Abstract
There is an ongoing debate regarding whether gliomas originate due to functional or genetic changes in neural stem cells (NSCs). Genetic engineering has made it possible to use NSCs to establish glioma models with the pathological features of human tumors. Here, we found that RAS, TERT, and p53 mutations or abnormal expression were associated with the occurrence of glioma in the mouse tumor transplantation model. Moreover, EZH2 palmitoylation mediated by ZDHHC5 played a significant role in this malignant transformation. EZH2 palmitoylation activates H3K27me3, which in turn decreases miR-1275, increases glial fibrillary acidic protein (GFAP) expression, and weakens the binding of DNA methyltransferase 3A (DNMT3A) to the OCT4 promoter region. Thus, these findings are significant because RAS, TERT, and p53 oncogenes in human neural stem cells are conducive to a fully malignant and rapid transformation, suggesting that gene changes and specific combinations of susceptible cell types are important factors in determining the occurrence of gliomas.





Similar content being viewed by others
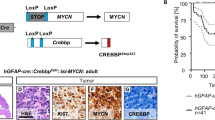
Data Availability
All data generated or analyzed during this study are included within this article.
Abbreviations
- ABE:
-
Acyl–biotin exchange assay
- CDKN1B:
-
Cyclin-dependent kinase inhibitor 1 B
- ChIP:
-
Chromatin immunoprecipitation
- CNS:
-
Central nervous system
- DNMT:
-
DNA methyltransferase
- FACS:
-
Fluorescence activating cell sorter
- HOXA5:
-
Homeobox A5
- MHC:
-
Major histocompatibility complex
- NF1:
-
Neurofibrin 1
- NSC:
-
Neural stem cell
- PBMCs:
-
Peripheral blood mononuclear cells
- RUNX3:
-
Runt-related transcription factor 3
- RT-PCR:
-
Real-time polymerase chain reaction
- TERT:
-
Telomerase reverse transcriptase
- ZDHHC:
-
Zinc finger DHHC domain-containing protein
References
Molinaro AM, Taylor JW, Wiencke JK, Wrensch MR (2019) Genetic and molecular epidemiology of adult diffuse glioma. Nat Rev Neurol 15(7):405–417
Kristensen BW, Priesterbach-Ackley LP, Petersen JK, Wesseling P (2019) Molecular pathology of tumors of the central nervous system. Ann Oncol 30(8):1265–1278
Delgado-Martín B, Medina M (2020) Advances in the knowledge of the molecular biology of glioblastoma and its impact in patient diagnosis, stratification, and treatment. Adv Sci (Weinh) 7(9):1902971
McFaline-Figueroa JR, Lee EQ (2018) Brain tumors. Am J Med 131(8):874–882
Lapointe S, Perry A, Butowski NA (2018) Primary brain tumours in adults. Lancet 392(10145):432–446
Brien GL, Bressan RB, Monger C, Gannon D, Lagan E, Doherty AM, Healy E, Neikes H et al (2021) Simultaneous disruption of PRC2 and enhancer function underlies histone H3.3-K27M oncogenic activity in human hindbrain neural stem cells. Nat Genet 53(8):1221–1232
Silverman DA, Martinez VK, Dougherty PM, Myers JN, Calin GA, Amit M (2021) Cancer-associated neurogenesis and nerve-cancer cross-talk. Cancer Res 81(6):1431–1440
Sutcliffe MD, Galvao RP, Wang L, Kim J, Rosenfeld LK, Singh S, Zong H, Janes KA (2021) Premalignant oligodendrocyte precursor cells stall in a heterogeneous state of replication stress prior to gliomagenesis. Cancer Res 81(7):1868–1882
Eriksson PS, Perfilieva E, Björk-Eriksson T, Alborn AM, Nordborg C, Peterson DA, Gage FH (1998) Neurogenesis in the adult human hippocampus. Nat Med 4(11):1313–1317
Gage FH (2000) Mammalian neural stem cells. Science 287(5457):1433–1438
Sanai N, Tramontin AD, Quiñones-Hinojosa A, Barbaro NM, Gupta N, Kunwar S (2004) Unique astrocyte ribbon in adult human brain contains neural stem cells but lacks chain migration. Nature 427:740–744
Alcantara Llaguno S, Chen J, Kwon CH, Jackson EL, Li Y, Burns DK, Alvarez-Buylla A, Parada LF (2009) Malignant astrocytomas originate from neural stem/progenitor cells in a somatic tumor suppressor mouse model. Cancer Cel 15(1):45–56
Muñoz DM, Tung T, Agnihotri S, Singh S, Guha A, Zadeh G (2013) Loss of p53 cooperates with K-ras activation to induce glioma formation in a region-independent manner. Glia 61(11):1862–1872
Liu C, Sage JC, Miller MR, Verhaak RG, Hippenmeyer S, Vogel H, Foreman O, Bronson RT et al (2011) Mosaic analysis with double markers reveals tumor cell of origin in glioma. Cell 146(2):209–221
Hambardzumyan D, Cheng YK, Haeno H, Holland EC, Michor F (2011) The probable cell of origin of NF1- and PDGF-driven glioblastomas. PLoS One 6(9):e24454
Fan X, Yang H, Zhao C, Hu L, Wang D, Wang R, Fang Z, Chen X (2021) Local anesthetics impair the growth and self-renewal of glioblastoma stem cells by inhibiting ZDHHC15-mediated GP130 palmitoylation. Stem Cell Res Ther 12(1):107
Chen X, Li H, Fan X, Zhao C, Ye K, Zhao Z, Hu L, Ma H et al (2020) Protein palmitoylation regulates cell survival by modulating XBP1 activity in glioblastoma multiforme. Mol Ther Oncolytics 17:518–530
Liu MC, Oxnard GR, Klein EA, Swanton C, Seiden MV (2020) Sensitive and specific multi-cancer detection and localization using methylation signatures in cell-free DNA. Ann Onco 31(6):745–759
Baum C, Lin YC, Fomenkov A, Anton BP, Chen L, Yan B (2021) Rapid identification of methylase specificity (RIMS-seq) jointly identifies methylated motifs and generates shotgun sequencing of bacterial genomes. Nucleic Acids Res 49(19):e113
Barthel L, Hadamitzky M, Dammann P, Schedlowski M, Sure U, Thakur BK, Hetze S (2022) Glioma: molecular signature and crossroads with tumor microenvironment. Cancer Metastasis Rev 41(1):53–75
Shamdani S, Uzan G, Naserian S (2020) TNFα-TNFR2 signaling pathway in control of the neural stem/progenitor cell immunosuppressive effect: different experimental approaches to assess this hypothetical mechanism behind their immunological function. Stem Cell Res Ther 11(1):307
Ko PJ, Dixon SJ (2018) Protein palmitoylation and cancer. EMBO Rep 19(10):e46666
Dietrich S, Hüllein J, Lee SC, Hutter B, Gonzalez D, Jayne S (2015) Recurrent CDKN1B (p27) mutations in hairy cell leukemia. Blood 126(8):1005–1008
Hoxha S, Shepard A, Troutman S, Diao H, Doherty JR, Janiszewska M, Witwicki RM, Pipkin ME et al (2020) YAP-mediated recruitment of YY1 and EZH2 represses transcription of key cell-cycle regulators. Cancer Res 80(12):2512–2522
Ma L, Lin K, Chang G, Chen Y, Yue C, Guo Q, Zhang S, Jia Z et al (2019) Aberrant activation of β-catenin signaling drives glioma tumorigenesis via USP1-mediated stabilization of EZH2. Cancer Res 79(1):72–85
Mai J, Gu J, Liu Y, Liu X, Sai K, Chen Z, Lu W, Yang X et al (2019) Negative regulation of miR-1275 by H3K27me3 is critical for glial induction of glioblastoma cells. Mol Onco 13(7):1589–1604
Mai J, Gu J, Liu Y, Liu X, Sai K, Chen Z, Lu W, Yang X et al (2019) Negative regulation of miR-1275 by H3K27me3 is critical for glial induction of glioblastoma cells. Mol Oncol 13(7):1589–1604
Singh SK, Clarke ID, Hide T, Dirks PB (2004) Cancer stem cells in nervous system tumors. Oncogene 23(43):7267–7273
Havrda MC, Paolella BR, Ran C, Jering KS, Wray CM, Sullivan JM, Nailor A, Hitoshi Y et al (2014) Id2 mediates oligodendrocyte precursor cell maturation arrest and is tumorigenic in a PDGF-rich microenvironment. Cancer Res 74(6):1822–1832
Zhu Y, Guignard F, Zhao D, Liu L, Burns DK, Mason RP, Messing A, Parada LF (2005) Early inactivation of p53 tumor suppressor gene cooperating with NF1 loss induces malignant astrocytoma. Cancer Cell 8(2):119–130
Kadiyala P, Carney SV, Gauss JC, Garcia-Fabiani MB, Haase S, Alghamri MS, Nunez FJ, Liu Y et al (2021) Inhibition of 2-hydroxyglutarate elicits metabolic reprogramming and mutant IDH1 glioma immunity in mice. J Clin Invest 131(4):e139542
Billard P, Guerriau C, Carpentier C, Juillard F, Grandin N, Lomonte P, Kantapareddy P, Dufay N, POLA network et al (2021) The TeloDIAG: how telomeric parameters can help in glioma rapid diagnosis and liquid biopsy approaches. Ann Oncol 32(12):1608–1617
Van Meir EG, Hadjipanayis CG, Norden AD, Shu HK, Wen PY, Olson JJ (2010) Exciting new advances in neuro-oncology: the avenue to a cure for malignant glioma. CA Cancer J Clin 60(3):166–193
Luo J, Liu P, Lu C, Bian W, Su D, Zhu C, Xie S, Pan Y et al (2021) Stepwise crosstalk between aberrant Nf1, Tp53 and Rb signalling pathways induces gliomagenesis in zebrafish. Brain 144(2):615–635
Chen X, Ma H, Wang Z, Zhang S, Yang H, Fang Z (2017) EZH2 palmitoylation mediated by ZDHHC5 in p53-mutant glioma drives malignant development and progression. Cancer Res 77(18):4998–5010
Chen X, Hu L, Yang H, Ma H, Ye K, Zhao C, Zhao Z, Dai H et al (2019) DHHC protein family targets different subsets of glioma stem cells in specific niches. J Exp Clin Cancer Res 38(1):25
Chen X, Hao A, Li X, Ye K, Zhao C, Yang H, Ma H, Hu L et al (2020) Activation of JNK and p38 MAPK mediated by ZDHHC17 drives glioblastoma multiforme development and malignant progression. Theranostics 10(3):998–1015
Shi J, Lv S, Wu M, Wang X, Deng Y, Li Y, Li K, Zhao H et al (2020) HOTAIR-EZH2 inhibitor AC1Q3QWB upregulates CWF19L1 and enhances cell cycle inhibition of CDK4/6 inhibitor palbociclib in glioma. Clin Transl Med 10(1):182–198
Mohammad F, Weissmann S, Leblanc B, Pandey DP, Højfeldt JW, Comet I, Zheng C, Johansen JV et al (2017) EZH2 is a potential therapeutic target for H3K27M-mutant pediatric gliomas. Nat Med 23(4):483–492
Griffiths-Jones S, Grocock RJ, van Dongen S, Bateman A, Enright AJ (2006) miRBase: microRNA sequences, targets and gene nomenclature. Nucleic Acids Res 34(1):D140–D144
Uddin MS, Mamun AA, Alghamdi BS, Tewari D, Jeandet P, Sarwar MS, Ashraf GM (2020) Epigenetics of glioblastoma multiforme: from molecular mechanisms to therapeutic approaches. Semin Cancer Biol 83(1):100–120
Pottoo FH, Javed MN, Rahman JU, Abu-Izneid T, Khan FA (2021) Targeted delivery of miRNA based therapeuticals in the clinical management of Glioblastoma Multiforme. Semin Cancer Biol 69:391–398
Yu JR, LeRoy G, Bready D, Frenster JD, Saldaña-Meyer R, Jin Y, Descostes N, Stafford JM et al (2021) The H3K36me2 writer-reader dependency in H3K27M-DIPG. Sci Adv 7(29):eabg7444
Haag D, Mack N, Goncalves B, da Silva P, Statz B, Clark J, Tanabe K, Sharma T et al (2021) H3.3-K27M drives neural stem cell-specific gliomagenesis in a human iPSC-derived model. Cancer Cell 39(3):407–22 e13
Acknowledgements
We thank the members of the technical assistance team at the Institute of Health and Medical Technology, Hefei Institutes of Physical Science, Chinese Academy of Sciences.
Funding
This research was supported by the National Natural Science Foundation of China (grant numbers 82172663, 82104208, 81872066, 81773131, and 81972635), the Innovative Program of Development Foundation of Hefei Centre for Physical Science and Technology (grant number 2021HSC-CIP011).
Author information
Authors and Affiliations
Contributions
XQF, HZW, and XRC conceived and designed the experiments; MTG, XQF, WXN, HHY, and SLS performed the experiments; XQF, MTG, and XRC analyzed the data; MTG, XQF, and HHY wrote the paper. All authors read and approved the final manuscript.
Corresponding authors
Ethics declarations
Ethics Approval and Consent to Participate
The study was approved by the Institutional Review Board of the Hefei Cancer Hospital, Chinese Academy of Sciences. All animal experiments were performed in accordance with the guidelines of the Animal Use and Care Committees at the Hefei Institutes of Physical Science, Chinese Academy of Sciences.
Consent for Publication
Not applicable.
Conflict of Interest
The authors declare no competing interests.
Additional information
Publisher’s Note
Springer Nature remains neutral with regard to jurisdictional claims in published maps and institutional affiliations.
Meiting Gong and Xiaoqing Fan serve as co-first authors.
Supplementary Information
Additional file 1:
Supplementary Figure 1: Gene alterations in glioma using TCGA database. (A) OncoPrint graphs represent p53, EGFR, IDH1, RB1, TERT, and RAS alteration in 871 gliomas using TCGA database. (B) Graphs represent p53 and RAS as well as p53 and TERT co-alteration in 871 gliomas using TCGA database. Supplementary Figure 2: Western blot for p53, EGFR, and RAS in hNSCs transduced with the indicated lentiviral vectors. Supplementary Figure 3: Immunological features of RASG12V/p53-/-/TERT human neural stem cells. (A) Western blot for TNFR2 in wild-type and RASG12V/p53-/-/TERT (Conoly #1, #2 and #3) human neural stem cells (hNSCs). β-tubulin was used as the loading control. (B) Messenger RNA levels of HLA-A and HLA-DRA in wild-type and RASG12V/p53-/-/TERT (Conoly #1, #2 and #3) hNSCs were analyzed by RT-PCR. β-Actin was used as the loading control. (C) Cell viabilityof peripheral blood mononuclear cells (PBMCs) co-cultured with wild-type or RASG12V/p53-/-/TERT hNSCs was determined using the CCK-8 assay. PBMCs were isolated and divided into three groups. In the negative control group, only PBMCs were cultured alone. Wild-type or RASG12V/p53-/-/TERT hNSCs (105/mL) were co-cultured with PBMCs (103/mL). Data represent the mean ± SD of three separate experiments. (D) Expression level of MHC, HLA-ABC, and HLA-DR in wild-type or RASG12V/p53-/-/TERT hNSCs was determined by FACS. Supplementary Figure 4: The summary data for Fig. 3C. Data from three independent experiments were shown. Supplementary Figure 5: The summary data for Fig. 3F. Data from three independent experiments were shown. Supplementary Figure 6: The summary data for Fig. 4A. Data from three independent experiments were shown. Supplementary Figure 7: The summary data for Fig. 4E. Data from three independent experiments were shown. Supplementary Figure 8: Negative contribution of miR-1275 to GFAP expression by targeting the 3’ -UTR. (A) Left panel: the predicted binding site for miR-1275 in the GFAP 3'-UTR is indicated (arrow). The complementary sequence between GFAP and miR-1275 is indicated above the arrow. The nucleotide position of the targsite is indicated relative to the position of the stop codon of GFAP (the first nucleotide after the stop codon of GFAP is defined as 1). Right panel: Scheme of reporter vectors; wild-type GFAP 3'-UTR sequence (upper) and mutant GFAP 3'-UTR sequence (lower) are shown. Black triangles indicate the predicted miR-1275 binding site within the 3'-UTR of the GFAP gene. (B) Luciferase reporter assay with GFAP-3'-UTR reporter vector and miRNA fragment at hNSC. Luciferase values are relative to Renilla luciferase activity (n = 3). Error bars indicate SD. statistical analysis was performed with one-way ANOVA.
Rights and permissions
Springer Nature or its licensor (e.g. a society or other partner) holds exclusive rights to this article under a publishing agreement with the author(s) or other rightsholder(s); author self-archiving of the accepted manuscript version of this article is solely governed by the terms of such publishing agreement and applicable law.
About this article
Cite this article
Gong, M., Fan, X., Yu, H. et al. Loss of p53 Concurrent with RAS and TERT Activation Induces Glioma Formation. Mol Neurobiol 60, 3452–3463 (2023). https://doi.org/10.1007/s12035-023-03288-w
Received:
Accepted:
Published:
Issue Date:
DOI: https://doi.org/10.1007/s12035-023-03288-w