Abstract
Friction stir welding is a manufacturing process with several positive outputs for duplex stainless steel joints, such as improved mechanical resistance and the maintenance of optimal phase fraction. Duplex steels are vastly used in naval and petrochemical operations, being commonly manufactured and joined via welding processes. Thus, there is a high demand for straightforward methods of thermal evaluation of friction stir welded joints. In this sense, numerical models are a practical tool for assessing the joints’ welding condition. However, the vast majority of works concerning numerical modeling of FSW of duplex steels are limited, by some considerations regarding the heat source modeling, by neglecting the material’s flow convective influence, and by omitting the thermomechanical properties of individual phases. This work focused on the development and application of a more complete coupled numerical model for friction stir welding of UNS S32205 plates in order to correlate processing conditions with microstructure evolution. A peak temperature of 1213 °C at the joint’s central line was observed. Distributions of temperature and material flow through the joint cross-section indicate that a more intense material flow at the retreating side favors coarser grain size. Simulation results indicated that the strain rate plays a more intense effect in microstructure development compared to the welding peak temperatures. The coupled numerical model was additionally used to obtain the temperature profile of the tool, which was thermally stable even after standing temperature values greater than 1200 °C. Even after 18 cm of welding procedure, thermal damage of the tool was not observed, leading to sound welded joints.


















Similar content being viewed by others
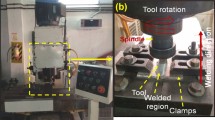
Data availability
All the data generated or analyzed during this study are included in this published article.
References
Charles J (2008) Duplex stainless steels, a review after DSS’07 in Grado. Rev Métallurgie 105:155–171. https://doi.org/10.1051/metal:2008028
Boillot P, Peultier J (2014) Use of stainless steels in the industry: recent and future developments. Procedia Eng 83:309–321. https://doi.org/10.1016/j.proeng.2014.09.015
Chail G, Kangas P (2016) Super and hyper duplex stainless steels: structures, properties and applications. Procedia Struct Integr 2:1755–1762. https://doi.org/10.1016/j.prostr.2016.06.221
Karlson L (2012) Welding duplex stainless steels — a review of current recommendations. Weld World 56:65–76. https://doi.org/10.1007/BF03321351
Lo KH, Shek CH, Lai JKL (2009) Recent developments in stainless steels. Mater Sci Eng R Rep 65:39–104. https://doi.org/10.1016/j.mser.2009.03.001
Michalska J, Sozańska M (2006) Qualitative and quantitative analysis of σ and χ phases in 2205 duplex stainless steel. Mater Charact 56:355–362. https://doi.org/10.1016/j.matchar.2005.11.003
Escriba DM, Materna-Morris E, Plaut RL, Padilha AF (2009) Chi-phase precipitation in a duplex stainless steel. Mater Charact 60:1214–1219. https://doi.org/10.1016/j.matchar.2009.04.013
Llorca-Isern N, López-Luque H, López-Jiménez I, Biezma MV (2016) Identification of sigma and chi phases in duplex stainless steels. Mater Charact 112:20–29. https://doi.org/10.1016/j.matchar.2015.12.004
Santa Cruz LA, Marques IJ, Urtiga Filho SL et al (2019) Corrosion evaluation of duplex and superduplex stainless steel friction stir welds using potentiodynamic measurements and immersion tests in chloride environments. Metallogr Microstruct Anal 8:32–44. https://doi.org/10.1007/s13632-018-0506-6
Pezzato L, Lago M, Brunelli K et al (2018) Effect of the heat treatment on the corrosion resistance of duplex stainless steels. J Mater Eng Perform 27:3859–3868. https://doi.org/10.1007/s11665-018-3408-5
Gennari C, Pezzato L, Piva E et al (2018) Influence of small amount and different morphology of secondary phases on impact toughness of UNS S32205 Duplex Stainless Steel. Mater Sci Eng A 729:149–156. https://doi.org/10.1016/j.msea.2018.05.063
Verma J, Taiwade RV (2017) Effect of welding processes and conditions on the microstructure, mechanical properties and corrosion resistance of duplex stainless steel weldments—a review. J Manuf Process 25:134–152. https://doi.org/10.1016/j.jmapro.2016.11.003
Giorjão RAR, Pereira VF, Terada M et al (2018) Microstructure and mechanical properties of friction stir welded 8mm pipe SAF 2507 super duplex stainless steel. J Mater Res Technol. https://doi.org/10.1016/j.jmrt.2018.01.002
Santos TFA, López EAT, Fonseca EB, Ramirez AJ (2016) Friction stir welding of duplex and superduplex stainless steels and some aspects of microstructural characterization and mechanical performance. Mater Res 19:117–131. https://doi.org/10.1590/1980-5373-MR-2015-0319
Atapour M, Sarlak H, Esmailzadeh M (2016) Pitting corrosion susceptibility of friction stir welded lean duplex stainless steel joints. Int J Adv Manuf Technol 83:721–728. https://doi.org/10.1007/s00170-015-7601-5
Sarlak H, Atapour M, Esmailzadeh M (2015) Corrosion behavior of friction stir welded lean duplex stainless steel. Mater Des 1980–2015(66):209–216. https://doi.org/10.1016/j.matdes.2014.10.060
Esmailzadeh M, Shamanian M, Kermanpur A, Saeid T (2013) Microstructure and mechanical properties of friction stir welded lean duplex stainless steel. Mater Sci Eng A 561:486–491. https://doi.org/10.1016/j.msea.2012.10.068
Thomas WM, Nicholas ED, Needham JC, Murch MG, Temple-Smith P, Dawes CJ (1995) (TWI): friction welding. United States Patent US 005460317A
Ramirez AJ, Hermenegildo TFC, Pereira VF et al (2013) Friction stir welding of steels for the oil and gas industry. In: Proceedings of the 1st international joint symposium on joining and welding. Elsevier. Osaka, Japan, 6–8 November 2013
Liu FC, Hovanski Y, Miles MP et al (2018) A review of friction stir welding of steels: tool, material flow, microstructure, and properties. J Mater Sci Technol 34:39–57. https://doi.org/10.1016/j.jmst.2017.10.024
Cui L, Zhang C, Liu Y et al (2018) Recent progress in friction stir welding tools used for steels. J Iron Steel Res Int 25:477–486. https://doi.org/10.1007/s42243-018-0066-7
Farnoush H, Momeni A, Dehghani K et al (2010) Hot deformation characteristics of 2205 duplex stainless steel based on the behavior of constituent phases. Mater Des 31:220–226. https://doi.org/10.1016/j.matdes.2009.06.028
Xu J, Bernath JJ (2010) Simulation of friction stir welding. In: Furrer DU, Semiatin SL (eds) ASM handbook 22B: metals process simulation. ASM International, pp 443–451. https://doi.org/10.31399/asm.hb.v22b.9781627081979
Nandan R, Roy GG, Lienert TJ, Debroy T (2007) Three-dimensional heat and material flow during friction stir welding of mild steel. Acta Mater 55:883–895. https://doi.org/10.1016/j.actamat.2006.09.009
Matsushita M, Kitani Y, Ikeda R et al (2012) Microstructure and toughness of friction stir weld of thick structural steel. ISIJ Int 52:1335–1341. https://doi.org/10.2355/isijinternational.52.1335
Schmidt H, Hattel J, Wert J (2004) An analytical model for the heat generation in friction stir welding. Model Simul Mater Sci Eng 12:143–157. https://doi.org/10.1088/0965-0393/12/1/013
Nandan R, Balu P, De A, Debroy T (2007) Improving reliability of heat transfer and materials flow calculations during friction stir welding of dissimilar aluminum alloys. Weld J 86:313–322
Sun Z, Wu CS (2018) A numerical model of pin thread effect on material flow and heat generation in shear layer during friction stir welding. J Manuf Process 36:10–21. https://doi.org/10.1016/j.jmapro.2018.09.021
Hernández CA, Ferrer VH, Mancilla JE, Martínez LC (2017) Three-dimensional numerical modeling of the friction stir welding of dissimilar steels. Int J Adv Manuf Technol 93:1567–1581. https://doi.org/10.1007/s00170-017-0578-5
Arafat ME, Al Badour F, Merah N (2021) Fully coupled thermo-mechanical finite element modeling of friction stir processing of super duplex stainless steel. Int J Adv Manuf Technol 112:3485–3500. https://doi.org/10.1007/s00170-020-06574-2
Deng L, Li S, Ke L et al (2019) Microstructure and fracture behavior of refill friction stir spot welded joints of AA2024 using a novel refill technique. Metals 9:286. https://doi.org/10.3390/met9030286
Muci-Küchler KH, Kakarla SST, Arbegast WJ, Allen CD (2005) Numerical simulation of the friction stir spot welding process. In: SAE 2005 World Congress & Exhibition. SAE International. Detroit, Michigan, United States, 11–14 April 2013. https://doi.org/10.4271/2005-01-1260
Santos TFA, Torres EA, Lippold JC, Ramirez AJ (2016) Detailed microstructural characterization and restoration mechanisms of duplex and superduplex stainless steel friction-stir-welded joints. J Mater Eng Perform 25:5173–5188. https://doi.org/10.1007/s11665-016-2357-0
Santos TFDA, Torres EA, Ramirez AJ (2018) Friction stir welding of duplex stainless steels. Weld Int 32:103–111. https://doi.org/10.1080/09507116.2017.1347323
Santa Cruz LA, Machado G, Vicente AA et al (2019) Effect of high anodic polarization on the passive layer properties of superduplex stainless steel friction stir welds at different chloride electrolyte pH values and temperatures. Int J Miner Metall Mater 26:710–721. https://doi.org/10.1007/s12613-019-1790-0
Marques IJ, Silva FJ, Santos TFA (2020) Rapid precipitation of intermetallic phases during isothermal treatment of duplex stainless steel joints produced by friction stir welding. J Alloys Compd 820:153170. https://doi.org/10.1016/j.jallcom.2019.153170
Marques IJ, da Silva FJ, de França TS et al (2019) Evaluation of abrasive wear in UNS S32101 and S32750 duplex stainless steels submitted to friction stir processing. Mater Res 22:e20180877. https://doi.org/10.1590/1980-5373-mr-2018-0877
Santos TFA, Idagawa HS, Ramirez AJ (2014) Thermal history in UNS S32205 duplex stainless steel friction stir welds. Sci Technol Weld Join 19:150–156. https://doi.org/10.1179/1362171813Y.0000000174
Santos TFA, Marinho RR, Paes MTP, Ramirez AJ (2013) Microstructure evaluation of UNS S32205 duplex stainless steel friction stir welds. Rem Rev Esc Minas 66:187–191. https://doi.org/10.1590/S0370-44672013000200008
Cho H-H, Hong S-T, Roh J-H et al (2013) Three-dimensional numerical and experimental investigation on friction stir welding processes of ferritic stainless steel. Acta Mater 61:2649–2661. https://doi.org/10.1016/j.actamat.2013.01.045
Saeid T, Abdollah-zadeh A, Assadi H, Malek Ghaini F (2008) Effect of friction stir welding speed on the microstructure and mechanical properties of a duplex stainless steel. Mater Sci Eng A 496:262–268. https://doi.org/10.1016/j.msea.2008.05.025
da Fonseca EB, Santos TFA, Button ST, Ramirez AJ (2016) Physical simulation of a duplex stainless steel friction stir welding by the numerical and experimental analysis of hot torsion tests. Metall Mater Trans A 47:4543–4552. https://doi.org/10.1007/s11661-016-3631-3
Momeni A, Dehghani K (2011) Hot working behavior of 2205 austenite–ferrite duplex stainless steel characterized by constitutive equations and processing maps. Mater Sci Eng A 528:1448–1454. https://doi.org/10.1016/j.msea.2010.11.020
Emami S, Saeid T, Abdollah-zadeh A (2019) Effect of friction stir welding parameters on the microstructure and microtexture evolution of SAF 2205 stainless steel. J Alloys Compd 810:151797. https://doi.org/10.1016/j.jallcom.2019.151797
Li H, Gao J, Li Q et al (2019) Effect of friction stir welding tool design on welding thermal efficiency. Sci Technol Weld Join 24:156–162. https://doi.org/10.1080/13621718.2018.1495868
Lienert TJ, Stellwag WL Jr, Grimmett BB, Warke RW (2003) Friction stir welding studies on mild steel. Weld J-N Y- 82:1-S
Zhu XK, Chao YJ (2004) Numerical simulation of transient temperature and residual stresses in friction stir welding of 304L stainless steel. J Mater Process Technol 146:263–272. https://doi.org/10.1016/j.jmatprotec.2003.10.025
Colegrove PA, Shercliff HR (2005) 3-Dimensional CFD modelling of flow round a threaded friction stir welding tool profile. J Mater Process Technol 169:320–327. https://doi.org/10.1016/j.jmatprotec.2005.03.015
Carslaw HS, Jaeger JC (1986) Conduction of heat in solids, 2nd edn. Oxford University Press, Oxford
Shi L, Wu CS (2017) Transient model of heat transfer and material flow at different stages of friction stir welding process. J Manuf Process 25:323–339. https://doi.org/10.1016/j.jmapro.2016.11.008
Nandan R, DebRoy T, Bhadeshia H (2008) Recent advances in friction-stir welding–process, weldment structure and properties. Prog Mater Sci 53:980–1023. https://doi.org/10.1016/j.pmatsci.2008.05.001
Manvatkar V, De A, Svensson L-E, DebRoy T (2015) Cooling rates and peak temperatures during friction stir welding of a high-carbon steel. Scr Mater 94:36–39. https://doi.org/10.1016/j.scriptamat.2014.09.012
Schmidt HB, Hattel JH (2008) Thermal modelling of friction stir welding. Scr Mater 58:332–337. https://doi.org/10.1016/j.scriptamat.2007.10.008
Silva ACF, De Backer J, Bolmsjö G (2017) Temperature measurements during friction stir welding. Int J Adv Manuf Technol 88:2899–2908. https://doi.org/10.1007/s00170-016-9007-4
Çam G (2011) Friction stir welded structural materials: beyond Al-alloys. Int Mater Rev 56:1–48. https://doi.org/10.1179/095066010X12777205875750
Mishra RS, Mahoney MW (2007) Friction stir welding and processing. ASM International Materials Park, Ohio
Badji R, Bouabdallah M, Bacroix B et al (2008) Phase transformation and mechanical behavior in annealed 2205 duplex stainless steel welds. Mater Charact 59:447–453. https://doi.org/10.1016/j.matchar.2007.03.004
De A, Bhadeshia HKDH, DebRoy T (2014) Friction stir welding of mild steel: tool durability and steel microstructure. Mater Sci Technol 30:1050–1056. https://doi.org/10.1179/1743284714Y.0000000534
Park SHC, Sato YS, Kokawa H et al (2003) Rapid formation of the sigma phase in 304 stainless steel during friction stir welding. Scr Mater 49:1175–1180. https://doi.org/10.1016/j.scriptamat.2003.08.022
Divinski SV, Geise J, Rabkin E, Chr H (2004) Grain boundary self-diffusion in α-iron of different purity: effect of dislocation enhanced diffusion. Z Für Met 95:945–952. https://doi.org/10.3139/146.018036
Zhang YN, Cao X, Larose S, Wanjara P (2012) Review of tools for friction stir welding and processing. Can Metall Q 51:250–261. https://doi.org/10.1179/1879139512Y.0000000015
Rai R, De A, Bhadeshia HKDH, DebRoy T (2011) Review: friction stir welding tools. Sci Technol Weld Join 16:325–342. https://doi.org/10.1179/1362171811Y.0000000023
Vicharapu B, Liu H, Morisada Y et al (2021) Degradation of nickel-bonded tungsten carbide tools in friction stir welding of high carbon steel. Int J Adv Manuf Technol 115:1049–1061. https://doi.org/10.1007/s00170-021-07159-3
Vicharapu B, Lemos GVB, Bergmann L et al (2021) Probing underlying mechanisms for PCBN tool decay during friction stir welding of nickel-based alloys. Tecnol Metal Mater Min 18:e2455. https://doi.org/10.4322/2176-1523.20202455
Bhadeshia HKDH, DebRoy T (2009) Critical assessment: friction stir welding of steels. Sci Technol Weld Join 14:193–196. https://doi.org/10.1179/136217109X421300
Al-moussawi M, Smith AJ, Faraji M, Cater S (2019) Estimation of the temperature in the stirred zone and cooling rate of friction stir welding of EH46 steel from TiN precipitates. Metall Mater Trans A 50:5103–5116. https://doi.org/10.1007/s11661-019-05383-x
Park SHC, Sato YS, Kokawa H et al (2009) Boride formation induced by pcBN tool wear in friction-stir-welded stainless steels. Metall Mater Trans A 40:625–636. https://doi.org/10.1007/s11661-008-9709-9
Çam G, İpekoğlu G, Küçükömeroğlu T, Aktarer SM (2017) Applicability of friction stir welding to steels. J Achiev Mater Manuf Eng 80:65–85. https://doi.org/10.5604/01.3001.0010.2027
Acknowledgements
The authors thank Brazilian and Portuguese funding agencies—Fundação de Amparo à Ciência e Tecnologia do Estado de Pernambuco (FACEPE), Coordenação de Aperfeiçoamento de Pessoal de Nível Superior (CAPES), Conselho Nacional de Desenvolvimento Científico e Tecnológico (CNPq), Financiadora de Estudos e Projetos (FINEP), Agência Nacional do Petróleo, Gás Natural e Biocombustíveis (ANP/Petrobras). The authors also acknowledge the experimental infrastructure of Laboratório Nacional de Nanotecnologia (LNNano) in Centro Nacional de Pesquisa em Energia e Materiais (CNPEM). TFAS also acknowledges the technical support, collaboration, and mentorship of Prof. Antonio Ramirez (Ohio State University). JPO acknowledges funding by national funds from FCT - Fundação para a Ciência e a Tecnologia, I.P., in the scope of the projects LA/P/0037/2020, UIDP/50025/2020 and UIDB/50025/2020 of the Associate Laboratory Institute of Nanostructures, Nanomodelling and Nanofabrication – i3N. JS acknowledges the China Scholarship Council for funding the Ph.D. grant (CSC NO. 201808320394).
Funding
This work was supported by multiuser funding of Fundação de Amparo à Ciência e Tecnologia do Estado de Pernambuco (FACEPE) under grant number APQ-0964–3.03/21. TFAS also thank Conselho Nacional de Desenvolvimento Científico e Tecnológico (CNPq) for scientific productivity scholarship (grant number 304741/2020–5). JPO and JS acknowledge Fundação para a Ciência e a Tecnologia (FCT—MCTES) for a its financial support via the project UID/00667/2020 (UNIDEMI). JS acknowledges the China Scholarship Council for funding the Ph.D. grant (CSC NO. 201808320394). JPO acknowledges the funding of CENIMAT/i3N by national funds through the FCT-Fundação para a Ciência e a Tecnologia, I.P., within the scope of multiannual financing of R&D units, reference UIDB/50025/2020–2023.
Author information
Authors and Affiliations
Contributions
G. G. S. Figueiredo: contributed to the development of numerical simulation, interpretation of numerical data, graphical planning, and framework.
I. J. Marques: contributed to the development of numerical simulation and microstructure data processing and discussion, the interpretation of numerical data, graphical planning, and a framework.
L. O. Siqueira: contributed to the development of numerical simulation, interpretation of numerical data, graphical planning, and framework.
J. Shen: contributed to the development of numerical simulation, gathered thermomechanical and thermophysical data of UNS S32205, and numerical data interpretation.
J. P. Oliveira: contributed to the development of numerical simulation, gathered thermomechanical and thermophysical data of UNS S32205, and numerical data interpretation.
T.F.A. Santos: contributed to the delimitation of the research topic, conducted experimental procedures of friction stir welding, gathered experimental temperature acquisition, supervised the findings of this work, and contributed to the interpretation of the results.
All the authors contributed to manuscript writing and reviewing.
Corresponding author
Ethics declarations
Ethics approval
Herewith the confirmation: The paper was and is not submitted for publication elsewhere. This paper has not been published elsewhere in its entirety, in part, or in a modified version. The paper was not submitted for possible publication elsewhere.
Consent to participate
Not applicable.
Consent for publication
Not applicable.
Conflict of interest
The authors declare no competing interests.
Additional information
Publisher's note
Springer Nature remains neutral with regard to jurisdictional claims in published maps and institutional affiliations.
The original online version of this article was revised: The information to the commission was missing
Recommended for publication by Commission III - Resistance Welding, Solid State Welding, and Allied Joining Process
Rights and permissions
Springer Nature or its licensor (e.g. a society or other partner) holds exclusive rights to this article under a publishing agreement with the author(s) or other rightsholder(s); author self-archiving of the accepted manuscript version of this article is solely governed by the terms of such publishing agreement and applicable law.
About this article
Cite this article
de Sousa Figueiredo, G.G., Marques, I.J., Siqueira, L.O. et al. A coupled computational fluid dynamics approach as a viable tool for thermal history assessment of UNS S32205 duplex stainless steels friction stir welded joints. Weld World 67, 353–372 (2023). https://doi.org/10.1007/s40194-022-01416-z
Received:
Accepted:
Published:
Issue Date:
DOI: https://doi.org/10.1007/s40194-022-01416-z