Abstract
The objective of the paper is to perform a systematic investigation of a flapping wing in inclined stroke-plane hovering which is observed in insects such as dragonflies, true hoverflies and Coleopteran. Numerical simulations are performed at pitch amplitudes (15° ≤ B ≤ 75°) in conjunction with other kinematic parameters such as stroke-plane inclination (10° ≤ β ≤ 80°), stroke amplitude (0.5 ≤ Ao/c ≤ 5), heave-pitch phase difference (− 45° ≤ φ ≤ 90°) and Reynolds number (15.7 ≤ Re ≤ 10,000). Moving mesh strategy implemented in finite volume code is used to simulate the flapping motion. The aerodynamic performance and vortex structures are obtained for each parametric space. Results indicate that the maximum time-averaged vertical force coefficient \(\overline{{C_{\text{v}} }}\) is obtained at B ≈ 30°–40°, β ≈ 52°–60°, Ao/c ≈ 2–4 and φ ≈ + 27°–+ 60°. On the other hand, the maximum lifting efficiency ηl is obtained at B ≈ 42°–57°, β ≈ 50°–70°, Ao/c ≈ 1–2 and φ ≈ 0°–+ 15°. Vortex structures show that the strength, growth and position of LEV and TEV play a significant role in the vertical force generation. To the best of author’s knowledge, this is the first work which has discussed the significance of pitch amplitude in inclined hovering insects. In addition, the best operating conditions are determined by mapping \(\overline{{C_{\text{v}} }}\) and ηl over a wide parametric space. The optimal parameters are then compared with the existing experimental results that show a good match.




















Similar content being viewed by others
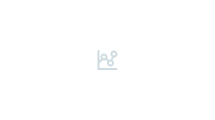
References
Weis-Fogh T (1973) Quick estimates of flight fitness in hovering animals, including novel mechanisms for lift production. J Exp Biol 59(1):169–230
Norberg RÅ (1975) Hovering flight of the dragonfly Aeschna juncea L., kinematics and aerodynamics. In: Swimming and flying in nature, Springer, Boston, MA, pp 763–781
Wang ZJ (2000) Two dimensional mechanism for insect hovering. Phys Rev Lett 85(10):2216
Wang ZJ (2004) The role of drag in insect hovering. J Exp Biol 207(23):4147–4155
Hsieh CT, Chang CC, Chu CC (2009) Revisiting the aerodynamics of hovering flight using simple models. J Fluid Mech 623:121–148
Sudhakar Y, Vengadesan S (2010) Flight force production by flapping insect wings in inclined stroke plane kinematics. Comput Fluids 39(4):683–695
Jones SK, Laurenza R, Hedrick TL, Griffith BE, Miller LA (2015) Lift vs. drag based mechanisms for vertical force production in the smallest flying insects. J Theor Biol 384:105–120
Kim D, Choi H (2007) Two-dimensional mechanism of hovering flight by single flapping wing. J Mech Sci Technol 21(1):207–221
Ellington C (1995) Unsteady aerodynamics of insect flight. In: Symposia of the society for experimental biology, pp 109–129
Dickinson MH, Lehmann FO, Sane SP (1999) Wing rotation and the aerodynamic basis of insect flight. Science 284(5422):1954–1960
Le TQ, Byun D, Ko JH, Park HC, Kim M (2010) Numerical investigation of the aerodynamic characteristics of a hovering Coleopteran insect. J Theor Biol 266(4):485–495
Mou XL, Liu YP, Sun M (2011) Wing motion measurement and aerodynamics of hovering true hoverflies. J Exp Biol 214(17):2832–2844
Birch JM, Dickson WB, Dickinson MH (2004) Force production and flow structure of the leading edge vortex on flapping wings at high and low Reynolds numbers. J Exp Biol 207(7):1063–1072
Ansari SA, Żbikowski R, Knowles K (2006) Non-linear unsteady aerodynamic model for insect-like flapping wings in the hover. Part 2: implementation and validation. P I Mech Eng G-J Aer 220(3):169–186
Wang ZJ, Birch JM, Dickinson MH (2004) Unsteady forces and flows in low Reynolds number hovering flight: two-dimensional computations vs robotic wing experiments. J Exp Biol 207(3):449–460
Li Q, Zheng M, Pan T, Su G (2018) Experimental and numerical investigation on dragonfly wing and body motion during voluntary take-off. Sci Rep 8(1):1011
Eldredge JD, Jones AR (2019) Leading-edge vortices: mechanics and modeling. Annu Rev Fluid Mech 51(1):75–104
Nabawy MR, Crowther WJ (2017) The role of the leading edge vortex in lift augmentation of steadily revolving wings: a change in perspective. J R Soc Interface 14(132):20170159
Bhat SS, Zhao J, Sheridan J, Hourigan K, Thompson MC (2019) Uncoupling the effects of aspect ratio, Reynolds number and Rossby number on a rotating insect-wing planform. J Fluid Mech 859:921–948
Phillips N, Knowles K, Bomphrey RJ (2015) The effect of aspect ratio on the leading-edge vortex over an insect-like flapping wing. Bioinspir Biomim 10(5):056020
Harbig RR, Sheridan J, Thompson MC (2014) The role of advance ratio and aspect ratio in determining leading-edge vortex stability for flapping flight. J Fluid Mech 751:71–105
Lee YJ, Lua KB, Lim TT (2016) Aspect ratio effects on revolving wings with Rossby number consideration. Bioinspir Biomim 11(5):056013
Ellington C (2006) Insects versus birds: the great divide. In: 44th AIAA aerospace sciences meeting and exhibit. https://doi.org/10.2514/6.2006-35
Ellington CP, Usherwood JR (2001) Lift and drag characteristics of rotary and flapping wings. In: Fixed and flapping wing aerodynamics for micro air vehicle applications, Progress in astronautics and aeronautics, AIAA, pp. 231–248. https://doi.org/10.2514/5.9781600866654.0231.0248
Zhu J, Jiang L, Zhao H, Tao B, Lei B (2015) Numerical study of a variable camber plunge airfoil under wind gust condition. J Mech Sci Technol 29(11):4681–4690
Zymaris AS, Papadimitriou DI, Giannakoglou KC, Othmer C (2009) Continuous adjoint approach to the Spalart-Allmaras turbulence model for incompressible flows. Comput Fluids 38(8):1528–1538
Fluent ANSYS (2012) 14.5 theory guide. ansys Inc, Canonsburg, PA
Gao T, Lu XY (2008) Insect normal hovering flight in ground effect. Phys Fluids 20(8):087101
Alexander DE (1984) Unusual phase relationships between the forewings and hindwings in flying dragonflies. J Exp Biol 109(1):379–383
Rüppell G (1989) Kinematic analysis of symmetrical flight manoeuvres of Odonata. J Exp Biol 144(1):13–42
Ellington CP (1984) The aerodynamics of hovering insect flight. IV. Aerodynamic mechanisms. Phil Trans R Soc Lond B 305(1122):79–113
Wakeling JM, Ellington CP (1997) Dragonfly flight. II. Velocities, accelerations and kinematics of flapping flight. J Exp Biol 200(3):557–582
Dickinson MH, Gotz KG (1993) Unsteady aerodynamic performance of model wings at low Reynolds numbers. J Exp Biol 174(1):45–64
Khan ZA, Agrawal SK (2011) Optimal hovering kinematics of flapping wings for micro air vehicles. AIAA J 49(2):257–268
Wang Q, Goosen JF, Van Keulen A (2013) Optimal hovering kinematics with respect to various flapping-wing shapes. In: IMAV 2013, Proceedings of the international micro air vehicle conference and flight competition, Toulouse, France
Lua KB, Zhang XH, Lim TT, Yeo KS (2015) Effects of pitching phase angle and amplitude on a two-dimensional flapping wing in hovering mode. Exp Fluids 56(2):35
Chen Y, Gravish N, Desbiens AL, Malka R, Wood RJ (2016) Experimental and computational studies of the aerodynamic performance of a flapping and passively rotating insect wing. J Fluid Mech 791:1–33
Azuma A, Watanabe T (1988) Flight performance of a dragonfly. J Exp Biol 137(1):221–252
Wakeling JM (1993) Dragonfly aerodynamics and unsteady mechanisms: a review. Odonatologica 22(3):319–334
Ellington CP (1984) The aerodynamics of flapping animal flight. Am Zool 24(1):95–105
Lua KB, Lim TT, Yeo KS (2011) Effect of wing–wake interaction on aerodynamic force generation on a 2D flapping wing. Exp Fluids 51(1):177–195
Acknowledgements
This work was supported by Basic Science Research Program through the National Research Foundation of Korea (NRF) funded by the Ministry of Education [NRF-2017R1D1A1B03032472].
Author information
Authors and Affiliations
Corresponding author
Additional information
Technical Editor: André Cavalieri.
Publisher's Note
Springer Nature remains neutral with regard to jurisdictional claims in published maps and institutional affiliations.
Rights and permissions
About this article
Cite this article
Shanmugam, A.R., Sohn, C.H. Systematic investigation of a flapping wing in inclined stroke-plane hovering. J Braz. Soc. Mech. Sci. Eng. 41, 347 (2019). https://doi.org/10.1007/s40430-019-1840-6
Received:
Accepted:
Published:
DOI: https://doi.org/10.1007/s40430-019-1840-6