoral system of the Sahelo-Sudanian zone, characterised by the dominance of Balanites aegyptiaca, resulted in the lowest AGC + BGC stocks, while that of the Sudanian zone, characterised by the dominance of Faidherbia albida and Balanites aegyptiaca had the highest AGC + BGC stocks. Although the agrisilvicultural and agrosilvopastoral systems were dominated by Faidherbia albida, the highest tree diameters were observed in the agrisilvicultural system, demonstrating the effect of diameter size on the carbon stock potential of agroforestry systems. Similar conclusions have been drawn by [60, 62]. Based on the results, agroforestry systems and management practices significantly affected the AGC + BGC stocks of the dominant species. The variation observed in the AGC + BGC stocks of Faidherbia albida in the Sahelo-Sudanian zone is due to pruning activities. However, in the Sudanian zone, it is linked to its very low density in the agrisilvicultural system, where Cordyla pinnata was dominant. Balanites aegyptiaca stored higher carbon in the silvopastoral system because it is less valued in agricultural lands due to its low soil enrichment capacity compared to Faidherbia albida and Cordyla pinnata. Furthermore, the understory biomass was crucial for the total carbon stocks. The selection of a 1 × 1 m2 sample size, as reported in previous studies [33, 34], captured significant variation in mean understory biomass carbon stocks between agroforestry systems. In both climatic zones, the carbon stored in understory biomass was significantly higher in the agrisilvicultural and agrosilvopastoral systems than in the silvopastoral systems. This disparity can be attributed to the high grazing pressure in the silvopastoral systems, which probably reduced the biomass of understory species and, consequently, their carbon storage potential [52]. In addition, the difference in understory carbon stocks between agrisilvicultural and agrosilvopastoral systems can be explained by differences in management practices such as planting density, effective crop management practices, fertilisation, pest and disease control, and the selection of specific crop varieties.
Moreover, the total biomass carbon stock (AGC + BGC + understory) of the agrisilvicultural, agrosilvopastoral, and silvopastoral systems in the Sahelo-Sudanian area and those in the Sudanian area exceeded the results reported by [63] for degraded savannas (9 tCha−1) in northern Senegal. However, it was lower than the carbon stock of the remaining forests (113 tCha−1) in the Senegal River valley. This difference may be due to the lower tree density in agroforestry systems compared to forests.
5.4 Relationship between AGC + BGC stocks and diversity
The results showed a significant and positive relationship between species richness and AGC + BGC stocks and no significant correlation between AGC + BGC stocks and Shannon or Evenness in the Sahelo-Sudanian area, as reported by [10, 56, 64,65,66]. In contrast, the relationship between AGC + BGC stocks and species richness was weak in the Sudanian region, as observed by [64, 66] in different agroforestry systems. However, relative abundance showed a positive and significant correlation with AGC + BGC stocks [66] in the Sudanian zone, while in the Sahelo-Sudanian area, the correlation was strong and negative. This can be attributed to the fact that the silvopastoral system, which recorded higher relative abundance, stored the lowest AGC + BGC stocks due to the dominance of Balanites aegyptiaca, which has a low carbon storage potential. This probably contributed to the observed discrepancy and negative correlation between relative abundance and AGC + BGC stocks. In contrast, in the Sudanian area, the silvopastoral system, with the highest relative abundance, recorded the highest carbon stocks, which may explain the positive correlation between relative abundance and AGC + BGC stocks.
5.5 Relationship between AGC + BGC stocks and dendrometric parameters
The results of this study underline a significant influence of dendrometric parameters on the variation of AGC + BGC stocks in both climatic zones of the Senegalese Groundnut basin. Previous studies [46, 67,68,69,70,71] have also reported that dendrometric parameters, especially DBH and basal area, are crucial for aboveground and belowground carbon stocks. While studies have mainly focused on the relationships between dendrometric parameters and carbon stocks in natural ecosystems, this study shows that these parameters are also strongly related to each other within agroforestry systems, despite the high human pressure. This finding shows that conserving and maintaining these dendrometric parameters can enhance biomass carbon sequestration. Thus, planting species with high growth rates, increasing density and adopting sustainable land management techniques, particularly reducing pruning, can significantly influence the AGC + BGC stocks in these systems. Furthermore, the correlations we found between dendrometric parameters and AGC + BGC stocks are consistent with basic ecological principles and the equations used for calculations. The insights from this research also contribute to our understanding of carbon dynamics in agroforestry systems and highlight the importance of considering dendrometric parameters, species richness, relative abundance, pruning and dominant species when assessing and managing carbon stocks in such environments.
6 Conclusion
This study showed a significant variation in biomass carbon stocks within agroforestry systems in each climatic zone. In the Sahelo-Sudanian region, the agrisilvicultural system had the highest average biomass carbon stocks, whereas in the Sudanian zone, the silvopastoral system showed higher carbon stocks. The main factors influencing the variability of AGC + BGC stocks within these agroforestry systems in both climatic zones were tree density, DBH, basal area, species richness, relative abundance and dominant species. Therefore, it is crucial to focus on improving species richness and tree density and minimising pruning and felling to maintain and enhance the carbon storage potential of agroforestry systems. In addition, Faidherbia albida, which has a higher carbon storage potential can be widely promoted to increase the biomass carbon stocks of agroforestry systems in Senegal. Furthermore, given the significant effect of agroforestry systems-climate interactions on carbon stocks, it is crucial to customise carbon management strategies to the specific characteristics of each climatic zone. Future research should also investigate the soil carbon stocks in these systems and the relationship between aboveground and belowground carbon. This will provide a better understanding of the carbon sequestration potential of agroforestry systems under different management practices.
Data availability
Data are available from the corresponding author upon reasonable request.
Code availability
Data are available from the corresponding author upon reasonable request.
References
Abbas F, Hammad HM, Fahad S, Cerdà A, Rizwan M, Farhad W, Bakhat HF. Agroforestry: a sustainable environmental practice for carbon sequestration under the climate change scenarios-a review. Environ Sci Pollut Res. 2017;24(12):11177–91. https://doi.org/10.1007/s11356-017-8687-0.
Nabuurs, G.J., R. Mrabet, A. Hatab, M. Bustamante, H. Clark, P. Havlík, J. House, C. Mbow, K.N. Ninan, A. Popp, S. Roe, B. Sohngen, S. Towprayoon. 2022. Agriculture Forestry and Other Land Uses (AFOLU). In: IPCC 2022 Climate Change 2022: Mitigation of PR Shukla J Skea R Slade A Al Khourdajie R Diemen van D McCollum M Pathak S Some P Vyas R Fradera M Belkacemi A Hasija G Lisboa S Luz J Malley (eds). Climate Change Contribution of Working Group III to the Sixth Assessment Report of the Intergovernmental Panel on Climate Change. Cambridge University Press. Cambridge
FAO (Food and Agriculture Organization of the United Nations). Soil carbon sequestration for improved land management: based on the work of Michel robert. Rome: World Soil Resources Reports; 2001.
Abeydeera LHUW, Mesthrige JW, Samarasinghalage TI. Global research on carbon emissions: a scientometric review. Sustainability. 2019;11(14):3972. https://doi.org/10.3390/su11143972.
Smith P. Carbon sequestration in croplands: the potential in Europe and the global context. J Agronomy. 2004. https://doi.org/10.1016/j.eja.2003.08.002.
Nair PKR, Kumar BM, Nair VD. An introduction to agroforestry: four decades of scientific developments. Cham: Springer International Publishing; 2021.
Plieninger T, Muñoz-Rojas J, Buck LE, Scherr SJ. Agroforestry for sustainable landscape management. Sustain Sci. 2020;15(5):1255–66. https://doi.org/10.1007/s11625-020-00836-4.
IPCC (The Intergovernmental Panel on Climate Change) (2003). Good Practice Guidance for Land Use, Land-Use Change and Forestry. National Greenhouse Gas Inventories Programme. ISBN 4–88788–003–0.
Lorenz K, Lal R. Soil organic carbon sequestration in agroforestry systems. Rev Agron Sustain Dev. 2014;34(2):443–54. https://doi.org/10.1007/s13593-014-0212-y.
Kassa G, Bekele T, Demissew S, Abebe T. Above- and belowground biomass and biomass carbon stocks in homegarden agroforestry systems of different age groups at three sites of southern and southwestern Ethiopia. Carbon Management. 2022;13(1):531–49. https://doi.org/10.1080/17583004.2022.2133743.
Kanmegne TD, Latifi H, Ullmann T, Baumhauer R, Bayala J, Thiel M. Estimation of aboveground biomass in agroforestry systems over three climatic regions in West Africa using sentinel-1, sentinel-2, ALOS, and GEDI data. Sensors. 2023;23:349. https://doi.org/10.3390/s23010349.
UNFCCC (United Nations Framework Convention on Climate Change). Challenges and opportunities for mitigation in the agricultural sector. J Diabetes Sci Technol. 2008;2(6):1157–60.
Ramachandran Nair PK, Mohan Kumar B, Nair VD. An introduction to agroforestry: four decades of scientific developments. Cham: Springer International Publishing; 2021.
Houghton RA, Hall F, Goetz SJ. Importance of biomass in the global carbon cycle. J Geophys Res. 2009. https://doi.org/10.1029/2009JG000935.
Handavu F, Syampungani S, Sileshi GW, Paxie WC. Aboveground and belowground tree biomass and carbon stocks in the miombo woodlands of the Copperbelt in Zambia. Carbon Management. 2021;12(3):307–21. https://doi.org/10.1080/17583004.2021.1926330.
Mbow C, Van Noordwijk M, Luedeling E, Neufeldt H, Minang PA, Kowero G. Agroforestry solutions to address food security and climate change challenges in Africa. Current Opin Environ Sustain. 2014;6(1):61–7. https://doi.org/10.1016/j.cosust.2013.10.014.
Marone D, Poirier V, Coyea M, Olivier A, Munson AD. Carbon storage in agroforestry systems in the semi-arid zone of Niayes Senegal. Agroforest Syst. 2017;91(5):941–54. https://doi.org/10.1007/s10457-016-9969-0.
Nair PKR. An Introduction to Agroforestry. Dordrecht: Kluwer Academic Publishers; 1993.
Mbow C, Toensmeier E, Brandt M, Skole D, Dieng M, Garrity D, Poulter B. Agroforestry as a solution for multiple climate change challenges in Africa. In: Deryng D, editor. Climate change and agriculture. Cambridge: Burleigh Dodds Science Publishing; 2020.
Skole DL, Mbow C, Mugabowindekwe M, Brandt MS, Samek JH. Trees outside of forests as natural climate solutions. Nat Clim Chang. 2021;11:1013–6. https://doi.org/10.1038/s41558-021-01230-3.
Bignebat C, Sakho-Jimbira MS. Migrations et diversification des activités économiques locales: Étude du Bassin arachidier du Sénégal. Mondes En Developpement. 2013;41(4):93–114. https://doi.org/10.3917/med.164.0093.
Kelly, V., Diagana, B., Reardon, T., Gaye, M., Crawford, E., (1996). “Cash Crop and food grain productivity in senegal: historical view, new survey evidence, and policy implication. MSU International Development Paper No.20, East Lansing, Michigan
Faye B, Du G. agricultural land transition in the “Groundnut Basin” of Senegal: 2009 to 2018. Land. 2021;10:996. https://doi.org/10.3390/land10100996.
Ricome A, Affholder F, Gérard F, Muller B, Poeydebat C, Quirion P, Sall M. Are subsidies to weather-index insurance the best use of public funds? a bio-economic farm model applied to the senegalese groundnut basin. Agric Syst. 2017;156:149–76.
Diallo M, Ndir K, Diop AM, Dieye B, Ndiaye S. Global analysis of millet-based household farms: Characterization of the Senegalese production system of Niayes and Groundnut basin areas. Afr J Agric Res. 2020;16(8):1133–40. https://doi.org/10.5897/ajar2019.14551.
Roquet D. Partir pour mieux durer : la migration comme réponse à la sécheresse au Sénégal ? Espace Populations Sociétés. 2008;1:37–53.
Ndong JB. L’évolution de la pluviométrie au Sénégal et les incidences de la sécheresse récente sur l’environnement/The evolution of rainfall in Senegal and the consequences of the recent drought on the environment. Rev De Geogaphie Lyon. 1995;70:193–8.
Kindt R, Coe R. Tree diversity analysis a manual and software for common statistical methods for ecological and biodiversity studies. Nairobi: World Agroforestry Centre (ICRAF); 2005.
Mbow C, Verstraete MM, Neufeldt H. Allometric models for aboveground biomass in dry savanna trees of the Sudan and Sudan-Guinean ecosystems of Southern Senegal. J For Res. 2014. https://doi.org/10.1007/s10310-013-0414-1.
Sinsin B, Glèlè Kakaï R. Structural description of two Isoberlinia dominated vegetation types in the Wari-Maro forest reserve (Benin). S Afr J Bot. 2009;75:43–51. https://doi.org/10.1016/j.sajb.2008.07.003.
Thiombiano, A., Glèlè Kakaï, R., Bayen, P., Boussim, J.I., Mahamane, A. (2015). Méthodes et dispositifs d’inventaires forestiers en afrique de l’ouest : état des lieux et propositions pour une harmonisation. Annales des sciences agronomiques—spécial 19(1). ISSN 1659 – 5009.
Sambou S, Faye LC, Diop FN, Dieng SD, Mbow C. Importance value index and species relative contribution to carbon stocks in savanna ecosystems: implications for climate change mitigation and forest management in Patako Forest (Senegal). Int J Biol Chem Sci. 2022;15(5):1770–82. https://doi.org/10.4314/ijbcs.v15i5.6.
Muhdi Muhdi D. Diversity, biomass, and carbon stock of understorey plants in the rubber agroforestry and rubber monoculture systems in Central Tapanuli District North Sumatra Indonesia. Biodiversitas. 2020;21(8):3508–18. https://doi.org/10.3057/biodiv/d210812.
Hairiah K, Dewi S, Agus F. Measurement of carbon reserves: from land level to landscape practical guide. 2nd ed. Bogor: World Agroforestry Center; 2011.
Agrawal A, Gopal K. Application of diversity index in measurement of species diversity biomonitoring of water and waste water. Berlin: Springer; 2013. https://doi.org/10.1007/978-81-322-0864-8_4.
Hill MO. Diversity and evenness: a unifying notation and its consequences. Ecology. 1973;54(2):427e432. https://doi.org/10.2307/1934352.
Philip MS. Measuring trees and forests. 2nd ed. London: CABI; 2002. p. 1–310.
Sambou A, Sambou B, Ræbild A. Farmers’ contributions to the conservation of tree diversity in the Groundnut Basin Senegal. J ForRes. 2017. https://doi.org/10.1007/s11676-017-0374-y.
Ketterings QM, Coe R, Van Noordwijk M, Ambagau Y, Palm CA. Reducing uncertainty in the use of allometric biomass equations for predicting aboveground tree biomass in mixed secondary forests For. Ecol Manage. 2001;146:1–39. https://doi.org/10.1016/S0378-1127(00)00460-6.
Ravindranath NH, Ostwald M. Carbon Inventory Methods Handbook for greenhouse gas inventory carbon mitigation and roundwood production projects. Adv Global Change Res. 2008. https://doi.org/10.1007/978-1-4020-6547-7.
Giri K, Buragohain P, Konwar S, Pradhan B, Mishra G, Meena DK. Tree diversity and ecosystem carbon stock assessment in Nambor Wildlife Sanctuary, Assam. Proc Natl Acad Sci India Sect B Biol Sci. 2018;89:1421–8. https://doi.org/10.1007/s40011-018-01072-8.
Brown S. Estimating biomass and biomass change of tropical forests: a primer. Rome: FAO Food and Agriculture Organization of the United Nations; 1997.
Chave J, Andalo C, Brown S, Cairns MA, Chambers JQ, Eamus D, Folster H, Fromard F, Higuchi N, Kira T, Lescure JP, Nelson BW, Ogawa H, Puig H, Riera B, Yamakura T. Tree allometry and improved estimation of carbon stocks and balance in tropical forests. Oecologia. 2005;145:87–99. https://doi.org/10.1007/s00442-005-0100-x.
Cairns MA, Helmer EH. Root biomass allocation in the world’s upland forests. Oecologia. 1997;111:1–11. https://doi.org/10.1007/s004420050201.
Petersson H, Holm S, Ståhl G, Alger D, Fridman J, Lehtonen A, Mäkipää R. Individual tree biomass equations or biomass expansion factors for assessment of carbon stock changes in living biomass. a comparative study. Forest Ecol Manage. 2012;270:78–84. https://doi.org/10.1016/j.foreco.2012.01.004.
Solomon N, Birhane E, Tadesse T, Treydte AC, Meles K. Carbon stocks and sequestration potential of dry forests under community management in Tigray Ethiopia. Ecol Process. 2017. https://doi.org/10.1186/s13717-017-0088-2.
Jones AR, Raja Segaran R, Clarke KD, Waycott M, Goh WSH, Gillanders BM. Estimating mangrove tree biomass and carbon content: a comparison of forest inventory techniques and drone imagery. Front Marine Sci. 2020. https://doi.org/10.3389/fmars.2019.00784.
Sharma, H., Pant, K. S., Bishist, R., Lal Gautam, K., Ludarmani, Dogra, R., Kumar, M., & Kumar, A. (2023). Estimation of biomass and carbon storage potential in agroforestry systems of north-western Himalayas, India. Catena, 225. https://doi.org/10.1016/j.catena.2023.107009.
Eze NM, Asogwa OC, Eze CM. Principal component factor analysis of some development factors in southern nigeria and its extension to regression analysis. J Adv Mathematics Computer Sci. 2021. https://doi.org/10.9734/jamcs/2021/v36i330351.
Le S, Josse J, Husson F. FactoMineR: an R package for multivariate analysis. J Stat Softw. 2008;25(1):118. https://doi.org/10.16837/jss.v025.i01.
Škornik S, Vidrih M, Kaligarič M. The effect of grazing pressure on species richness, composition and productivity in North Adriatic Karst pastures’. Plant Biosyst Int J Dealing Aspects Plant Biol. 2010;144(2):355–64. https://doi.org/10.1080/11263501003750250.
Wang J, Li W, Cao W, Wang S. Effects of different intensities of long-term grazing on plant diversity, biomass and carbon stock in alpine shrubland on the Qinghai-Tibetan Plateau. PeerJ. 2022;10: e12771. https://doi.org/10.7717/peerj.12771.
Yu J, Zhang X, Xu C, Hao M. Thinning can increase shrub diversity and decrease herb diversity by regulating light and soil environments. Front Plant Sci. 2022;13: 948648. https://doi.org/10.3389/fpls.2022.948648.
Prasad JVNS, Korwar GR, Rao KV, Srinivas K, Rama Rao CA, Srinivasarao C, Kulkarni HD. Effect of modification of tree density and geometry on intercrop yields and economic returns in Leucaena-based agroforestry systems for wood production in Andhra Pradesh, southern India. Experim Agric. 2010;46(02):155. https://doi.org/10.1017/s0014479709990858.
Zida D, Tigabu M, Sawadogo L, Tiveau D, Odén PC. Long-term effects of prescribed early fire, grazing and selective tree cutting on seedling populations in the Sudanian savanna of Burkina Faso. Afr J Ecol. 2009;47(1):97–108. https://doi.org/10.1111/j.1365-2028.2008.01011.x.
Balima LH, Marie B, Nacoulma I, Bayen P. Agricultural land use reduces plant biodiversity and carbon storage in tropical West African savanna ecosystems : Implications for sustainability. Global Ecol Conserv. 2020. https://doi.org/10.1016/j.gecco.2019.e00875.
Nath AJ, Sileshi GW, Laskar SY, Pathak K, Reang D, Nath A, Das AK. Quantifying carbon stocks and sequestration potential in agroforestry systems under divergent management scenarios relevant to India’s Nationally determined contribution. J Clean Prod. 2021;281: 124831. https://doi.org/10.1016/j.jclepro.2020.124831.
Erkan N, Uzun E, Aydin AC, Bas MN. Effect of pruning on diameter growth in Pinus brutia ten plantations in Turkey Croat. J Eng. 2012. https://doi.org/10.1016/j.gecco.2019.e00875.
Balima HL, Nacoulma MI, Bayen P. Agricultural land use reduces plant biodiversity and carbon storage in tropical West African savanna ecosystems: Implications for sustainability. Global Ecol Conserv. 2020. https://doi.org/10.1016/j.gecco.2019.e00875.
Baul TK, Chakraborty A, Nandi R, Mohiuddin M, Kilpeläinen A. Effects of tree species diversity and stand structure on carbon stocks of homestead forests in Maheshkhali Isl and Southern Bangladesh. Carbon Balance Management. 2021. https://doi.org/10.1186/s13021-021-00175-6.
Sida TS, Baudron F, Kim H, Giller KE. Climate-smart agroforestry: Faidherbia albida trees buffer wheat against climatic extremes in the Central Rift Valley of Ethiopia. Agric For Meteorol. 2018;248:339–47. https://doi.org/10.1016/j.agrformet.2017.10.013.
Tsedeke RE, Dawud SM, Tafere SM. Assessment of carbon stock potential of parkland agroforestry practice the case of Minjar Shenkora; North Shewa. Ethiopia Environ Syst Res. 2021;10:2. https://doi.org/10.1186/s40068-020-00211-3.
Woomer P, Tieszen L, Tappan G, Touré A, Sall M. Land use change and terrestrial carbon stocks in Senegal. J Arid Environ. 2004;59(3):625–42. https://doi.org/10.1016/j.jaridenv.2004.03.02.
Gebre AB, Birhane E, Gebresamuel G, Hadgu KM, Norgrove L. Woody species diversity and carbon stock under different land use types at Gergera watershed in eastern Tigray Ethiopia. Agroforest Syst. 2018. https://doi.org/10.1007/s10457-018-0226-6.
Gebrewahid Y, Meressa E. Tree species diversity and its relationship with carbon stock in the parkland agroforestry of Northern Ethiopia. Cogent Biol. 2020;6:1–1728945. https://doi.org/10.1080/23312025.2020.1728945.
Manaye A, Tesfamariam B, Tesfaye M, Worku A, Gufi Y. Tree diversity and carbon stocks in agroforestry systems in northern Ethiopia. Carbon Balance Manage. 2021. https://doi.org/10.1186/s13021-021-00174-7.
Hasen-yusuf M, Treydte AC, Abule E, Sauerborn J. Predicting aboveground biomass of woody encroacher species in semi-arid rangelands, Ethiopia. J Arid Environ. 2013;96:64–72. https://doi.org/10.1016/j.jaridenv.2013.04.007.
Sintayehu DW, Belayneh A, Dechassa N. Aboveground carbon stock is related to land cover and woody species diversity in tropical ecosystems of Eastern Ethiopia. Ecol Process. 2020. https://doi.org/10.1186/s13717-020-00237-6.
Sahoo UK, Tripathi OP, Nath AJ, Deb S. Quantifying tree diversity, carbon stocks, and sequestration potential for diverse land uses in Northeast. Front Environ Sci. 2021;9: 724950. https://doi.org/10.3389/fenvs.2021.724950.
Hartoyo APP, Khairunnisa S, Pamoengkas P, Solikhin A, Supriyanto S, Prasetyo IZ, Istomo LB. Estimating carbon stocks of three traditional agroforestry systems and their relationships with tree diversity and stand density. Biodiversitas. 2022;23(12):6137–46. https://doi.org/10.1057/biodiv/d231207.
Yasin G, Nawaz MF, Zubair M, Azhar MF, Gilani MM, Ashraf MN, Qin A, Rahman SU. Role of traditional agroforestry systems in climate change mitigation through carbon sequestration : an investigation from the Semi-Arid Region of Pakistan. Land. 2023. https://doi.org/10.3390/land12020513.
Acknowledgements
We are grateful for the support of WASCAL and the German Federal Ministry of Education and Research (BMBF). We thank the members of the Institute of Environmental Sciences Institute of Senegal and the Natural Ecosystems and Environment Research Unit (URENE). Special thanks are due to Mr Justin Diatta, Antoine Sambou and all those who made valuable contributions to this study.
Funding
This study was funded by the West African Science Service Centre on Climate Change and Adapted Land Use (WASCAL) through the German Federal Ministry of Education and Research (BMBF).
Author information
Authors and Affiliations
Contributions
MS design the study, the statistical analysis and drafted the manuscript. BK. and BS. carefully supervised the design and writing of the manuscript. SS. and SS. contributed to the design of the statistical analysis, and ensured the accuracy and reliability of the results. The conceptualisation and study design were enriched by the contributions of MD., AG., HS. and FN who brought their expertise to improve the research methodology. The final version of the manuscript was revised by CM, who improved the overall clarity of the content. All authors reviewed and approved the final version of the manuscript.
Corresponding author
Ethics declarations
Ethics approval and consent to participate
Not applicable.
Consent for publication
Not applicable.
Competing interests
The authors declare that they have no known conflicts of interest.
Additional information
Publisher's Note
Springer Nature remains neutral with regard to jurisdictional claims in published maps and institutional affiliations.
Supplementary Information
Rights and permissions
Open Access This article is licensed under a Creative Commons Attribution 4.0 International License, which permits use, sharing, adaptation, distribution and reproduction in any medium or format, as long as you give appropriate credit to the original author(s) and the source, provide a link to the Creative Commons licence, and indicate if changes were made. The images or other third party material in this article are included in the article's Creative Commons licence, unless indicated otherwise in a credit line to the material. If material is not included in the article's Creative Commons licence and your intended use is not permitted by statutory regulation or exceeds the permitted use, you will need to obtain permission directly from the copyright holder. To view a copy of this licence, visit http://creativecommons.org/licenses/by/4.0/.
About this article
Cite this article
Sambou, M., Koné, B., Sambou, S. et al. Variation of biomass carbon stock within agroforestry systems in the Senegalese groundnut basin. Discov Sustain 5, 35 (2024). https://doi.org/10.1007/s43621-024-00208-3
Received:
Accepted:
Published:
DOI: https://doi.org/10.1007/s43621-024-00208-3