Abstract
Purpose
Protoplasmic tubes of Physarum polycephalum, also know as Physarum Wires (PW), have been previously suggested as novel bio-electronic components. Until recently, practical examples of electronic circuits using PWs have been limited. These PWs have been shown to be self repairing, offering significant advantage over traditional electronic components. This article documents work performed to produce practical circuits using PWs.
Methods
We have demonstrated through manufacture and testing of hybrid circuits that PWs can be used to produce a variety of practical electronic circuits. A plurality of different applications of PWs have been tested to show the universality of PWs in analogue and digital electronics.
Results
Voltage dividers can be produced using a pair of PWs in series with an output voltage accurate to within 12%. PWs can also transmit analogue and digital data with a frequency of up to 19 kHz, which with the addition of a buffer, can drive high current circuits. We have demonstrated that PWs can last approximately two months, a 4 fold increase on previous literature. Protoplasmic tubes can be modified with the addition of conductive or magnetic nano-particles to provide changes in functionality.
Conclusions
This work has documented novel macro-scale data transmission through biological material; it has advanced the field of bio-electronics by providing a cheap and easy to grow conducting bio-material which may be used in future hybrid electronic technology.
Similar content being viewed by others
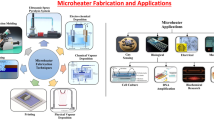
References
Palchetti I, Mascini M. Biosensor technology: a brief history. Sensors and Microsystems. Springer Netherlands; 2010. pp. 15–23.
Renaud S, Catargi B, Lang J. Biosensors in diabetes. IEEE Pulse. 2-14; 5(3): 30-4.
Deutscher L, Renner LD, Cuniberti G. Flagella - templates for the synthesis of metallic nanowires. IFMBE Proc. 2013; 41:860–3.
Magoga M, Joachim C. Conductance and transparence of long molecular wires. Phys Rev B. 1997; 56(8):4722.
Ratner MA, Davis B, Kemp M, Mujica V, Roitberg A, Yaliraki S. Molecular wires: charge transport, mechanisms and control. Ann NY Acad Sci. 1998; 852:22–37.
Tian W, Datta S, Hong S, Reifenberger R, Henderson JI, Kubiak CP. Conductance spectra of molecular wires. J Chem Phys. 1998; 109(7):2874.
Ito Y, Fukusaki E. DNA as a ‘Nanomaterial’. J Mol Catal B Enzym. 2004; 28(4–6):155–66.
Adamatzky A. Towards plant wires. Biosystems. 2014; 122:1–6.
Lovley DR. Live wires: direct extracellular electron exchange for bioenergy and the bioremediation of energy-related contamination. Energy Environ Sci. 2011; 4(12):4896–906.
Reguera G, Nevin KP, Nicoll JS, Covalla SF, Woodard TL, Lovley DR. Biofilm and nanowire production leads to increased current in Geobacter sulfurreducens fuel cells. Appl Environ Microbiol. 2006; 72(11):7345–8.
Stephenson S, Stempen H. Myxomycetes: a handbook of slime molds. Timber Press; 2000.
Ueda T, Terayama K, Kurihara K, Kobatake Y. Threshold phenomena in chemoreception and taxis in slime mold Physarum polycephalum. J Gen Physiol. 1975; 65(2):223–34.
Whiting J, de Lacy Costello B, Adamatzky A. Towards slime mould chemical sensor: mapping chemical inputs onto electrical potential dynamics of Physarum Polycephalum. Sens Actuators B Chem. 2014; 191:844–53.
Adamatzky A. Physarum Machines: Computers from Slime Mould. London: World Scientific Publishing Co.; 2010.
Adamatzky A. Tactile bristle sensors made with slime mold. IEEE Sens J. 2014; 14(2):324–32.
Adamatzky A. Slime mould tactile sensor. Sens Actuators B Chem. 2013; 188:38–44.
Häder DP, Schreckenbach T. Phototactic orientation in plasmodia of the acellular slime mold, Physarum polycephalum. Plant Cell Physiol. 1984; 25(1):55.
Mayne R, Adamatzky A. Slime mould foraging behaviour as optically-coupled logical operations. Int J Gen Syst. 2015; 44(3):305–13.
Nakagaki T, Yamada H, Ueda T. Modulation of cellular rhythm and photoavoidance by oscillatory irradiation in the Physarum plasmodium. Biophys Chem. 1999; 82(1):23–8.
Whiting J, de Lacy Costello B, Adamatzky A. Development and initial testing of a novel slime mould biosensor. Conf Proc IEEE Eng Med Biol Soc. 2014; 4042–5.
Whiting J, de Lacy Costello B, Adamatzky A. Physarum Chip:developments in growing computers from slime mould. UCNC: Unconventional Computation in Europe Workshop. 2014; 3.
Whiting J, de Lacy Costello B, Adamatzky A. Sensory fusion in Physarum polycephalum and implementing multi-sensory functional computation. Biosystems. 2014; 119:45–52.
Whiting J, de Lacy Costello B, Adamatzky A. Slime mould logic gates based on frequency changes of electrical potential oscillation. Biosystems. 2014; 124:21–5.
de Lacy Costello BP, Adamatzky A. Routing of Physarum polycephalum “signals” using simple chemicals. Commun Intergr Biol. 2014; 7(3):e28543.
Nakagaki T, Yamada H. Tóth A. Intelligence: maze-solving by an amoeboid organism. Nature. 2000; 407(6803):470.
Adamatzky A, Jones J. Road planning with slime mould: if Physarum built motorways it would route M6/M74 through Newcastle. Int J Bifurcation Chaos. 2010; 20(10):3065.
Adamatzky A. The world’s colonization and trade routes formation as imitated by slime mould. Int J Bifurcation Chaos. 2012; 22(08):1230028.
Adamatzky A. Physarum wires: self-growing self-repairing smart wires made from slime mould. Biomed Eng Lett. 2013; 3(4):232–41.
Adamatzky A. Slime mould electronic oscillators. Microelectron Eng. 2014; 124:58–65.
Adamatzky AI. Route 20, autobahn 7, and slime mold:approximating the longest roads in USA and Germany with slime mold on 3-D terrains. Cybernetics. IEEE Trans. 2014; 44(1):126–36.
Whiting J, de Lacy Costello B, Adamatzky A. Transfer function of protoplasmic tubes of Physarum polycephalum. Biosystems. 2015; 128:48–51.
Palleau E, Reece S, Desai SC, Smith ME, Dickey MD. Selfhealing stretchable wires for reconfigurable circuit wiring and 3D microfluidics. Adv Mater. 2013; 25(11):1589–92.
Williams KA, Boydston AJ, Bielawski CW. Towards electrically conductive, self-healing materials. J R Soc Interface. 2007; 4(13):359–62.
Mayne R, Adamatzky A. Toward hybrid nanostructure-slime mould devices. Nano Life. 2015. 4:1450007.
Mayne R, Patton D, de Lacy Costello B, Adamatzky A, Patton RC. On the internalisation, intraplasmodial carriage and excretion of metallic nanoparticles in the slime mould, Physarum polycephalum. Int J Nanotechnol Mol Comput. 2013; 3(3):1–14.
Jones J, Whiting J, Adamatzky A. Quantitative transformation for implementation of adder circuits in physical systems. Biosystems. 2015; 134:16–23.
Whiting J, Jones J, Bull L, Levin M, Adamatzky A. Towards a Physarum learning chip. Sci Rep. 2016; 6: 19948.
Daniel JW, Rusch HP. Method for inducing sporulation of pure cultures of the myxomycete Physarum polycephalum. J Bacteriol. 1962; 83:234–40.
Reid CR, Latty T, Dussutour A, Beekman M. Slime mold uses an externalized spatial “memory” to navigate in complex environments. Proc Natl Acad Sci USA. 2012; 109(43):17490–4.
Smith D, Ryan M. Implementing best practices and validation of cryopreservation techniques for microorganisms. Scientific World Journal. 2012; 2012:805659.
Dimonte A, Berzina T, Cifarelli A, Chiesi V, Albertini F, Erokhin V. Conductivity patterning with Physarum polycephalum:natural growth and deflecting. Phys Stat Solidi (C). 2015; 12(1–2):197–201.
Takamatsu A, Takaba E, Takizawa G. Environment-dependent morphology in plasmodium of true slime mold Physarum polycephalum and a network growth model. J Theor Biol. 2009; 256(1):29–44.
Renugopalakrishnan V, Lewis R. Bionanotechnology: Proteins to nanodevices. Springer Netherlands; 2006.
Author information
Authors and Affiliations
Corresponding author
Rights and permissions
About this article
Cite this article
Whiting, J.G.H., Mayne, R., Moody, N. et al. Practical circuits with Physarum Wires. Biomed. Eng. Lett. 6, 57–65 (2016). https://doi.org/10.1007/s13534-016-0212-8
Received:
Revised:
Accepted:
Published:
Issue Date:
DOI: https://doi.org/10.1007/s13534-016-0212-8