Abstract
The unstable interface between lithium metal anodes and carbonate-based electrolytes is a key challenge limiting the cycling lifespan of high-energy lithium metal batteries. Here, a resilient artificial solid electrolyte interphase (RASEI) was designed by regulating poly(hexafluorobutyl acrylate) (PHFBA) matrix with the benzene-containing bisphenol A ethoxylate dimethacrylate (BAED) crosslinker to address this issue. The rigid BAED molecule can finely tune the flexible PHFBA matrix, enabling superior resilience from 600% elongation to 90% compression with a high Young’s modulus of over 2 MPa. RASEI with these characteristics can accommodate large volume changes of lithium metal and ensure the intimate contact between the lithium metal and the RASEI during battery operation. Consequently, it facilitated homogeneous lithium deposition while mitigating parasitic side reactions. Thanks to the tough and resilient nature of the fluorinated RASEI, the long-term cycling of Li∣∣Li symmetric cells can be achieved for over 500 h at 1 mA cm−2 and 1 mAh cm−2. The following post-mortem of cycled Li metal reveals that Li dendrite growth is effectively inhibited. Furthermore, the NCM811 pouch cell with a high cathode loading of 20 mg cm−2 exhibits a capacity retention of over 85% after 200 cycles at 1 C.

摘要
锂金属负极和碳酸酯类电解液之间不稳定的界面是限制高比能 锂金属电池循环寿命的关键挑战. 本文使用含苯环的双酚A乙氧基化物 二甲基丙烯酸酯(BAED)交联剂调节聚(丙烯酸六氟丁酯)(PHFBA), 设 计了一种弹性人造固体电解质中间相(RASEI)来解决这个问题. 刚性 BAED分子可以对柔性PHBA基体进行调控, 实现从600%伸长率到90% 压缩率的卓越回弹性, 并具有超过2 MPa的高杨氏模量. RASEI可以适 应锂金属较大的体积变化, 并确保电池运行过程中锂金属与RASEI之 间的紧密接触, 促进均匀的锂沉积并减少副反应. 因此, 经过RASEI修 饰的Li∣∣Li对称电池可以在1 mA cm−2和1 mAh cm−2下实现超过500小 时的长期循环. 对循环后锂金属进行测试分析表明锂枝晶的生长得到 了有效的抑制. 此外, 搭配20 mg cm−2高阴极负载的NCM811软包电池 在1 C下, 经过200次循环后容量保持率超过85%.
Similar content being viewed by others
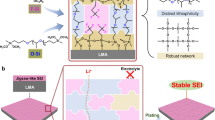
References
Lin D, Liu Y, Cui Y. Reviving the lithium metal anode for high-energy batteries. Nat Nanotech, 2017, 12: 194–206
Xu P, Shuang ZY, Zhao CZ, et al. A review of solid-state lithium metal batteries through in-situ solidification. Sci China Chem, 2023, 67: 67–86
Fang W, Wen Z, Chen L, et al. Constructing inorganic-rich solid electrolyte interphase via abundant anionic solvation sheath in commercial carbonate electrolytes. Nano Energy, 2022, 104: 107881
Yeddala M, Rynearson L, Lucht BL. Modification of carbonate electrolytes for lithium metal electrodes. ACS Energy Lett, 2023, 8: 4782–4793
Lu G, Nai J, Luan D, et al. Surface engineering toward stable lithium metal anodes. Sci Adv, 2023, 9: eadf1550
Xin S, Zhang X, Wang L, et al. Roadmap for rechargeable batteries: present and beyond. Sci China Chem, 2023, 67: 13–42
Tang W, Ma J, Zhang X, et al. Interfacial strategies towards highly stable Li-metal anode of liquid-based Li-metal batteries. Energy Storage Mater, 2024, 64: 103084
Chen XR, Zhao BC, Yan C, et al. Review on Li deposition in working batteries: from nucleation to early growth. Adv Mater, 2021, 33: 2004128
Choudhury S, Mangal R, Agrawal A, et al. A highly reversible room-temperature lithium metal battery based on crosslinked hairy nano-particles. Nat Commun, 2015, 6: 10101
Goodenough JB, Kim Y. Challenges for rechargeable Li batteries. Chem Mater, 2009, 22: 587–603
Zhu XF, Li X, Liang TQ, et al. Electrolyte perspective on stabilizing LiNi0.8Co0.1Mn0.1O2 cathode for lithium-ion batteries. Rare Met, 2023, 42: 387–398
Piao N, Liu S, Zhang B, et al. Lithium metal batteries enabled by synergetic additives in commercial carbonate electrolytes ACS Energy Lett, 2021, 6: 1839–1848
Xu X, Yue X, Chen Y, et al. Li Plating regulation on fast-charging graphite anodes by a triglyme-LiNO3 synergistic electrolyte additive. Angew Chem Int Ed, 2023, 62: e202306963
Wu Q, Li L, Zheng Y, et al. Achieving physical blocking and chemical electrocatalysis of polysulfides by using a separator coating layer in lithium–sulfur batteries. Sci China Mater, 2024, 67: 107–115
Song YH, Wu KJ, Zhang TW, et al. A nacre-inspired separator coating for impact-tolerant lithium batteries. Adv Mater, 2019, 31: 1905711
Huang X, He R, Li M, et al. Functionalized separator for next-generation batteries. Mater Today, 2020, 41: 143–155
Yang QY, Yu Z, Li Y, et al. Understanding and modifications on lithium deposition in lithium metal batteries. Rare Met, 2022, 41: 2800–2818
Zhang C, Yang Y, Sun Y, et al. 2D sp2-carbon-linked covalent organic frameworks as artificial SEI film for dendrite-free lithium metal batteries. Sci China Mater, 2023, 66: 2591–2600
Wu J, Rao Z, Liu X, et al. Polycationic polymer layer for air-stable and dendrite-free Li metal anodes in carbonate electrolytes. Adv Mater, 2021, 33: e2007428
Liu K, Pei A, Lee HR, et al. Lithium metal anodes with an adaptive “solid-liquid” interfacial protective layer. J Am Chem Soc, 2017, 139: 4815–4820
Yu Z, Cui Y, Bao Z. Design principles of artificial solid electrolyte interphases for lithium-metal anodes. Cell Rep Phys Sci, 2020, 1: 100119
Jagger B, Pasta M. Solid electrolyte interphases in lithium metal batteries. Joule, 2023, 7: 2228–2244
Han Z, Zhang C, Lin Q, et al. A protective layer for lithium metal anode: why and how. Small Methods, 2021, 5: e2001035
Chen L, Chen KS, Chen X, et al. Novel ALD chemistry enabled low-temperature synthesis of lithium fluoride coatings for durable lithium anodes. ACS Appl Mater Interfaces, 2018, 10: 26972–26981
Tan J, Matz J, Dong P, et al. A growing appreciation for the role of LiF in the solid electrolyte interphase. Adv Energy Mater, 2021, 11: 2100046
Wang W, Yue X, Meng J, et al. Lithium phosphorus oxynitride as an efficient protective layer on lithium metal anodes for advanced lithium-sulfur batteries. Energy Storage Mater, 2019, 18: 414–422
Chen L, Connell JG, Nie A, et al. Lithium metal protected by atomic layer deposition metal oxide for high performance anodes. J Mater Chem A, 2017, 5: 12297–12309
Yan C, Cheng XB, Yao YX, et al. An armored mixed conductor interphase on a dendrite-free lithium-metal anode. Adv Mater, 2018, 30: e1804461
Guo JC, Tan SJ, Zhang CH, et al. A self-reconfigured, dual-layered artificial interphase toward high-current-density quasi-solid-state lithium metal batteries. Adv Mater, 2023, 35: e2300350
Alaboina PK, Rodrigues S, Rottmayer M, et al. In situ dendrite suppression study of nanolayer encapsulated Li metal enabled by zirconia atomic layer deposition. ACS Appl Mater Interfaces, 2018, 10: 32801–32808
Pathak R, Chen K, Gurung A, et al. Fluorinated hybrid solid-electrolyte-interphase for dendrite-free lithium deposition. Nat Commun, 2020, 11: 93
Gu M, Rao AM, Zhou J, et al. In situ formed uniform and elastic SEI for high-performance batteries. Energy Environ Sci, 2023, 16: 1166–1175
Chen C, Zhang J, Hu B, et al. Dynamic gel as artificial interphase layer for ultrahigh-rate and large-capacity lithium metal anode. Nat Commun, 2023, 14: 4018
Tamate R, Peng Y, Kamiyama Y, et al. Extremely tough, stretchable gel electrolytes with strong interpolymer hydrogen bonding prepared using concentrated electrolytes to stabilize lithium-metal anodes. Adv Mater, 2023, 35: 2211679
Li Q, Zeng FL, Guan YP, et al. Poly (dimethylsiloxane) modified lithium anode for enhanced performance of lithium-sulfur batteries. Energy Storage Mater, 2018, 13: 151–159
Yang N, Cui Y, Su H, et al. A chemically bonded ultraconformal layer between the elastic solid electrolyte and lithium anode for high-performance lithium metal batteries. Angew Chem Int Ed, 2023, 62: e202304339
Han J, Lee MJ, Min JH, et al. Fluorine-containing phase-separated polymer electrolytes enabling high-energy solid-state lithium metal batteries. Adv Funct Mater, 2024, 34: 2310801
Huang J, Shen Z, Robertson SJ, et al. Fluorine grafted gel polymer electrolyte by in situ construction for high-voltage lithium metal batteries. Chem Eng J, 2023, 475: 145802
Yang B, Pan Y, Li T, et al. High-safety lithium metal pouch cells for extreme abuse conditions by implementing flame-retardant perfluorinated gel polymer electrolytes. Energy Storage Mater, 2024, 65: 103124
Xue T, Qian J, Guo X, et al. Tailoring fluorine-rich solid electrolyte interphase to boost high efficiency and long cycling stability of lithium metal batteries. Sci China Chem, 2023, 66: 2121–2129
Wang Y, Wu Z, Azad FM, et al. Fluorination in advanced battery design. Nat Rev Mater, 2024, 9: 119–133
Liang J, Chen Q, Liao X, et al. A nano-shield design for separators to resist dendrite formation in lithium-metal batteries. Angew Chem Int Ed, 2020, 59: 6561–6566
Deng Z, Huang Z, Shen Y, et al. Ultrasonic scanning to observe wetting and “unwetting” in Li-ion pouch cells. Joule, 2020, 4: 2017–2029
Deng Z, Lin X, Huang Z, et al. Recent progress on advanced imaging techniques for lithium-ion batteries. Adv Energy Mater, 2020, 11: 2000806
Acknowledgements
This work was supported by National Key R&D Program of China (2023YFB2503801), the National Natural Science Foundation of China (Grant Nos. 52302253, and 5202780089), the Key Program of the National Natural Science Foundation of China (Grant No. 52231009), the Fundamental Research Funds for the Central Universities (HUST: 2172020kfyXJJS089), Key R&D Program of Hubei Province (2023BAB028). The authors thank the Analytical and Testing Centre of HUST and the State Key Laboratory of Materials Processing and Die & Mold Technology of HUST for XRD and SEM measurements. The authors thank the Neware Technology Limited for the electrochemical testing. Thanks to eceshi (www.eceshi.com) for FTIR.
Author information
Authors and Affiliations
Contributions
Author contributions Kong J and Hou T conceived the project. Kong J designed the experiments and collected all data, Deng X, Li J helped with the characterization and data analysis. Kong J wrote the paper with support from Shi T, Hou T. Li D, Xu H and Huang Y provided critical feedback and helped shape the manuscript.
Corresponding authors
Ethics declarations
Conflict of interest The authors declare no conflict of interest.
Additional information
Supplementary information Experimental details and supporting data are available in the online version of the paper.
Jia Kong is currently a Master degree candidate at the School of Materials Science and Engineering, Huazhong University of Science and Technology. His current research mainly focuses on lithium metal batteries.
Henghui Xu received his PhD at Huazhong University of Science and Technology (HUST) in 2015, followed by a five-year postdoctoral research at The University of Texas at Austin. He is now a Professor of materials science in HUST working on solid-state batteries.
Yunhui Huang received his BS, MS, and PhD degrees from Peking University. From 2002 to 2004, he worked as an associate professor at Fudan University. He then worked with Prof. John B. Goodenough at the University of Texas at Austin for more than three years. In 2008, he became a chair professor of materials science at Huazhong University of Science and Technology. His research group works on rechargeable batteries and electrode materials.
Rights and permissions
About this article
Cite this article
Kong, J., Hou, T., Shi, T. et al. A tough, resilient, and fluorinated solid-electrolyte interphase stabilizing lithium metal in carbonate electrolytes. Sci. China Mater. 67, 1403–1411 (2024). https://doi.org/10.1007/s40843-024-2914-x
Received:
Accepted:
Published:
Issue Date:
DOI: https://doi.org/10.1007/s40843-024-2914-x