Abstract
The research work aims to estimate the bioenergy potential of pistachio shell and study its degradation kinetics which is necessary for the efficient design and optimization of thermochemical processes for bioenergy generation. Initial characterizations (proximate, ultimate, higher heating value, and compositional analysis), kinetic study, and thermodynamic analysis accompanied by reaction mechanism are investigated. Physicochemical characterization results confirmed high volatile matter (~ 79.8 wt%) and high heating value (16.85 MJ/kg) of pistachio shell. Thermogravimetric analysis (TGA) is performed at four different heating rates of 10, 20, 30, and 40 °C/min under nitrogen gas flow rate from ambient temperature to 900 °C. TGA results show the three-stage pyrolysis reaction which involves removal of moisture and light volatiles, degradation of cellulose and hemicellulose, and decomposition of lignin. The result also reveals that maximum degradation occurred in the temperature range of 200–400 °C. For calculating the kinetic (activation energy and pre-exponential factor) and thermodynamic parameters (enthalpy, entropy, and Gibbs free energy), different iso-conversional models, i.e. Flynn-wall-Ozawa (FWO), Kissinger–Akahira–Sunose (KAS), Starink, and Friedman, are employed which gives the average value of activation energy as 168.86, 165.80, 166.29, and 190.10 kJ/mol, respectively and the pre-exponential factor values lie in the range of 107-1021 s−1. The average values of Gibbs free energy calculated for FWO, KAS, Starink, and Friedman methods are 182.09, 182.15, 182.13, and 181.42 kJ/mol, respectively. Criado method and Z plot are showing complex reaction mechanism. The results of kinetics and thermodynamic study reveal pistachio shell is an efficient biomass for bioenergy production.








Similar content being viewed by others
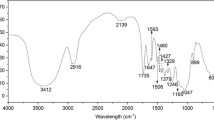
Abbreviations
- HHV:
-
Higher heating value (MJ/kg)
- TGA:
-
Thermogravimetric analysis
- DTG:
-
Differential thermogravimetry
- T :
-
time (s)
- K :
-
Reaction rate constant
- Α :
-
Fractional conversion
- m o :
-
Initial mass of sample (mg)
- m t :
-
Mass of sample at any time t (mg)
- m f :
-
Final mass of sample (mg)
- E a :
-
Activation energy (kJ/mol)
- A :
-
Pre-exponential factor (s−1)
- R :
-
Universal gas constant
- Β :
-
Heating rate (°C/min)
- T :
-
Temperature (K)
- ΔH :
-
Change in enthalpy (kJ/mol)
- ΔG :
-
Change in Gibbs free energy (kJ/mol)
- ΔS :
-
Change in entropy (J/mol K)
- K B :
-
Boltzmann constant (1.381*10−23 J/K)
- h :
-
Plank constant (6.626*10−34 J s)
References
Gupta GK, Mondal MK (2019) Kinetics and thermodynamic analysis of maize cob pyrolysis for its bio energy potential using thermogravimetric analyzer. J Therm Anal Calorim 137:1431–1441
Açıkalın K (2011) Thermogravimetric analysis of walnut shell as pyrolysis feedstock. J Therm Anal Calorim 105:145–150
Olatunji OO, Akinlabi S, Madushele N, Adedeji PA, Ndolomingo MJ (2020) Geospatial investigation of physicochemical properties and thermodynamic parameters of biomass residue for energy generation. Biomass Conv Bioref. https://doi.org/10.1007/s13399-020-00723-z
David E, Kopac J, Armeanu A, Niculescu V, Sandru C, Badescu V (2019) Biomass-alternative renewable energy resource and its conversion for hydrogen rich gas production. E3S Web of Conferences 122
Balat H, Kirtay E (2010) Hydrogen from biomass–present scenario and future prospects. Int J Hydrogen Energy 35:7416–7426
Dufour A, Girods P, Masson E, Rogaume Y, Zoulalian A (2009) Synthesis gas production by biomass pyrolysis: effect of reactor temperature on product distribution. Int J Hydrogen Energy 34:1726–1734
Panwar NL, Kothari R, Tyagi VV (2012) Thermochemical conversion of biomass–ecofriendly energy routes. Renew Sust Energ Rev 16:1801–1816
Zhang X, Xu M, Sun L (2004) Study on biomass pyrolysis kinetics. J Eng Gas Turbines Power 128(3):493–496
Soetardji JP, Widjaja C, Djojorahardjo Y, Soetaredjo FE, Ismadji S (2014) Bio oil from jackfruit peel waste. Procedia Chem 9:158–164
Jahirul MI, Rasul MG, Chowdhury AA, Ashwath N (2012) Biofuel production through biomass pyrolysis–a technological review. Energies 5:4952–5001
Couce AA (2016) Reaction mechanisms and multi scale modeling of lignocellulosic biomass pyrolysis. Prog Energy Combust Sci 53:41–79
Mumbach GD, Alves JLF, Gomes da Silva JC, Domenico MD, Felix de Sena R, Marangoni C, Machado RAF, Bolzan A (2020) Pyrolysis of cocoa shell and its bioenergy potential: evaluating the kinetic triplet, thermodynamic parameters, and evolved gas analysis using TGA-FTIR. Biomass Conv Bioref. https://doi.org/10.1007/s13399-020-01058-5
Ahmad MS, Mehmood MA, Taqvi S, Elkamel A, Liu CG, Xu J, Rahimuddin S, Gull M (2017) Pyrolysis, kinetic analysis, thermodynamic parameters and reaction mechanism of Typha Latifolia to evaluate its bioenergy potential. Bioresour Technol 245:491–501
Rasool T, Srivastava VC, Khan MNS (2018) Kinetic and thermodynamic analysis of thermal decomposition of Deodar sawdust and rice husk as potential for pyrolysis. Int J Chem React Eng 17
Slopiecka K, Bartocci P, Fantozzi F (2012) Thermogravimetric analysis and kinetic study of poplar wood pyrolysis. Appl Energy 97:491–497
Rodriguez RA, Mazza G, Echegaray M, Fernandez A, Garcia DZ (2018) Thermodynamic and kinetic study of lignocellulosic waste gasification. IntechOpen
Mahani NM, Oftadeh M (2011) Kinetic and thermodynamic study of activated carbon from pistachio shell by thermogravimetric analysis. J Mater Sci Eng 1:887–891
Nejad ASM (2012) Global pistachio production and marketing challenges in Iran. Acta Hortic (963):133–141
Ghasemynasabparizi M, Ahmadi A, Mazloomi SM (2015) A review on pistachio: its composition and benefits regarding the prevention or treatment of diseases. JOHE 4:57–69
Isitan S, Ceylan S, Topcu Y, Hintz C, Tefft J, Chellappa T, Guo J, Goldfarb JL (2016) Product quality optimization in an integrated biorefinery: conversion of pistachio nutshell biomass to biofuels and activated biochars via pyrolysis. Energy Convers Manag 127:576–588
Açıkalın K, Karaca F, Bolat E (2012) Pyrolysis of pistachio shell: effects of pyrolysis conditions and analysis of products. Fuel 95:169–177
Varol EA, Putun E, Putun AE (2007) Slow pyrolysis of pistachio shell. Fuel 86:1892–1899
Açıkalın K (2012) Pyrolytic characteristics and kinetics of pistachio shell by thermogravimetric analysis. J Therm Anal Calorim 109:227–235
Silva JC, Alves JL, Galdino WV, Moreira R, Jose HJ, Sena RF, Andersen SL (2018) Combustion of pistachio shell: physicochemical characterization and evaluation of kinetic parameters. Environ Sci Pollut Res
Singh S, Chakraborty JP, Mondal MK (2020) Intrinsic kinetics, thermodynamic parameters and reaction mechanism of non-isothermal degradation of torrefied Acacia Nilotica using iso conversional methods. Fuel 259:116263
Zawawi AZ, Gaik LP, Sebran NH, Othman J, Hussain AS (2018) An optimization study on biomass delignification process using alkaline wash. Biomass Conv Bioref 8:59–68
Gu X, Liu C, Jiang X, Ma X, Li L, Cheng K, Li Z (2014) Thermal behaviour and kinetics of the pyrolysis of the raw/steam exploded poplar wood sawdust. J Anal Appl Pyrolysis 106:177–186
Kaur R, Gera P, Jha MK, Bhaskar T (2018) Pyrolysis kinetics and thermodynamic parameters of castor (Ricinus communis) residue using thermogravimetric analysis. Bioresour Technol 250:422–428
Cai J, Xu D, Dong Z, Yu X, Yang Y, Banks SW, Bridgwater AV (2018) Processing thermogravimetric analysis data for isoconversional kinetic analysis of lignocellulosic biomass pyrolysis: case study of corn stalk. Renew Sust Energ Rev 82:2705–2715
Aboyade AO, Hugo TJ, Carrier M, Meyer EL, Stahl R, Knoetze JH, Gorgens JF (2011) Non-isothermal kinetic analysis of the devolatilization of corn cobs and sugarcane bagasse in an inert atmosphere. Thermochim Acta 517:81–89
Rony AS, Kong L, Lu W, Dejam M, Adidharma H, Gasem KAM, Zheng Y, Norton U, Fan M (2019) Kinetics, thermodynamics and physical characterization of corn stover (Zea mays) for solar biomass pyrolysis potential analysis. Bioresour Technol 284:466–473
Ahmad MS, Mehmood MA, Liu CG, Tawab A, Bai FW, Sakdaronnarong C, Xu J, Rahimuddin SA, Gull M (2018) Bioenergy potential of Wolffia arrhiza appraised through pyrolysis, kinetics, thermodynamic parameters and TG-FTIR-MS study of the evolved gases. Bioresour Technol 253:297–303
Raj T, Kapoor M, Gaur R, Christopher J, Lamba B, Tuli DK, Kumar R (2015) Physical and chemical characterization of various Indian agriculture residues for biofuels production. Energy Fuel 29:3111–3118
Shen D, Jin W, Hu J, Xiao R, Luo K (2015) An overview on fast pyrolysis of the main constituents in lignocellulosic biomass to value-added chemicals: structures, pathways and interactions. Renew Sust Energ Rev 51:761–774
Nanda S, Mohanty P, Kozinski JA, Dalai AK (2014) Physico-chemical properties of bio-oils from pyrolysis of lignocellulosic biomass with high and slow heating rate. Energy Environ Res 4:569–577
Cai J, He Y, Yu X, Banks SW, Yang Y, Zhang X, Yu Y, Liu R, Bridgwater AV (2017) Review of physicochemical properties and analytical characterization of lignocellulosic biomass. Renew Sust Energ Rev 76:309–322
Kebelmann K, Hornung A, Karsten U, Griffiths G (2013) Thermochemical behaviour and chemical product formation from Polar seaweeds during intermediate pyrolysis. J Anal Appl Pyrolysis 104:131–138
El-Sayed SA, Mostafa ME (2015) Kinetic parameters determination of biomass pyrolysis fuels using TGA and DTA techniques. Waste Biomass Valor 6:401–415
Salema AA, Ting RMW, Shang YK (2019) Pyrolysis of blend (oil palm biomass and sawdust) biomass using TG-MS. Bioresour Technol 274:439–446
Sharma A, Pareek V, Wang S, Zhang Z, Yang H, Zhang D (2014) A phenomenological model of the mechanisms of lignocellulosic biomass pyrolysis processes. Comput Chem Eng 60:231–241
Babiker ME, Aziz AR, Heikal M, Yusup S, Abakar M (2013) Pyrolysis characteristics of Phoenix Dactylifera Date Palm seeds using thermogravimetric analysis. Int J Environ Sci Dev 4:521–524
Sun Z, Xu B, Rony AH, Toan S, Chen S, Gasem KAM, Adidharma H, Fan M, Xiang W (2017) Thermogravimetric and kinetics investigation of pine wood pyrolysis catalyzed with alkali treated CaO/ZSM-5. Energy Convers Manag 146:182–194
Shen D, Xiao R, Gu S, Luo K (2011) The pyrolytic behaviour of cellulose in lignicellulosic biomass: a review. RSC Adv 1:1641–1660
Varma AK, Mondal P (2016) Physicochemical characterization and pyrolysis kinetics of wood sawdust. Energy Sources A 38:2536–2544
Barrie PJ (2012a) The mathematical origins of the kinetic compensation effect: 1. the effect of random experimental errors. Phys Chem Chem Phys 14:318–326
Barrie PJ (2012b) The mathematical origins of the kinetic compensation effect: 2. the effect of systematic errors. Phys Chem Chem Phys 14:327–336
Wang S, Dai G, Yang H, Luo Z (2017) Lignocellulosic biomass pyrolysis mechanism: a state-of-the-art review. Prog Energy Combust Sci 62:33–86
Millan LMR, Vargas FES, Nzihou A (2017) Kinetic analysis of tropical lignocellulosic agrowaste pyrolysis. Bioenergy Res 10:832–845
Doddapaneni TRKC, Konttinen J, Hukka TI, Moilanen A (2016) Influence of torrefaction pretreatment on the pyrolysis of Eucalyptus clone: a study on kinetics, reaction mechanism and heat flow. Ind Crop Prod 92:244–254
Poletto M, Zattera AJ, Santana RMC (2012) Thermal decomposition of wood: kinetics and degradation mechanisms. Bioresour Technol 126:7–12
Vlaev LT, Markovska LG, Lyubchev LA (2003) Non-isothermal kinetics of pyrolysis of rice husk. Thermochim Acta 406(1):1–7
Alves JLF, Gomes da Silva JC, Domenico MD, Galdino WVDA, Andersen SLF, Alves RF, Felix De Sena R (2020) Exploring Açaí Seed (Euterpe oleracea) pyrolysis using multi-component kinetics and thermodynamics assessment towards its bioenergy potential. Biomass Conv Bioref. https://doi.org/10.1007/s12155-020-10175-y
Alves JLF, Gomes da Silva JC, Languer MP, Batistella L, Domenico MD, Filho VFS, Moreira RFPM, Jose HJ (2020) Assessing the bioenergy potential of high-ash anaerobic sewage sludge using pyrolysis kinetics and thermodynamics to design a sustainable integrated biorefinery. Biomass Conv Bioref. https://doi.org/10.1007/s13399020-01023-2
Acknowledgments
The authors are extremely obliged to the Chemical Engineering and Technology department and Central Instrument Facility Centre, Indian Institute of Technology, BHU, Varanasi for imparting amenities towards the completion of this research study.
Author information
Authors and Affiliations
Corresponding author
Additional information
Publisher’s Note
Springer Nature remains neutral with regard to jurisdictional claims in published maps and institutional affiliations.
Rights and permissions
About this article
Cite this article
Gupta, S., Gupta, G.K. & Mondal, M.K. Thermal degradation characteristics, kinetics, thermodynamic, and reaction mechanism analysis of pistachio shell pyrolysis for its bioenergy potential. Biomass Conv. Bioref. 12, 4847–4861 (2022). https://doi.org/10.1007/s13399-020-01104-2
Received:
Revised:
Accepted:
Published:
Issue Date:
DOI: https://doi.org/10.1007/s13399-020-01104-2