Abstract
To prevent global warming, improvements in the efficiency of internal combustion engines and the introduction of hybrid electric vehicles are gaining popularity as ways of controlling carbon dioxide emissions. Because of the lower average temperature of the exhaust gases from these systems, catalytic reactions mediated by an electric field have attracted attention because catalysts can have higher catalytic activity at lower temperatures than conventional catalysts. In addition, they consume less power than electrically heated catalysts. In this study, we determined the catalytic activity of a palladium/ceria-zirconia catalyst in an electric field with exhaust gas temperatures lower than conventional gas temperatures. Further evaluation using catalytic materials with modified ceria-zirconia ratios revealed the importance of the electrical resistance of the materials during electric field–mediated catalytic reactions. Upon modulating the applied current, the current strength was found to be related to a change in the electrical resistance of the catalyst during the reaction. Furthermore, we observed that the activity and electrical resistance of the catalysts were intrinsically linked. These results suggest that electron-promoted surface proton transport and intra-lattice oxygen defects in metal oxide catalysts, as well as their structural changes, significantly contribute to their catalytic activity in an electric field. These electric field–mediated catalytic reactions using modified catalysts can adapt to the growing shift in engine operating conditions by ensuring that the benefits associated with the use of hybrid vehicles and high-efficiency combustion engines are not offset by an increase in the production of carbon monoxide, nitrogen oxide, and unburned hydrocarbons.









Similar content being viewed by others
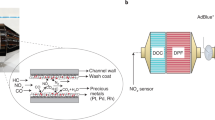
Data Availability
The datasets generated and/or analyzed during the current study are available from the corresponding author on reasonable request.
References
Adelman, ZE.: A reevaluation of the carbon bound-IV photochemical mechanism. MS. thesis, University of North Carolina, USA, (1999)
Avery, R.J.: Reactivity-based VOC control for solvent products: more efficient ozone reduction strategies. Environ. Sci. Technol. 40, 4845–4850 (2006). https://doi.org/10.1021/es060296u
Castell, N., Mantilla, E., Stein, A.F., et al.: A modeling study of the impact of a power plant on ground-level ozone in relation to its location: southwestern Spain as a case study. Water. Air. Soil. Pollut. 209, 61–79 (2010). https://doi.org/10.1007/s11270-009-0181-y
Environmental Protection Agency of United States. Regulations for emissions from vehicles and engines, https://www.epa.gov/regulations-emissions-vehicles-and-engines/regulations-onroad-vehicles-and-engines (2021). Accessed 28 May 2023
Internal Market, Industry, Entrepreneurship and SMEs of European- Union. Emissions in the automotive sector, https://www.ec.europa.eu/growth/sectors/automotive/environment-protection/emissions_en (2019). Accessed 28 May 2023
Ministry of the Environment of Government of Japan. Motor vehicle exhaust emission standards, https://www.env.go.jp/en/air/aq/mv/standards.html (2016). Accessed 28 May 2023
Kawaguchi, B., Umemoto, K., Misawa, S., Hirooka, S. et al.: ICE vehicle challenge toward zero emissions: future technology harmonization in electrified powertrain system. SAE Technical Paper 2019–01–2217 (2019). https://doi.org/10.4271/2019-01-2217
Kaniyu, J., Sakatani, S., Matsumura, E., and Kitamura, T.: Analysis of spray feature injected by tailpipe injector for aftertreatment of diesel engine emissions. SAE Technical Paper 2017–01–2373 (2017). https://doi.org/10.4271/2017-01-2373.
Uenishi, T., Umemoto, K., Yoshida, K., et al.: Development of the design methodology for a new De-NOx system. Int. J. Automot. Eng. 5, 115–120 (2014). https://doi.org/10.20485/jsaeijae.5.3_115
Johansson, Å., Wallin, U., Karlsson, M., Isaksson, A. et al.: Investigation on uniformity indices used for diesel exhaust aftertreatment systems. SAE Technical Paper 2008–01–0613 (2008). https://doi.org/10.4271/2008-01-0613
Martin, A., Will, N., Bordet, A., Cornet, P. et al.: Effect of flow distribution on emissions performance of catalytic converters. SAE Technical Paper 980936 (1998). https://doi.org/10.4271/980936
Weltens, H., Bressler, H., Terres, F., Neumaier, H. et al.: Optimisation of catalytic converter gas flow distribution by CFD prediction. SAE Technical Paper 930780 (1993). https://doi.org/10.4271/930780
Torkashvand, B., Maier, L., Hettel, M., Schedlbauer, T., et al.: On the challenges and constrains of ultra-low emission limits: formaldehyde oxidation in catalytic sinusoidal-shaped channels. Chem. Eng. Sci. 195, 841–850 (2018). https://doi.org/10.1016/j.ces.2018.10.031
Adams, K.M., Cavataio, J.V., Hammerle, R.H.: Lean NOx catalysis for diesel passenger cars: investigating effects of sulfur dioxide and space velocity. Appl. Catal. B: Environ. 10, 157–181 (1996). https://doi.org/10.1016/0926-3373(96)00029-X
Subramanian, N.D., Gao, J., Mo, X., et al.: La and/or V oxide promoted Rh/SiO2 catalysts: effect of temperature, H2/CO ratio, space velocity, and pressure on ethanol selectivity from syngas. J. Catal. 272, 204–209 (2010). https://doi.org/10.1016/j.jcat.2010.03.019
Martin AP, Will NS, Bordet A, et al.: Effect of flow distribution on emissions performance of catalytic converters. SAE Int. J. Fuels Lubr. 107, 384–390 (1998). https://www.jstor.org/stable/44746459
Korotkikh, O., Farrauto, R.: Selective catalytic oxidation of CO in H2: fuel cell applications. Catal. Today 62, 249–254 (2000)
Balakotaiah, V.: On the relationship between Aris and Sherwood numbers and friction and effectiveness factors. Chem. Eng. Sci. 63, 5802–5812 (2008). https://doi.org/10.1016/j.ces.2008.08.025
Bissett, E.J.: An asymptotic solution for washcoat pore diffusion in catalytic monoliths. Emiss. Control Sci. Technol. 1, 3–16 (2015). https://doi.org/10.1007/s40825-015-0010-2
Rink, J., Mozaffari, B., Tischer, S., et al.: Real-time simulation of dual-layer catalytic converters based on the internal mass transfer coefficient approach. Top. Catal. 60, 225–229 (2017). https://doi.org/10.1007/s11244-016-0602-2
Yamamoto, O., Matsuo, Y., Tosa, S., Okayama, T. et al.: Numerical modeling study of detailed gas diffusivity into catalyst washcoat for lean NOx catalyst. SAE Technical Paper 2019–01–0993 (2019). https://doi.org/10.4271/2019-01-0993
Wang, X., Chen, H., Sachtler, W.M.H.: Selective reduction of NOx with hydrocarbons over Co/MFI prepared by sublimation of CoBr2 and other methods. Appl. Catal. B: Environ. 29, 47–60 (2001). https://doi.org/10.1016/S0926-3373(00)00186-7
Matsumoto, S., Yokota, K., Doi, H.: Research on new DeNOx catalysts for automotive engines. Catal. Today 22, 127–146 (1994). https://doi.org/10.1016/0920-5861(94)80097-9
Witzel, F., Sill, G.A., Hall, W.K.: Reaction studies of the selective reduction of NO by various hydrocarbons. J. Catal. 149, 229–237 (1994). https://doi.org/10.1006/jcat.1994.1289
Dzwigaj, S., Janas, J., Gurgul, J., et al.: Do Cu(II) ions need Al atoms in their environment to make CuSiBEA active in the SCR of NO by ethanol or propane? A spectroscopy and catalysis study. Appl. Catal. B: Environ. 85, 131–138 (2009). https://doi.org/10.1016/j.apcatb.2008.07.003
Iliopoulou, E.F., Evdou, A.P., Lemonidou, A.A., et al.: Ag/alumina catalysts for the selective catalytic reduction of NOx using various reductants. Appl. Catal. A: Gen. 274, 179–189 (2004). https://doi.org/10.1016/j.apcata.2004.06.052
Shimizu, K., Satsuma, A., Hattori, T., et al.: Catalytic performance of Ag–Al2O3 catalyst for the selective catalytic reduction of NO by higher hydrocarbons. Appl. Catal. B: Environ. 25, 239–247 (2000). https://doi.org/10.1016/j.apcata.2004.06.052
Shibata, J., Shimizu, K., Satsuma, A., et al.: Influence of hydrocarbon structure on selective catalytic reduction of NO by hydrocarbons over Cu-Al2O3. Appl. Catal. B: Environ. 37, 197–204 (2002). https://doi.org/10.1016/S0926-3373(01)00336-8
Bisaiji, Y., Yoshida, K., Inoue, M., Umemoto, K., et al.: Development of Di-Air - a new diesel deNOx system by adsorbed intermediate reductants. SAE Int. J. Fuels Lubr. 5(1), 380–388 (2012). https://doi.org/10.4271/2011-01-2089
Bisaiji, Y., Yoshida, K., Inoue, M., Takagi, N., et al.: Reaction mechanism analysis of di-air-contributions of hydrocarbons and intermediates. SAE Int. J. Fuels Lubr. 5(3), 1310–1316 (2012). https://doi.org/10.4271/2012-01-1744
Uenishi, T., Shigeno, G., Shigeno, G. et al.: Research on numerical analysis code of oxidation behavior of hydrocarbon on diesel oxidation catalyst. In: The Ninth International Conference on Modeling and Diagnostics for Advanced (ed Tomita E), Okayama, Japan, 25 July-28 July 2017, Tokyo: JSME
Shigeno, G., Shigeno, G., Uenishi, T., et al.: The numerical analysis for the effect of higher hydrocarbon on diesel oxidation catalyst oxidation reaction. Tran. Soc. Auto. Eng. J. 49, 1097–1102 (2018). https://doi.org/10.11351/jsaeronbun.49.1097
Shigeno, G., Nishiyama, H., Uenishi, T., et al.: The experimental analysis for the effect of hydrocarbon in species and concentration on diesel oxidation catalysts oxidation reaction. Tran. Soc. Auto. Eng. J. 48, 609–614 (2017). https://doi.org/10.11351/jsaeronbun.48.609
Shigeno, G., Hata, H., Shigeno, G., et al.: The experimental analysis for the effect of competitive adsorption of multicomponent hydrocarbons on diesel oxidation catalysts oxidation Reaction. Tran. Soc. Auto. Eng. J. 49, 1114–1119 (2018). https://doi.org/10.11351/jsaeronbun.49.1114
Tsuchiya, A., Masaoka, S., Ohyama, J., et al.: Effects of carbon number and bond saturation on hydrocarbon combustion over a diesel oxidation catalyst. Catal. Sci. Technol. 10, 3868–3874 (2020). https://doi.org/10.1039/D0CY00017E
Masaoka, S., Tsuchiya, A., Satsuma, A., et al.: Studies on the poisoning effect of saturated and unsaturated hydrocarbons on the surface of diesel oxidation catalysts. 122nd Annual Conference of Catalysis Society of Japan, 2E06, (2018)
Uenishi, T.: Study on the effect of hydrocarbon structure on the reactivity of the three-way catalytic converter. Int. J. Engine Res. 24, 2772–2782 (2023). https://doi.org/10.1177/14680874221133435
Ohnishi, T., Kawakami, K., Nishioka, M., Ogura, M.: Direct decomposition of NO on metal-loaded zeolites with coexistence of oxygen and water vapor under unsteady-state conditions by NO concentration and microwave rapid heating. Catal. Today 281, 566–574 (2016). https://doi.org/10.1016/j.cattod.2016.07.012
Jing, C., Kan, Z., Mei, W.: Experimental study on the plasma purification for diesel engine exhaust gas. Earth. Environ. Sci. 113, 012186 (2018). https://doi.org/10.1088/1755-1315/113/1/012186
Oshima, K., Tanaka, K., Yabe, T., Kikuchi, E., Sekine, Y.: Oxidative coupling of methane using carbon dioxide in an electric field over La-ZrO2 catalyst at low external temperature. Fuel 107, 879–881 (2013). https://doi.org/10.1016/j.fuel.2013.01.058
Manabe, R., Nakatsubo, H., Gondo, A., Murakami, K., Ogo, S., Tsuneki, H., Ikeda, M., Ishikawa, A., Nakai, H., Sekine, Y.: Electrocatalytic synthesis of ammonia by surface proton hopping. Chem. Sci. 8, 5434–5439 (2017). https://doi.org/10.1039/C7SC00840F
Takise, K., Sato, A., Murakami, K., Ogo, S., Seo, J.G., Imagawa, K., Kado, S., Sekine, Y.: Irreversible catalytic methylcyclohexane dehydrogenation by surface protonics at low temperature. RSC Adv. 9, 5918–5924 (2019). https://doi.org/10.1039/C9RA00407F
Mukai, D., Tochiya, S., Murai, Y., Imori, M., Hashimoto, T., Sugiura, Y., Sekine, Y.: Role of support lattice oxygen on steam reforming of toluene for hydrogen production over Ni/La0.7Sr0.3AlO3-d catalyst. Appl. Catal. A. 453, 60–70 (2013). https://doi.org/10.1016/j.apcata.2012.11.040
Yabe, T., Mitarai, K., Oshima, K., Ogo, S., Sekine, Y.: Low-temperature dry reforming of methane to produce syngas in an electric field over La-doped Ni/ZrO2 catalysts. Fuel Process. Technol. 158, 96–103 (2017). https://doi.org/10.1016/j.fuproc.2016.11.013
Sakurai, S., Ogo, S., Sekine, Y.: Hydrogen production by steam reforming of ethanol over Pt/CeO2 catalyst in electric field at low temperature. J. Jpn. Petrol. Inst. 59, 174–183 (2016). https://doi.org/10.1627/jpi.59.174
Oshima, K., Shinagawa, T., Haraguchi, M., Sekine, Y.: Low temperature hydrogen production by catalytic steam reforming of methane in an electric field. Int. J. Hydrog. 38, 3003–3011 (2013). https://doi.org/10.1016/j.ijhydene.2012.12.069
Inagaki, R., Manabe, R., Hisai, Y., Kamite, Y., Yabe, T., Ogo, S., Sekine, Y.: Steam reforming of dimethyl ether promoted by surface protonics in an electric field Int. J. Hydrog. 43, 14310–14318 (2018). https://doi.org/10.1016/j.ijhydene.2018.05.164
Shigemoto, A., Higo, T., Narita, Y., Yamazoe, S., Uenishi, T., Sekine, Y.: Elucidation of catalytic NOx reduction mechanism in an electric field at low temperatures. Catal. Sci. Technol. 12, 4450–4455 (2022). https://doi.org/10.1039/D2CY00129B
Omori, Y., Shigemoto, A., Sugihara, K., Higo, T., Uenishi, T., Sekine, Y.: Electrical promotion-assisted automotive exhaust catalyst: highly active and selective NO reduction to N2 at low-temperatures. Catal. Sci. Technol. 11, 4008–4011 (2021). https://doi.org/10.1039/D1CY00591J
Uenishi, T., Shigemoto, A., Omori, Y., Higo, T., Ogo, S., Sekine, Y.: Three-way catalytic reaction in an electric field for exhaust emission control application. SAE Technical Paper 2021–01–0573 (2021). https://doi.org/10.4271/2021-01-0573
Nakano, N., Torimoto, M., Sampei, H., Yamashita, R., Yamano, R., Saegusa, K., Motomura, A., Nagakawa, K., Tsuneki, H., Ogo, S., Sekine, Y.: Elucidation of the reaction mechanism on dry reforming of methane in an electric field by in-situ DRIFTs. RSC Adv. 12, 9036–9043 (2022). https://doi.org/10.1039/D2RA00402J
Matsuda, T., Ishibashi, R., Koshizuka, Y., Tsuneki, H., Sekine, Y.: Quantitative investigation of CeO2 surface proton conduction in H2 atmosphere. Chem. Comm. 58, 10789–10792 (2022). https://doi.org/10.1039/D2CC03687H
Burch, R., Breen, J.P., Meunier, F.C.: A review of the selective reduction of NOx with hydrocarbons under lean-burn conditions with non-zeolitic oxide and platinum group metal catalysts. Appl. Catal. B 39, 283–303 (2002). https://doi.org/10.1016/S0926-3373(02)00118-2
Higo, T., Ueno, K., Omori, Y., Tsuchiya, H., et al.: Perovskite lattice oxygen contributes to low-temperature catalysis for exhaust gas cleaning. RSC Adv. 9, 22721–22728 (2019). https://doi.org/10.1039/C9RA03050F
Stotz, H., Maier, L., Deutschmann, O.: Methane oxidation over palladium: on the mechanism in fuel-rich mixtures at high temperatures. Top. Catal. 60, 83–109 (2017). https://doi.org/10.1007/s11244-016-0717-5
Hartmann, M., Maier, L., Minh, H.D., Deutschmann, O.: Catalytic partial oxidation of iso-octane over rhodium catalysts: an experimental, modeling, and simulation study. Combust. Flame 157, 1771–1782 (2010). https://doi.org/10.1016/j.combustflame.2010.03.005
Oshima, K., Shinagawa, T.: Sekine Y : Methane conversion assisted by plasma or electric field. J. Japan Pet. Inst. 56, 11–21 (2013). https://doi.org/10.1627/jpi.56.11
Acknowledgements
The authors would like to thank Editage (www.editage.com) for the English language editing. The authors acknowledge the technical support from the members of the Higashifuji Technical Center at Toyota Motor Corporation.
Author information
Authors and Affiliations
Contributions
Toru Uenishi: Conceptualization; Data curation; Formal analysis; Investigation; Methodology; Software; Validation; Visualization; Funding acquisition; Project administration; Resources; Supervision; original draft, Yasushi Sekine: Conceptualization; Writing—review & editing.
Corresponding author
Ethics declarations
Competing interests
The authors have no relevant financial or non-financial interests to disclose.
Additional information
Publisher's Note
Springer Nature remains neutral with regard to jurisdictional claims in published maps and institutional affiliations.
Rights and permissions
Springer Nature or its licensor (e.g. a society or other partner) holds exclusive rights to this article under a publishing agreement with the author(s) or other rightsholder(s); author self-archiving of the accepted manuscript version of this article is solely governed by the terms of such publishing agreement and applicable law.
About this article
Cite this article
Uenishi, T., Sekine, Y. The Effect of Catalyst Composition on Electric Field-Mediated Catalytic Reactions for Exhaust Emission Control. Emiss. Control Sci. Technol. 9, 189–199 (2023). https://doi.org/10.1007/s40825-023-00230-3
Received:
Revised:
Accepted:
Published:
Issue Date:
DOI: https://doi.org/10.1007/s40825-023-00230-3