Abstract
The reverse electrodialysis reactor (REDR) degrades wastewater by coupling with different treatment methods. In the paper, the anodic oxidation (AO) and electro-Fenton (EF) processes were adopted in REDR. And the three different anodes, ruthenium and iridium dioxide (Ti/RuO2-IrO2), lead dioxide (Ti/PbO2) and boron-doped diamond (BDD) were used as the anode in REDR. The influences of working fluids velocity, electrode rinse solution (ERS) flow rate on degradation performance under different anodes were determined and discussed. The results indicated that increasing the working fluid velocity and ERS flow rate can improve COD removal efficiency. And the Ti/PbO2 presented a greater degradation effect than other electrodes. Moreover, the paper investigated the effect of operating conditions on total current efficiency (TCE) and the energy consumption (EC) of REDR when three anodes were employed. The results showed the growth of ERS flow rate could significantly enhance the TCE. However, the increasing of working fluids velocity and ERS flow rate also enhances the EC of the system. Meanwhile, among the three anodes, the value of TCE could reach the largest when the BDD anode was adopted in REDR under the same output current. Furthermore, the system presented excellent economic efficiency compared with other traditional oxidation processes.
Graphical Abstract







Similar content being viewed by others
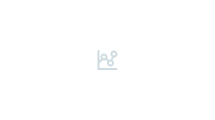
Data Availability Statement
The authors confirm that the data supporting the findings of this study are available within the article.
References
C. Trellu, E. Mousset, Y. Pechaud, D. Huguenot, E.D. van Hullebusch, G. Esposito et al., Removal of hydrophobic organic pollutants from soil washing/flushing solutions: A critical review. J. Hazard. Mater. 306, 149–174 (2016). https://doi.org/10.1016/j.jhazmat.2015.12.008
N. Wang, T. Zheng, G. Zhang, P. Wang, A review on Fenton-like processes for organic wastewater treatment. J. Environ. Chem. Eng. 4, 762–787 (2016). https://doi.org/10.1016/j.jece.2015.12.016
D.A. Yaseen, M. Scholz, Textile dye wastewater characteristics and constituents of synthetic effluents: a critical review. Int. J. Environ. Sci. Technol. 16, 1193–1226 (2019). https://doi.org/10.1007/s13762-018-2130-z
F.C. Moreira, R.A.R. Boaventura, E. Brillas, V.J.P. Vilar, Electrochemical advanced oxidation processes: A review on their application to synthetic and real wastewaters. Appl. Catal. B 202, 217–261 (2017). https://doi.org/10.1016/j.apcatb.2016.08.037
I. Sires, E. Brillas, M.A. Oturan, M.A. Rodrigo, M. Panizza, Electrochemical advanced oxidation processes: today and tomorrow. A review. Environ. Sci. Pollut. Res. 21, 8336–8367 (2014). https://doi.org/10.1007/s11356-014-2783-1
S. Garcia-Segura, J.D. Ocon, M.N. Chong, Electrochemical oxidation remediation of real wastewater effluents - A review. Process Saf. Environ. Prot. 113, 48–67 (2018). https://doi.org/10.1016/j.psep.2017.09.014
A.R. Lado Ribeiro, N.F.F. Moreira, G.L. Puma, A.M.T. Silva, Impact of water matrix on the removal of micropollutants by advanced oxidation technologies. Chem. Eng. J. 363, 155–173 (2019). https://doi.org/10.1016/j.cej.2019.01.080
S.O. Ganiyu, C.A. Martinez-Huitle, M.A. Rodrigo, Renewable energies driven electrochemical wastewater/soil decontamination technologies: A critical review of fundamental concepts and applications. Appl. Catal. B 270, (2020). https://doi.org/10.1016/j.apcatb.2020.118857
X. Luo, X. Cao, Y. Mo, K. Xiao, X. Zhang, P. Liang et al., Power generation by coupling reverse electrodialysis and ammonium bicarbonate: Implication for recovery of waste heat. Electrochem. Commun. 19, 25–28 (2012). https://doi.org/10.1016/j.elecom.2012.03.004
P. Palenzuela, M. Micari, B. Ortega-Delgado, F. Giacalone, G. Zaragoza, D.-C. Alarcon-Padilla et al., Performance Analysis of a RED-MED Salinity Gradient Heat Engine. Energies 11, (2018). https://doi.org/10.3390/en11123385
R. Long, B. Li, Z. Liu, W. Liu, Hybrid membrane distillation-reverse electrodialysis electricity generation system to harvest low-grade thermal energy. J. Membr. Sci. 525, 107–115 (2017). https://doi.org/10.1016/j.memsci.2016.10.035
F. Zhang, S. Xu, D. Feng, S. Chen, R. Du, C. Su et al., A low-temperature multi-effect desalination system powered by the cooling water of a diesel engine. Desalination 404, 112–120 (2017). https://doi.org/10.1016/j.desal.2016.11.006
A. Al-Othman, M. Tawalbeh, M.E. Assad, T. Alkayyali, A. Eisa, Novel multi-stage flash (MSF) desalination plant driven by parabolic trough collectors and a solar pond: A simulation study in UAE. Desalination 443, 237–244 (2018). https://doi.org/10.1016/j.desal.2018.06.005
D. Gonzalez, J. Amigo, F. Suarez, Membrane distillation: Perspectives for sustainable and improved desalination. Renewable Sustainable Energy Rev. 80, 238–259 (2017). https://doi.org/10.1016/j.rser.2017.05.078
O. Scialdone, A. D’Angelo, E. De Lume, A. Galia, Cathodic reduction of hexavalent chromium coupled with electricity generation achieved by reverse-electrodialysis processes using salinity gradients. Electrochim. Acta 137, 258–265 (2014). https://doi.org/10.1016/j.electacta.2014.06.007
O. Scialdone, A. D’Angelo, A. Galia, Energy generation and abatement of Acid Orange 7 in reverse electrodialysis cells using salinity gradients. J. Electroanal. Chem. 738, 61–68 (2015). https://doi.org/10.1016/j.jelechem.2014.11.024
Y. Zhou, K. Zhao, C. Hu, H. Liu, Y. Wang, J. Qu, Electrochemical oxidation of ammonia accompanied with electricity generation based on reverse electrodialysis. Electrochim. Acta 269, 128–135 (2018). https://doi.org/10.1016/j.electacta.2018.02.136
P.F. Ma, X.G. Hao, A. Galia, O. Scialdone, Development of a process for the treatment of synthetic wastewater without energy inputs using the salinity gradient of wastewaters and a reverse electrodialysis stack. Chemosphere 248, 8 (2020). https://doi.org/10.1016/j.chemosphere.2020.125994
S. Xu, Q. Leng, D. Jin, X. Wu, Z. Xu, P. Wang et al., Experimental investigation on dye wastewater treatment with reverse electrodialysis reactor powered by salinity gradient energy. Desalination 495, (2020). https://doi.org/10.1016/j.desal.2020.114541
S. Xu, Q. Leng, X. Wu, Z. Xu, J. Hu, D. Wu et al., Influence of output current on decolorization efficiency of azo dye wastewater by a series system with multi-stage reverse electrodialysis reactors. Energy Convers. Manage. 228, (2021). https://doi.org/10.1016/j.enconman.2020.113639
G.R. Salazar-Banda, G.D.S. Santos, I.M.D. Gonzaga, A.R. Doria, K.I.B. Eguiluz, Developments in electrode materials for wastewater treatment. Curr. Opin. Electrochem. 26, (2021). https://doi.org/10.1016/j.coelec.2020.100663
G.R.P. Malpass, A.D. Motheo, Recent advances on the use of active anodes in environmental electrochemistry. Curr. Opin. Electrochem. 27, (2021). https://doi.org/10.1016/j.coelec.2021.100689
Z.Z. Hu, J.J. Cai, G. Song, Y.S. Tian, M.H. Zhou, Anodic oxidation of organic pollutants: Anode fabrication, process hybrid and environmental applications. Curr. Opin. Electrochem. 26, (2021). https://doi.org/10.1016/j.coelec.2020.100659
I. Sires, E. Brillas, Upgrading and expanding the electro-Fenton and related processes. Curr. Opin. Electrochem. 27, (2021). https://doi.org/10.1016/j.coelec.2020.100686
L. Labiadh, A. Barbucci, M.P. Carpanese, A. Gadri, S. Ammar, M. Panizza, Comparative depollution of Methyl Orange aqueous solutions by electrochemical incineration using TiRuSnO2, BDD and PbO2 as high oxidation power anodes. J. Electroanal. Chem. 766, 94–99 (2016). https://doi.org/10.1016/j.jelechem.2016.01.036
E.B. Cavalcanti, S.G. Segura, F. Centellas, E. Brillas, Electrochemical incineration of omeprazole in neutral aqueous medium using a platinum or boron-doped diamond anode: Degradation kinetics and oxidation products. Water Res. 47, 1803–1815 (2013). https://doi.org/10.1016/j.watres.2013.01.002
M. Malakootian, A. Moridi, Efficiency of electro-Fenton process in removing Acid Red 18 dye from aqueous solutions. Process Saf. Environ. Prot. 111, 138–147 (2017). https://doi.org/10.1016/j.psep.2017.06.008
S. Qiu, D. He, J.X. Ma, T.X. Liu, T.D. Waite, Kinetic Modeling of the Electro-Fenton Process: Quantification of Reactive Oxygen Species Generation. Electrochim. Acta 176, 51–58 (2015). https://doi.org/10.1016/j.electacta.2015.06.103
J.H. Han, H. Kim, K.S. Hwang, N. Jeong, C.S. Kim, Hydrogen Production from Water Electrolysis Driven by High Membrane Voltage of Reverse Electrodialysis. J. Electrochem. Sci. Technol. 10, 302–312 (2019). https://doi.org/10.33961/jecst.2019.03160
C.A. Martinez-Huitle, E.V. dos Santos, D.M. de Araujo, M. Panizza, Applicability of diamond electrode/anode to the electrochemical treatment of a real textile effluent. J. Electroanal. Chem. 674, 103–107 (2012). https://doi.org/10.1016/j.jelechem.2012.02.005
E. Mousset, Y. Pechaud, N. Oturan, M.A. Oturan, Charge transfer/mass transport competition in advanced hybrid electrocatalytic wastewater treatment: Development of a new current efficiency relation. Appl. Catal. B 240, 102–111 (2019). https://doi.org/10.1016/j.apcatb.2018.08.055
E. Tsantaki, T. Velegraki, A. Katsaounis, D. Mantzavinos, Anodic oxidation of textile dyehouse effluents on boron-doped diamond electrode. J. Hazard. Mater. 207, 91–96 (2012). https://doi.org/10.1016/j.jhazmat.2011.03.107
N. Abdessamad, H. Akrout, G. Hamdaoui, K. Elghniji, M. Ksibi, L. Bousselmi, Evaluation of the efficiency of monopolar and bipolar BDD electrodes for electrochemical oxidation of anthraquinone textile synthetic effluent for reuse. Chemosphere 93, 1309–1316 (2013). https://doi.org/10.1016/j.chemosphere.2013.07.011
N. Mohammadi, H. Khani, V.K. Gupta, E. Amereh, S. Agarwal, Adsorption process of methyl orange dye onto mesoporous carbon material-kinetic and thermodynamic studies. J. Colloid Interface Sci. 362, 457–462 (2011). https://doi.org/10.1016/j.jcis.2011.06.067
Y. Xiao, J.M. Hill, Mechanistic insights for the electro-Fenton regeneration of carbon materials saturated with methyl orange: Dominance of electrodesorption. J. Hazard. Mater. 367, 59–67 (2019). https://doi.org/10.1016/j.jhazmat.2018.12.066
M. Tedesco, A. Cipollina, A. Tamburini, G. Micale, Towards 1 kW power production in a reverse electrodialysis pilot plant with saline waters and concentrated brines. J. Membr. Sci. 522, 226–236 (2017). https://doi.org/10.1016/j.memsci.2016.09.015
J. Hu, S. Xu, X. Wu, Multi-stage reverse electrodialysis: Strategies to harvest salinity gradient energy. Energy Convers. Manage. 803–815 (2019)
M. Tedesco, E. Brauns, A. Cipollina, G. Micale, P. Modica, G. Russo et al., Reverse electrodialysis with saline waters and concentrated brines: A laboratory investigation towards technology scale-up. J. Membr. Sci. 492, 9–20 (2015). https://doi.org/10.1016/j.memsci.2015.05.020
O.M. Rodriguez-Narvaez, A.R. Picos, N. Bravo-Yumi, M. Pacheco-Alvarez, C.A. Martinez-Huitle, J.M. Peralta-Hernandez, Electrochemical oxidation technology to treat textile wastewaters. Curr. Opin. Electrochem. 29, (2021). https://doi.org/10.1016/j.coelec.2021.100806
B. Ramirez, V. Rondan, L. Ortiz-Hernandez, S. Silva-Martinez, A. Alvarez-Gallegos, Semi-empirical chemical model for indirect advanced oxidation of Acid Orange 7 using an unmodified carbon fabric cathode for H2O2 production in an electrochemical reactor. J. Environ. Manage. 171, 29–34 (2016). https://doi.org/10.1016/j.jenvman.2016.02.004
Acknowledgements
This project was supported by the National Natural Science Foundations of China (NSFC, No. 51776029 and No.52076026).
Funding
National Natural Science Foundation of China, 51776029, Shiming Xu, 52076026, Xi Wu
Author information
Authors and Affiliations
Corresponding author
Ethics declarations
Conflict of Interest
The authors declare that there are no known competing financial interests or personal relationships that could have appeared to influence the work reported in this paper.
Additional information
Publisher's Note
Springer Nature remains neutral with regard to jurisdictional claims in published maps and institutional affiliations.
Rights and permissions
About this article
Cite this article
Leng, Q., Xu, S., Wu, X. et al. Degrade Methyl Orange by a Reverse Electrodialysis Reactor Coupled with Electrochemical Direct Oxidation and Electro-Fenton Processes. Electrocatalysis 13, 242–254 (2022). https://doi.org/10.1007/s12678-022-00712-y
Accepted:
Published:
Issue Date:
DOI: https://doi.org/10.1007/s12678-022-00712-y