Abstract
The black timber bark beetle (Xylosandrus germanus) is a strongly invasive ambrosia beetle and an important forest pest in Slovakia. This pest is closely associated with symbiotic fungi used as its food source. We investigated the fungi associated with X. germanus adults in Slovakia. In this study, Beauveria bassiana, B. pseudobassiana, Clonostachys rosea, Fusarium oxysporum, Ophiostoma quercus, Phaeoacremonium scolyti, and Talaromyces amestolkiae were isolated and identified by morphological and molecular analyses. The fungus Ophiostoma quercus was most frequently isolated from living beetles, while the entomopathogenic Beauveria bassiana was the most commonly isolated from dead beetles. The morphological descriptions of fungi based on isolates from the surface of X. germanus adults are provided.
Similar content being viewed by others
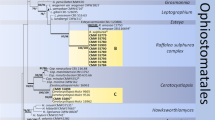
Avoid common mistakes on your manuscript.
Introduction
The black timber bark beetle Xylosandrus germanus (Blandorf 1894) (Curculionidae: Scolytinae) is native to East Asia but has spread to North America (Felt 1932; Hoffmann 1941; Schneider and Farrier 1969; Staines 1984) and Europe (Groschke 1952; Maksymov 1987; Holzschuh 1993; Frigimelica et al. 1999; Henin and Versteirt 2004; Mokrzycki et al. 2011; Galko 2013; Franjević et al. 2016; Fiala et al. 2020). Xylosandrus germanus was introduced into Europe from Japan with oak trees (Wichmann 1957). It spread at a rate of several tens of kilometres per year (Henin and Versteirt 2004). The rapid spread of X. germanus in Central Europe has been facilitated by environmental changes, particularly global warming, and an increase in the timber trade (Galko et al. 2019). The black timber bark beetle occurs in the temperate zone, in both natural and artificially planted forests of coniferous and deciduous trees, as well as in orchards of ornamental and fruit trees, vineyards, and forest nurseries (Olenici et al. 2015). It is able to withstand adverse winter conditions, and low winter temperatures do not reduce its spread in the forests of Central Europe (Dzurenko et al. 2022). Xylosandrus germanus is a polyphagous species attacking over 200 shrub and tree species (Weber and McPherson 1983). It attacks weakened or freshly dead host plants. Infested trees show symptoms of wilting, dieback, tree decline, and death (Gilrein 2011).
Xylosandrus germanus, like other ambrosia beetles, has an established symbiotic relationship with ambrosia fungi which are transmitted by females in their mycangia (Francke-Grossman 1967). Once introduced, the fungi develop in the wood as mycelia and serve as food for adults and larvae (Baker and Norris 1968). The most common ambrosia fungi associated with X. germanus are Ambrosiella hartigii L.R. Batra (Weber and McPherson 1984) and Ambrosiella grosmanniae C. Mayers, McNew & T.C. Harr. (Mayers et al. 2015). The species Ceratocystis ulmi (Buisman) C. Moreau (synonym of Ophiostoma ulmi (Buisman) Nannf.), Fusarium lateritium Nees, and F. oxysporum Schltdl. have also been reported in association with X. germanus (Norris 1979). Fusarium moniliforme J. Sheld. (syn. of F. fujikuroi Nirenberg), F. sambucinum Fuckel, and F. solani (Mart.) Sacc. were isolated from the walls of the X. germanus galleries on different tree species (Weber and McPherson 1984). An apparent symbiosis between Fusarium fungi and X. germanus causes canker on stems of Juglans nigra L. (Kessler 1974). Numerous species of fungi, namely Ambrosiella xylebori Brader ex Arx & Hennebert, Nectria haematococca Berk. & Broome (teleomorph of Fusarium solani), Alternaria alternata (Fr.) Keissl., Fusarium acuminatum Ellis & Everh., Trichoderma gamsii Samuels & Druzhin., Ophiostoma quercus (Georgev.) Nannf., Cytospora sophorae Bres., and Paraconiothyrium brasiliense Verkley, were isolated from X. germanus adults and infested wood of apple trees (Agnello et al. 2017).
In Slovakia, X. germanus was recorded for the first time in 2010 (Galko 2013). Since then, the number of individuals caught in traps has increased rapidly each year, and X. germanus has become the most abundant ambrosia beetle in the country (Galko et al. 2019). In Slovakia, attacks on recently felled trunks of oak, beech, and spruce trees by X. germanus have the greatest negative impact on timber/lumber quality. There are no published data on fungi associated with X. germanus in Slovakia. The aim of this study was to isolate and identify fungi associated with X. germanus adults on European beech, one of the most economically important trees in Slovakia.
Materials and methods
Sampling of bark beetles
In June 2018, Xylosandrus germanus adults were collected in the beech stand (Fagus sylvatica L.) of Banská Belá municipal forests (48.498288 N, 18.963989E; 494 m a.s.l.; humid continental climate) in central Slovakia. To catch X. germanus adults, two lengths of beech branch with bark measuring 20 cm in length and 10 cm in width were attached to a beetle trap (Lindgren funnel trap) baited with 96% ethanol. Longitudinal holes, approximately 8 cm in depth, were drilled into the branch sections. To make the wood samples more attractive to ambrosia beetles, 96% ethanol was poured into one of them (Galko et al. 2014). The branch sections were checked twice a week and transferred to the laboratory after they had been infested by bark beetles. Bark beetle adults collected from branch sections were separately placed in plastic tubes. They were identified to the species level according to Pfeffer (1995), and only the individuals of X. germanus were used to isolate microscopic fungi.
Fungal isolation
Live individuals of X. germanus were separately placed in Petri dishes with 3% Malt Extract Agar (MEA) medium containing streptomycin sulphate (100 mg/L), and incubated at 20 °C in the dark. The Petri dishes were checked daily, and all fungal colonies were aseptically transferred to fresh MEA plates and cultivated for 14 days. Pure isolates were transferred to Potato Dextrose Agar (PDA) plates (Merck, Darmstadt, Germany) and/or Synthetic Nutrient-poor Agar (SNA; Nirenberg 1976) to improve conidia production for morphological identification.
Dead individuals showing symptoms of infection by entomopathogenic fungi (Humber 2012) were incubated on Sabouraud Dextrose Agar (SDA) plates supplemented with fungicides (500 mg/L dodine and 250 mg/L cycloheximide) and antibiotics (50 mg/L tetracycline hydrochloride and 600 mg/L streptomycin sulphate) to obtain pure cultures. The incubation was carried out for 10–14 days at 20 °C.
Morphological observations
Microscopic characteristics of isolated fungi were analysed using a standard light microscope (Olympus BX51, Tokyo, Japan). Colony descriptions and measurements of reproductive structures were taken from fungal cultures grown at 20 °C in the dark after 14 days of incubation. The microscopic preparations were mounted in water and small hyaline spores were stained with Lactophenol Blue solution (Merck, Darmstadt). Morphological characteristics were described and measured, with 30 relevant microscopic structures measured for each. Reproductive structures were photographed using an Olympus SP350 digital camera. The fungi were identified based on their morphological and microscopic characteristics following identification manuals (Upadhyay 1981; Gerlach and Nirenberg 1982; Nelson et al. 1983; Harrington et al. 2001; Schroers 2001; Mostert et al. 2006; Rehner et al. 2011; Humber 2012; Yilmaz et al. 2014). The species identity was also confirmed through molecular analysis.
DNA extraction, PCR and sequencing
Pure isolates were used for DNA extractions. For molecular identification, fungal genomic DNA was extracted from mycelia using the E.Z.N.A. Fungal DNA Mini Kit (Omega Bio-Tek Inc., Norcross, GA, USA), following the manufacturer’s protocol. For species identification, the internal transcribed spacer (ITS) and 5.8S regions of ribosomal DNA and translation elongation factor 1-α (EF1-α) gene were amplified using primer pairs ITS1-F/ITS4 (Gardes and Bruns 1993; White et al. 1990), and EF1-728F/EF1-986R (Carbone and Kohn 1999) or EF-983F/EF-2218R (Rehner and Buckley 2005), respectively. A partial β-tubulin (BT2) gene was additionally amplified using primer pair Bt2a/Bt2b (Glass and Donaldson 1995) for a subset of isolates (excluding Beauveria). PCR conditions for the ITS and β-tubulin regions were the same as described by Pastirčáková et al. (2018). Thermal cycling parameters for EF1-α using primer pairs EF-983F/EF-2218R were according to Rehner and Buckley (2005), while for EF1-728F/EF1-986R were as follows: an initial denaturation step at 95 °C for 15 min., followed by 35 cycles of denaturing at 94 °C for 1 min., annealing at 55 °C for 30 s., elongation at 72 °C for 1 min., and a 10 min. final extension at 72 °C.
Amplification of DNA was performed using a PCR reaction mix that consisted of 2 ng/µl of template DNA, 5x HOT FIREPol Blend Master Mix (Solis BioDyne, Tartu, Estonia), forward and reverse primers (10 pmol/µl) and molecular-grade water for the 20 µl reaction. Molecular-grade water was used as negative control during the preparation of the reaction mix. Ophiostoma minus DNA (DNA extraction no. PS1, Pastirčáková et al. 2018) was used as a positive control.
The PCR products were visualized by horizontal electrophoresis on 1% (w/v) TBE agarose gels stained with Simply Safe (EURx Ltd., Gdansk, Poland) and purified using the QIAquick PCR Purification Kit (Qiagen, Hilden, Germany). The amplified products were sequenced by the Sanger sequencing method at SEQme Ltd. (Dobříš, Czech Republic). The retrieved sequences were compared using the Basic Local Alignment Search Tool (https://blast.ncbi.nlm.nih.gov/Blast.cgi). The fungal species were identified based on 99–100% sequence identity. All sequences have been deposited in the NCBI GenBank Sequence Database.
Results
Fungal isolation
The isolations from the surfaces of 60 X. germanus adults yielded a total of 15 pure fungal isolates belonging to five different fungal species. These included Clonostachys rosea (3 isolates), Fusarium oxysporum (1), Ophiostoma quercus (9), Phaeoacremonium scolyti (1), and Talaromyces amestolkiae (1).
As many as 50 beetles displaying external symptoms of infection by entomopathogenic fungi were caught in the forest and 19 in vitro cultures were isolated. The isolates were preliminarily identified as Beauveria sp. by microscopic examination. Blast-N analysis of DNA sequences of ITS and partial EF1-α regions identified two Beauveria species, B. bassiana (13 isolates) and B. pseudobassiana (6 isolates).
Morphology
The morphology of fungi isolated from X. germanus adults were examined and described as follows based on the representative isolates grown at 20 °C in the dark.
Beauveria bassiana (Bals.-Criv.) Vuill., Bull. Soc. bot. Fr. 59: 40 (1912). Figure 1.
Beauveria bassiana (isolate XG11). A colony on PDA after 14 days of incubation at 20 °C; B colony on SNA after 14 days of incubation at 20 °C; C conidiophores gregarious in dense clusters (stained with Lactophenol Blue solution); D conidiophores; E conidia. Scale bars: B = 5 mm; C = 20 μm; D, E = 10 μm
Description from culture: Colonies on PDA growing slowly, colony diam. in dark 35 mm after 14 days at 20 °C, woolly, floccose, at first white but later becoming yellowish, margin regular. Colonies on SNA growing slowly, reaching 28 mm diam. in 14 days at 20 °C, white, sparse, margin irregular. Conidiogenous cells arising either singly or more commonly in dense clusters; with subglobose to flask-shaped base, 3–6 × 2.5–4 μm, and zig-zag shaped denticulate rhachis 5–20 μm long and 1 μm wide. Conidia hyaline, aseptate, smooth-walled, globose or subglobose, 2–3 μm diam. Sexual morph not observed.
Beauveria pseudobassiana S.A. Rehner & Humber, Mycologia 103(5): 1068 (2011). Figure 2.
Beauveria pseudobassiana (isolate XG19). A colony on PDA after 14 days of incubation at 20 °C; B sparse mycelium with white conidial structures on SNA; C–D conidiophores gregarious in clusters; E conidiophores; F conidia. Scale bars: B = 200 μm; C, E, F = 10 μm; D = 20 μm. Images C–E from material stained with Lactophenol Blue solution
Description from culture: Colonies on PDA growing slowly, colony diam. in dark 35 mm after 14 days at 20 °C, woolly, floccose, at first white but later becoming creamy yellowish, margin regular. Colonies on SNA growing slowly, reaching 30 mm diam. in 14 days at 20 °C, white, sparse, margin irregular. Conidiogenous cells arising singly or in clusters; with subglobose to flask-shaped base, 3–6 × 2.5–3.5 μm, and zig-zag shaped denticulate rhachis 10–35 μm long and 1 μm wide. Conidia hyaline, aseptate, smooth-walled, subglobose or broadly ellipsoid, with slightly pointed base, 2–3.5 × 1.5–3 μm diam. Sexual morph not observed.
Clonostachys rosea (Link) Schroers, Samuels, Seifert & W. Gams, Mycologia 91(2): 369 (1999). Figure 3.
Description from culture: Colonies on PDA growing slowly, colony diam. in dark 42 mm after 14 days at 20 °C, white, felty to granulose. Primary conidiophores (Verticillium-type), smooth, hyaline, mononematous, 0–3-level verticillate, rarely with side branches, with divergent branches and phialides, 100–250 μm tall; phialides in whorls of 2–5, straight, 25–40 × 2.5–3.5 μm, each producing a small, hyaline drop of conidia. Conidia hyaline, smooth, slightly curved, 8–12 × 2.5–5.5 μm. No secondary (penicillate) conidiophores were observed. Ascomata not observed.
Fusarium oxysporum Schltdl., Fl. berol. (Berlin) 2: 139 (1824). Figure 4.
Fusarium oxysporum (isolate X4). A colony on PDA after 14 days of incubation at 20 °C; B–C conidiophores with microconidia on the surface of the growth medium; D microconidia; E sporodochia on the surface of the growth medium; F macroconidia; G–H chlamydospores formed in pairs or singly. Scale bars: B, C = 25 μm; D, G, H = 5 μm; E = 30 μm; F = 10 μm
Description from culture: Colonies on PDA growing fast, Petri dish with a diameter of 90 mm overgrown in 7 days at 20 °C. Aerial mycelium abundant, floccose, white with a purple tinge. Conidiophores 15–75 μm tall, unbranched or branched monophialides bearing microconidia; microconidia hyaline, aseptate, ellipsoidal to cylindrical, straight or curved, 6–12 × 2.5–4 μm, produced in false heads on the tips of the phialides; macroconidia slightly sickle-shaped, hyaline, thin-walled, with an attenuated apical cell and a foot-shaped basal cell, 3(–5)-septate, 25–48 × 3–5 μm, formed in sporodochia; chlamydospores terminal or intercalary in hyphae, hyaline, globose to subglobose, 5–10 μm diam., formed in pairs or singly.
Ophiostoma quercus (Georgev.) Nannf., Svenska Skogsvårdsföreningens Tidskrift 32: 408 (1934). Figure 5.
Ophiostoma quercus (isolate X8). A, B colonies on PDA after 14 days of incubation at 20 °C; C–FPesotum asexual morph; C–E Synnemata; F conidia from synnema; G–H perithecia with long necks that exude globoid masses of ascospores; I perithecium; J ostiolar hyphae at the apex of the perithecial neck; K ascospores (stained with Lactophenol Blue solution). Scale bars: C = 500 μm; D = 250 μm; E = 100 μm; F, J = 10 μm; G–I = 200 μm; K = 5 μm
Description from culture: Colonies on PDA growing slowly, reaching 45–55 mm diam. in 14 days at 20 °C, mycelium hyaline at the beginning, later becoming white to brownish grey with concentric rings of aerial mycelium. Perithecia globose, black, 120–200 μm in diam., with long necks, cylindrical, straight to flexuous, 1000–1500 μm long at maturity, with hyaline ostiolar hyphae at the apex of the perithecial neck. Asci not observed. Ascospores hyaline, aseptate, reniform, 3–4.5 × 1.5–2 μm, without mucilaginous sheath. Pesotum asexual morph: conidiophores synnematous, simple, dark brown to black becoming lighter at the apex, 500–1,200 μm long including conidiogenous apparatus. Conidia grouped in a terminal slimy droplet, aseptate, ellipsoid to ovoid, hyaline, smooth, 3–5 × 1.5–2.5 μm. Sporothrix asexual morph not observed.
Phaeoacremonium scolyti L. Mostert, Summerb. & Crous, in Mostert, Groenewald, Summerbell, Robert, Sutton, Padhye & Crous, J. Clin. Microbiol. 43(4): 1763 (2005). Figure 6.
Description from culture: Colonies on PDA growing slowly, 35 mm after 14 days at 20 °C, felty, reddish brown. Hyphae septate, verruculose, occasionally verrucose, medium brown to pale brown, 1–2 μm wide. Conidiophores mostly short and usually unbranched, subcylindrical to navicular, 0- to 3-septate, 15–38 × 1.5–2.5 μm. The apical cell of the conidiophore producing up to three phialides. Phialides terminal or lateral, cylindrical or subcylindrical, swollen or constricted at the base, tapering towards the apex, pale brown to hyaline, 12–20 × 1.5–2.5 μm. Collarettes 1.5–2 × 1–1.5 μm. Conidia hyaline, oblong-ellipsoidal or obovoid, rarely reniform or allantoid, 2.5–3.5 × 1–2 μm.
Talaromyces amestolkiae N. Yilmaz, Houbraken, Frisvad & Samson, in Yilmaz, Houbraken, Hoekstra, Frisvad, Visagie & Samson, Persoonia 29: 48 (2012). Figure 7.
Description from culture: Colonies low, plane, spreading broadly, reaching 70 mm diam. in 14 days at 20 °C on PDA. Conidiophores biverticillate symmetrical, have a greenish pigmentation; stipes smooth-walled, 90–180 × 2.5–3 μm; metulae in verticils of 3–5, measuring 10–15 × 3–4 μm; phialides acerose, 3–6 per metula, 9–12 × 2.5–3 μm; conidia aseptate, smooth, brownish green, ellipsoidal, 2–3.5 × 2.0–2.5 μm, in long chains. Ascomata not observed.
Sequence analyses
DNA was extracted from 34 fungal isolates. The primer pair ITS1-F/ITS4 produced a single amplification band from all 34 tested fungal isolates. PCR of the ITS regions ranging from 517 to 635 bp in size. Nine sequences showed 99.84 to 100% identity with Ophiostoma quercus strain N2016-1570/3/1 (MH055672). Three sequences showed 99.82% similarity to DNA sequence of Clonostachys rosea isolate FIS26 (KY378958). Three sequences had 100% similarity to DNA sequences of Fusarium oxysporum strain NL19-94008 (MZ890539), Phaeoacremonium scolyti strain STE-U 5955 (EU128034) and Talaromyces amestolkiae isolate RPeIT (KX555604).
EF1-α and β-tubulin genes confirmed the identity of Ophiostoma isolates at the species level. PCR of the EF1-α region ranging from 403 to 470 bp in size and showed 99–100% identity with DNA sequences of O. quercus strain CMW3119 (AY466696) and KFL81WbRJXM (MH283457). EF1-α sequences for four Ophiostoma isolates (X5, X6, X11, and X15) did not yield readable data. PCR of the β-tubulin region delivered products of ~ 385 bp in size and showed 99% similarity to DNA sequences of O. quercus strain KFL43716WRJSR (MH283273). β-tubulin sequence for one Ophiostoma sample (X6) did not yield readable data.
EF1-α sequences for three Clonostachys isolates showed over 98% similarity with the sequence of the type C. rosea strain CBS 710.86 (OQ944786), whereas partial BT2 sequences showed 94% (AF358161). The identification of Talaromyces isolate was supported by 100% identity with EF1-α and BT2 sequences of T. amestolkiae isolates AC5802 VI (KJ476420) and CMIAT 055 (KX827631), respectively. The EF1-α sequences for Fusarium isolate (X4) exhibited strong similarity (99.26%) with the GenBank sequence of F. oxysporum isolate CH1977 (MH828024), and the BT2 sequence for Phaeoacremonium (X12) isolate showed high similarity (99.77%) with P. scolyti strain MH950 (LN901180). BT2 sequence for Fusarium isolate and EF1-α sequence for Phaeoacremonium isolate did not yield readable data.
The identification of the entomopathogenic fungi was supported by a high degree (> 99%) of identity with the GenBank sequences (ITS and EF1-α) of the neotype Beauveria bassiana strain (ARSEF 1564) or the type strain of B. pseudobassiana (ARSEF 3405).
All sequences obtained in this study were deposited in the NCBI GenBank (Table 1).
Discussion
This study represents the first report on fungi associated with Xylosandrus germanus from Slovakia. Ophiostomatoid (Ophiostoma quercus) and non-ophiostomatoid fungi (Beauveria bassiana, B. pseudobassiana, Clonostachys rosea, Fusarium oxysporum, Phaeoacremonium scolyti, Talaromyces amestolkiae) were isolated from the X. germanus beetles.
The Beauveria genus is a group of entomopathogenic fungi that are widely distributed all over the world and have a wide range of hosts, encompassing insect orders such as Coleoptera, Diptera, Hemiptera, Hymenoptera, Lepidoptera, Orthoptera, Thysanoptera, and others. Currently, a total of 30 species of Beauveria have been recognized, with only five of them documented in Europe. These species include B. bassiana, B. brongniartii (Sacc.) Petch, B. pseudobassiana, B. caledonica Bissett & Widden, and B. varroae S.A. Rehner & Humber (Rehner et al. 2011; Khonsanit et al. 2020; Kobmoo et al. 2021; Wang et al. 2022). In this study, the presence of B. bassiana and B. pseudobassiana in X. germanus is documented for the first time. According to Wang et al. (2020), B.bassiana exhibits a wider range of hosts, whereas B. pseudobassiana primarily prevails among insects belonging to the Coleoptera and Lepidoptera orders. Furthermore, B. pseudobassiana is a species that exhibits a higher level of adaptation to forest ecosystems, as evidenced by its documented preference for forest habitats over agricultural or meadow environments (Medo et al. 2016).
The fungus Clonostachys rosea, of which three isolates were identified in this study, grows in a wide range of habitats, including soil, fungi, plant parts, nematodes, ticks, and insects (Walker and Maude 1975; Domsch et al. 1980; Mueller and Sinclair 1986; Schroers 2001; Haarith et al. 2020; Sun et al. 2020). Its ability to mycoparasitize other fungi has been used for the biological control of a wide range of plant pathogenic fungi affecting different crops and forest species (Yu and Sutton 1997; Mamarabadi et al. 2008; Rodriguez et al. 2011; Moraga-Suazo et al. 2016; Jensen et al. 2022). C. rosea interacts with fungi in bark beetle galleries, preventing colonization by blue-stain fungi, including some ophiostomatoid fungi (Seifert et al. 1988) that are vectored by bark beetles, or stimulating sexual reproduction of other mycoparasitic fungi (Kawchuk et al. 1993). This fungus also exhibits biological control of insect pests such as Callosobruchus maculatus, Oncometopia tucumana, Sonesimia grossa, Thrips tabaci, Tribolium castaneum, Trogoderma granarium, and Varroa destructor (Toledo et al. 2006; Hamiduzzaman et al. 2012; Muvea et al. 2014; Mohammed et al. 2021). C. rosea is known to be associated with hazelnuts infested by X. germanus in Turkey (Kushiyev et al. 2022) and with Ceratonia siliqua L. infested by Xylosandrus compactus in Italy (Gugliuzzo et al. 2020). C. rosea (syn. Gliocladium roseum Bainier) was also reported from bark beetles’ galleries in Poland (Bałazy 1962), Germany, Italy and Switzerland (Kirschner 2001). In Slovakia, C. rosea is known to be associated with necrosis of Pinus coulteri D.Don and P. ponderosa var. jeffreyi (Balf.) Vasey needles (Ivanová et al. 2017).
Xylosandrus ambrosia beetles are associated with Fusarium species, which can act as both nutritional symbionts and phytopathogens (Baker and Norris 1968; Kessler 1974; Contarini et al. 2020). The introduction of East Asian ambrosia beetle X. germanus into North America and Europe has been associated with host dieback and canker disease caused by Fusarium lateritium, F. oxysporum, and F. solani on Juglans nigra and Liriodendron tulipifera L. in the USA (Dochinger and Seliskar 1962; Kessler 1974; Anderson and Hoffard 1978). The fungus F. oxysporum on X. germanus has also been recorded on hazelnut in Turkey (Tuncer et al. 2018). Other Fusarium species (F. moniliforme, F. sambucinum) were isolated from X. germanus galleries in black walnut from the USA (Weber and McPherson 1984). According to Yang et al. (2008) two Fusarium species were associated with X. germanus in China and their dominance in the gallery system and in the mycangium varied with the beetle’s developmental stage. F. oxysporum associated with bark beetles on Fagus sylvatica has not yet been recorded.
The ophiostomatoid fungus Ophiostoma quercus, which was the most often isolated fungus from X. germanus beetles in this study, can be vectored by many species of bark beetles, nitidulids, weevils, and mites (Juzwik et al. 1998; Jankowiak 2012; Jankowiak and Bilański 2013; Chang et al. 2017). Nevertheless, no information has yet been published on O. quercus isolated from X. germanus. This fungus has been recorded in the wood of deciduous (Acacia, Acer, Alnus, Betula, Carpinus, Castanea, Cerasus, Eucalyptus, Fagus, Fraxinus, Nothofagus, Populus, Quercus, Salix, Tilia) and coniferous (Pinus, Pseudotsuga, Tsuga) trees (Harrington et al. 2001; Grobbelaar et al. 2009; Paciura et al. 2010; De Errasti et al. 2016; Aas et al. 2018; Jankowiak et al. 2019a, b). Harrington et al. (2001) considered the fungus to be the cause of oak decline in Europe. However, the results of inoculation studies indicate that the fungus is saprotrophic and non-pathogenic (Luque et al. 2000; Geldenhuis et al. 2004; Jankowiak 2013). So far, this fungus has been recorded in association with bark beetles on Fagus sylvatica in Austria, Belgium, France, and Poland (Carlier et al. 2006; Grobbelaar et al. 2009; Taerum et al. 2018; Jankowiak et al. 2019a, b).
Species of Phaeoacremonium are known vascular plant pathogens causing wilting and dieback of woody plants (Mostert et al. 2006). Kubátová et al. (2004) isolated Phaeoacremonium rubrigenum W. Gams, Crous & M.J. Wingf. from the galleries and larvae of a Scolytus intricatus bark beetle on Quercus robur L., as well as from adults of a Hylesinus varius (syn. Leperisinus fraxini) bark beetle on Fraxinus excelsior L. in the Czech Republic. Phaeoacremonium scolyti has been isolated from necrotic woody tissue of Prunus spp. in South Africa, from Vitis vinifera L. in France, and from the larvae of Scolytus intricatus from Czech Republic (Mostert et al. 2006; Damm et al. 2008). Our record represents the first record of P. scolyti in Slovakia. The association of X. germanus with P. scolyti is also recorded for the first time.
Talaromyces amestolkiae was also found in association with X. germanus for the first time. This is a cosmopolitan species that has been isolated from indoor house dust, sputum and lungs from cystic fibrosis patients, soil, plants, sculptures, chickenfeed, and manure (Yilmaz et al. 2016; Villanueva-Lozano et al. 2017). Strains of T. amestolkiae were also isolated from different insect hosts, Capnodis tenebrionis (Coleoptera, Buprestidae), Culex sp. (Diptera, Culicidae) and unidentified species of Pyralidae (Lepidoptera) and Pyrrhocoridae (Hemiptera) (Jaber et al. 2016). Several species of the genus Talaromyces are associated with ambrosia/bark beetles (Nicoletti and Becchimanzi 2022).
Several studies have investigated fungi associated with X. germanus in North America (Weber and McPherson 1984; Mayers et al. 2015; Agnello et al. 2017), Asia (Ito and Kajimura 2017), and Europe (Contarini et al. 2020). Ambrosiella grosmanniae and A. hartigii, known as mutualistic symbionts of X. germanus (Weber and McPherson 1984; Mayers et al. 2015), were not recorded in this study. Associations among X. germanus and many other fungi, including species of Acremonium, Alternaria, Cephalosporium, Cladosporium, Geosmithia, Graphium, and Penicillium, have also been reported (Baker and Norris 1968; Kühnholz et al. 2001; Contarini et al. 2020). Although the fungi isolated in this study were recorded for the first time in Slovakia as fungi associated with X. germanus, the mycobiota of this bark beetle in Slovakia is certainly much more diverse and a further mycological survey is needed.
Conclusions
Our research offers initial insights into the mycobiota of Xylosandrus germanus in Slovakia. Further investigation of the microscopic fungi associated with this invasive pest of European beech trees could enhance our knowledge of its effects on the health of Fagus sylvatica trees. To deepen the understanding of the complex relationship between X. germanus and its fungal symbionts further research is necessary focussing on how these interactions influence the beetle’s life cycle, reproduction, and ability to colonize new habitats. This information could be valuable for developing integrated strategies to manage X. germanus, protect beech forests, and assess associated risks.
References
Aas T, Solheim H, Jankowiak R, Bilanski P, Hausner G (2018) Four new Ophiostoma species associated with hardwood-infesting bark beetles in Norway and Poland. Fungal Biol 122:1142–1158. https://doi.org/10.1016/j.funbio.2018.08.001
Agnello AM, Breth DI, Tee EM, Cox KD, Villani SM, Ayer KM, Wallis AE, Donahue DJ, Combs DB, Davis AE, Neal JA, English-Loeb FM (2017) Xylosandrus germanus (Coleoptera: Curculionidae: Scolytinae) occurrence, fungal associations, and management trials in New York apple orchards. J Econ Entomol 110:2149–2164. https://doi.org/10.1093/jee/tox189
Anderson RL, Hoffard WH (1978) Fusarium canker ambrosia beetle complex on tulip poplar in Ohio. Plant Dis Rep 62:751
Baker JM, Norris DM (1968) A complex of fungi mutualistically involved in the nutrition of the ambrosia beetle Xyleborus ferrugineus. J Invertebr Pathol 2:246–250. https://doi.org/10.1016/0022-2011(68)90157-2
Bałazy S (1962) Obserwacje nad występowaniem niektórych grzybów owadobójczych z grupy Fungi Imperfecti na owadach leśnych. Pol Pismo Ent 3–4:149–164
Carbone I, Kohn LM (1999) A method for designing primer sets for speciation studies in filamentous ascomycetes. Mycologia 91:553–556. https://doi.org/10.1080/00275514.1999.12061051
Carlier FX, Decock C, Jacobs K, Maraite H (2006) Ophiostoma arduennense sp. nov. (Ophiostomatales, Ascomycota) from Fagus sylvatica in southern Belgium. Mycol Res 110:801–810. https://doi.org/10.1016/j.mycres.2006.03.010
Chang R, Duong TA, Taerum SJ, Wingfield MJ, Zhou X, de Beer ZW (2017) Ophiostomatoid fungi associated with conifer-infesting beetles and their phoretic mites in Yunnan,China. MycoKeys 28:19–64. https://doi.org/10.3897/mycokeys.28.21758
Contarini M, Vannini A, Giarruzzo F, Faccoli M, Morales-Rodriguez C, Rossini L, Speranza S (2020) First record of Xylosandrus germanus (Blandford) (Coleoptera: Curculionidae, Scolytinae) in the Mediterranean scrubland in Southern Italy, and its co-presence with the co-generic species X. compactus (Eichhoff) and X. crassiusculus (Motschulsky). EPPO Bull 50:311–315. https://doi.org/10.1111/epp.12660
Damm U, Mostert L, Crous PW, Fourie PH (2008) Novel Phaeoacremonium species associated with necrotic wood of Prunus trees. Persoonia 20:87–102. https://doi.org/10.3767/003158508X324227
De Errasti A, de Beer ZW, Coetzee MPA, Roux J, Rajchenberg M, Wingfield MJ (2016) Three new species of Ophiostomatales from Nothofagus in Patagonia. Mycol Prog 15:17. https://doi.org/10.1007/s11557-016-1158-z
Dochinger LS, Seliskar CE (1962) Fusarium canker found on yellow poplar. J for 60:331–333
Domsch KH, Gams W, Anderson TH (1980) Compendium of soil fungi. Academic, London
Dzurenko M, Galko J, Kulfan J, Váľka J, Holec J, Saniga M, Zúbrik M, Vakula J, Ranger CM, Skuhrovec J, Jauschová T, Zach P (2022) Can the invasive ambrosia beetle Xylosandrus germanus withstand an unusually cold winter in the West Carpathian Forest in Central Europe? Folia Oecol 49:1–8. https://doi.org/10.2478/foecol-2022-0001
Felt EP (1932) A new pest in greenhouse grown grape stems. J Econ Entomol 25:418. https://doi.org/10.1093/jee/25.2.412
Fiala T, Holuša J, Procházka J, Čížek L, Dzurenko M, Foit J, Galko J, Kašák J, Kulfan J, Lakatos F, Nakládal O, Schlaghamerský J, Svatoš M, Trombik J, Zábranský P, Zach P, Kula E (2020) Xylosandrus germanus in Central Europe: spread into and within the Czech Republic. J Appl Entomol 144:423–433. https://doi.org/10.1111/jen.12759
Francke-Grosmann H (1967) Ectosymbiosis in wood inhabiting insects. In: Henry SM (ed) Symbiosis, vol 2. Academic, New York, pp 141–205
Franjević M, Poršinsky T, Đuka A (2016) Integrated oak timber protection from ambrosia bark beetles: Economic and ecological importance in harvesting operations. Croat J Eng 37:353–364
Frigimelica G, Stergulc F, Zandigiacomo P, Faccoli M, Battisti A (1999) Xylosandrus germanus and walnut disease: an association new to Europe. In: Forster B, Knížek M, Grodzki W (eds) Methodology of forest insect and disease survey in Central Europe. Swiss Federal Institute for Forest, Snow and Landscape Research, Birmensdorf, pp 98–101
Galko J (2013) First record of the ambrosia beetle, Xylosandrus germanus (Blandford, 1984) (Coleoptera: Curculionidae, Scolytinae) in Slovakia. Lesnícky časopis– Forestry J 58:279
Galko J, Nikolov C, Kimoto T, Kunca A, Gubka A, Vakula J, Zúbrik M, Ostrihoň M (2014) Attraction of ambrosia beetles to ethanol baited traps in a Slovakian oak forest. Biologia 69:1376–1383. https://doi.org/10.2478/s11756-014-0443-z
Galko J, Dzurenko M, Ranger CM, Kulfan J, Kula E, Nikolov C, Zúbrik M, Zach P (2019) Distribution, habitat preference, and management of the invasive ambrosia beetle Xylosandrus germanus (Coleoptera: Curculionidae, Scolytinae) in European forests with an emphasis on the West Carpathians. Forests 10:10. https://doi.org/10.3390/f10010010
Gardes M, Bruns TD (1993) ITS primers with enhanced specificity for basidiomycetes– application to the identification of mycorrhizae and rusts. Mol Ecol 2:113–118. https://doi.org/10.1111/j.1365-294X.1993.tb00005.x
Geldenhuis MM, Roux J, Montenegro F, de Beer ZW, Wingfield MJ, Wingfield BD (2004) Identification and pathogenicity of Graphium and Pesotum species from machete wounds on Schizolobium parahybum in Ecuador. Fungal Divers 15:137–151
Gerlach W, Nirenberg H (1982) The genus Fusarium– a pictorial atlas. Mitt Biol Bundesanst Land- Forstwirtsch Berl-Dahl 209:1–406
Gilrein D (2011) Ambrosia beetles in the News. Branch Out 18:2
Glass NL, Donaldson GC (1995) Development of primer sets designed for use with the PCR to amplify conserved genes from filamentous ascomycetes. Appl Environ Microbiol 61:1323–1330. https://doi.org/10.1128/aem.61.4.1323-1330.1995
Grobbelaar JW, Aghayeva DN, de Beer ZW, Bloomer P, Wingfield MJ, Wingfield BD (2009) Delimitation of Ophiostoma quercus and its synonyms using multiple gene phylogenies. Mycol Prog 8:221–236. https://doi.org/10.1007/s11557-009-0594-4
Groschke F (1952) Der „Schwarze Nutzholzborkenkäfer, Xylosandrus germanus Blandf., Ein Neuer Schädling in Deutschland. J Appl Entomol 34:297–302. https://doi.org/10.1111/j.1439-0418.1953.tb00698.x
Gugliuzzo A, Criscione G, Biondi A, Aiello D, Vitale A, Polizzi G, Tropea Garzia G (2020) Seasonal changes in population structure of the ambrosia beetle Xylosandrus compactus and its associated fungi in a southern Mediterranean environment. PLoS ONE 15:e0239011. https://doi.org/10.1371/journal.pone.0239011
Haarith D, Bushley KE, Chen SY (2020) Fungal communities associated with Heterodera glycines and their potential in biological control: a current update. J Nematol 52:1–17. https://doi.org/10.21307/jofnem-2020-022
Hamiduzzaman MM, Sinia A, Guzman-Novoa E, Goodwin PH (2012) Entomopathogenic fungi as potential biocontrol agents of the ecto-parasitic mite, Varroa destructor, and their effect on the immune response of honey bees (Apis mellifera L). J Invertebr Pathol 111:237–243. https://doi.org/10.1016/j.jip.2012.09.001
Harrington TC, McNew D, Steimel J, Hofstra D, Farrell R (2001) Phylogeny and taxonomy of the Ophiostoma piceae complex and the Dutch elm disease fungi. Mycologia 93:111–136. https://doi.org/10.2307/3761610
Henin JM, Versteirt V (2004) Abundance and distribution of Xylosandrus germanus (Blandford 1894) (Coleoptera, Scolytidae) in Belgium: new observations and an attempt to outline its range. J Pest Sci 77:57–63. https://doi.org/10.1007/s10340-003-0030-5
Hoffmann CH (1941) Biological observations on Xylosandrus germanus (Bldfd). J Econ Entomol 34:38–42. https://doi.org/10.1093/jee/34.1.38
Holzschuh C (1993) Erster Nachweis Des Schwarzen Nutzholzborkenkäfers (Xylosandrus germanus) in Österreich. Forstschutz Aktuell 12–13:10
Humber RA (2012) Identification of entomopathogenic fungi. In: Lacey LA (ed) Manual of techniques in Invertebrate Pathology, 2nd edn. Academic, Amsterdam, pp 151–187
Ito M, Kajimura H (2017) Landscape-scale genetic differentiation of a mycangial fungus associated with the ambrosia beetle, Xylosandrus germanus (Blandford) (Curculionidae: Scolytinae) in Japan. Ecol Evol 7:9203–9221. https://doi.org/10.1002/ece3.3437
Ivanová H, Hamarová Ľ, Pristaš P (2017) Clonostachys rosea associated with ponderosa and Coulter pine needles in Slovakia. Biologia 72:1258–1263. https://doi.org/10.1515/biolog-2017-0145
Jaber S, Mercier A, Knio K, Brun S, Kambris Z (2016) Isolation of fungi from dead arthropods and identification of a new mosquito natural pathogen. Parasites Vectors 9:491. https://doi.org/10.1186/s13071-016-1763-3
Jankowiak R (2012) Ophiostomatoid fungi associated with Ips sexdentatus on Pinus sylvestris in Poland. Dendrobiology 68:43–54
Jankowiak R (2013) Assessing the virulence of ophiostomatoid fungi associated with the pine-infesting weevils to scots pine Pinus sylvestris L. seedlings. Acta Agrobot 66:85–94. https://doi.org/10.5586/aa.2013.026
Jankowiak R, Bilański P (2013) Diversity of ophiostomatoid fungi associated with the large pine weevil, Hylobius abietis, and infested scots pine seedlings in Poland. Ann Sci 70:391–402. https://doi.org/10.1007/s13595-013-0266-z
Jankowiak R, Bilanski P, Ostafinska A, Linnakoski R (2019a) Ophiostomatales associated with wounds on hardwood trees in Poland. Plant Pathol 68:1407–1424. https://doi.org/10.1111/ppa.13061
Jankowiak R, Strzałka B, Bilański P, Kacprzyk M, Wieczorek P, Linnakoski R (2019b) Ophiostomatoid fungi associated with hardwood-infesting bark and ambrosia beetles in Poland: taxonomic diversity and vector specificity. Fungal Ecol 39:152–167. https://doi.org/10.1016/j.funeco.2019.02.001
Jensen DF, Dubey M, Jensen B, Karlsson M (2022) Clonostachys rosea to control plant diseases. In: Köhl J, Ravensberg W (eds) Microbial bioprotectants for plant disease management. Burleigh Dodds Science Publishing, Cambridge, pp 1–43. https://doi.org/10.19103/AS.2021.0093.14
Juzwik J, Cease KR, Meyer JM (1998) Acquisition of Ophiostoma quercus and Ceratocystis fagacearum by nitidulids from O. quercus-colonized oak wilt mats. Plant Dis 82:239–243. https://doi.org/10.1094/PDIS.1998.82.2.239
Kawchuk LM, Hutchison LJ, Reid J (1993) Stimulation of growth, sporulation, and potential staining capability in Ceratocystiopsis falcata. Eur J Pathol 23:178–181. https://doi.org/10.1111/j.1439-0329.1993.tb00958.x
Kessler KJ (1974) An apparent symbiosis between Fusarium fungi and ambrosia beetles causes canker on black walnut stems. Plant Dis Rep 58:1044–1047
Khonsanit A, Luangsa-ard JJ, Thanakitpipattana D, Noisripoom W, Chaitika T, Kobmoo N (2020) Cryptic diversity of the genus Beauveria with a new species from Thailand. Mycol Prog 19:291–315. https://doi.org/10.1007/s11557-020-01557-9
Kirschner R (2001) Diversity of filamentous fungi in bark beetle galleries in central Europe. In: Misra JK, Horn BW (eds) Trichomycetes and other fungal groups. CRC, Boca Raton, pp 175–196
Kobmoo N, Arnamnart N, Pootakham W, Sonthirod C, Khonsanit A, Kuephadungphan W, Suntivich R, Mosunova OV, Giraud T, Luangsa-ard JJ (2021) The integrative taxonomy of Beauveria asiatica and B. bassiana species complexes with whole-genome sequencing, morphometric and chemical analyses. Persoonia 47:136–150. https://doi.org/10.3767/persoonia.2021.47.04
Kubátová A, Kolařík M, Pažoutová S (2004) Phaeoacremonium rubrigenum - hyphomycete associated with bark beetles found in Czechia. Folia Microbiol 49:99–104. https://doi.org/10.1007/BF02931381
Kühnholz S, Borden JH, Uzunovic A (2001) Secondary ambrosia beetles in apparently healthy trees: adaptations, potential causes and suggested research. Integr Pest Manage Rev 6:209–219. https://doi.org/10.1023/A:1025702930580
Kushiyev R, Tunçer C, Özdemir İO, Erper İ, Kalendar R, Alkan M, Özer G (2022) Molecular characterization of native entomopathogenic fungi from ambrosia beetles in hazelnut orchards of Turkey and evaluation of their in vitro efficacy. Insects 13:824. https://doi.org/10.3390/insects13090824
Luque J, Parladé J, Pera J (2000) Pathogenicity of fungi isolated from Quercus suber in Catalonia (NE Spain). Pathol 30:247–263. https://doi.org/10.1046/j.1439-0329.2000.00208.x
Maksymov JK (1987) Erstmaliger Massenbefall Des Schwarzen nutzholzborkenkäfers, Xylosandrus germanus Blandf., in Der Schweiz. Schweiz Z Forstwes 138:215–227
Mamarabadi M, Jensen B, Lübeck M (2008) Three endochitinase-encoding genes identified in the biocontrol fungus Clonostachys rosea are differentially expressed. Curr Genet 54:57. https://doi.org/10.1007/s00294-008-0199-5
Mayers CG, McNew DL, Harrington TC, Roeper RA, Fraedrich SW, Biedermann PHW, Castrillo LA, Reed SE (2015) Three genera in the Ceratocystidaceae are the respective symbionts of three independent lineages of ambrosia beetles with large, complex mycangia. Fungal Biol 119:1075–1092. https://doi.org/10.1016/j.funbio.2015.08.002
Medo J, Michalko J, Medová J, Cagáň Ľ (2016) Phylogenetic structure and habitat associations of Beauveria species isolated from soils in Slovakia. J Invertebr Pathol 140:46–50. https://doi.org/10.1016/j.jip.2016.08.009
Mohammed AA, Younus AS, Ali AN (2021) Efficacy of Clonostachys rosea, as a promising entomopathogenic fungus, against coleopteran stored product insect pests under laboratory conditions. Egypt J Biol Pest Control 31:55. https://doi.org/10.1186/s41938-021-00405-6
Mokrzycki T, Hilszczański J, Borowski J, Cieślak R, Mazur A, Miłkowski M, Szołtys H (2011) Faunistic review of Polish Platypodinae and Scolytinae (Coleoptera: Curculionidae). Pol J Entomol 80:343–364. https://doi.org/10.2478/v10200-011-0024-1
Moraga-Suazo P, Sanfuentes E, Le-Feuvre R (2016) Induced systemic resistance triggered by Clonostachys rosea against Fusarium circinatum in Pinus radiata. For Res 5:174. https://doi.org/10.4172/2168-9776.1000174
Mostert L, Groenewald JZ, Summerbell RC, Gams W, Crous PW (2006) Taxonomy and pathology of Togninia (Diaporthales) and its Phaeoacremonium anamorphs. Stud Mycol 54:1–113. https://doi.org/10.3114/sim.54.1.1
Mueller JD, Sinclair JB (1986) Occurrence and role of Gliocladium roseum in field-grown soybeans in Illinois. Trans Brit Mycol Soc 86:677–680. https://doi.org/10.1016/S0007-1536(86)80078-X
Muvea AM, Meyhöfer R, Subramanian S, Poehling HM, Ekesi S, Maniania NK (2014) Colonization of onions by endophytic fungi and their impacts on the biology of Thrips tabaci. PLoS ONE 9:e108242. https://doi.org/10.1371/journal.pone.0108242
Nelson PE, Toussoun TA, Marasas WFO (1983) Fusarium species: an Illustrated Manual for Identification. Pennsylvania State University, University Park
Nicoletti R, Becchimanzi A (2022) Talaromyces–Insect Relationships Microorganisms 10:45. https://doi.org/10.3390/microorganisms10010045
Nirenberg H (1976) Untersuchungen über die morphologische und biologische Differenzierung in Der Fusarium-Sektion Liseola. Mitt Biol Bundesanst Land Forstw 169:1–117
Norris DM (1979) The mutualistic fungi of Xyleborini beetles. In: Batra LR (ed) Insect-fungus symbiosis: nutrition, mutualism and commensalism. Allanheld, Osmun & Co., Montclair, New Jersey, pp 53–63
Olenici N, Duduman LM, Tomescu R (2015) Xylosandrus germanus (Coleoptera, Curculionidae, Scolytinae)– a potential pest of forests, orchards and vineyards in Romania. Bucov For 5:207–216
Paciura D, Zhou XD, De Beer ZW, Jacobs K, Ye H, Wingfield MJ (2010) Characterisation of synnematous bark beetle-associated fungi from China, including Graphium carbonarium sp. nov. Fungal Divers 40:75–88. https://doi.org/10.1007/s13225-009-0004-x
Pastirčáková K, Adamčíková K, Pastirčák M, Zach P, Galko J, Kováč M, Laco J (2018) Two blue-stain fungi colonizing scots pine (Pinus sylvestris) trees infested by bark beetles in Slovakia, Central Europe. Biologia 73:1053–1066. https://doi.org/10.2478/s11756-018-0114-6
Pfeffer A (1995) Zentral- Und Westpaläarktische Borken- Und Kernkäfer (Coleoptera: Scolytidae, Platypodidae). Pro Entomologia, c/o Naturhistorisches Museum, Basel
Rehner SA, Buckley EPA (2005) Beauveria phylogeny inferred from ITS and EF1-α sequences: evidence for cryptic diversification and links to Cordyceps teleomorphs. Mycologia 97:84–98. https://doi.org/10.1080/15572536.2006.11832842
Rehner SA, Minnis AM, Sung GH, Luangsa-ard JJ, Devotto L, Humber RA (2011) Phylogeny and systematics of anamorphic, entomopathogenic genus Beauveria. Mycologia 103:1055–1073. https://doi.org/10.3852/10-302
Rodriguez MA, Cabrera G, Gozzo FC, Eberlin MN, Godeas A (2011) Clonostachys rosea BAFC3874 as a Sclerotinia sclerotiorum antagonist: mechanisms involved and potential as a biocontrol agent. J Appl Microbiol 110:1177–1186. https://doi.org/10.1111/j.1365-2672.2011.04970.x
Schneider I, Farrier MH (1969) New hosts, distribution, and biological notes on an imported ambrosia beetle, Xylosandrus germanus (Coleoptera: Scolytidae). Can Entomol 101:412–415. https://doi.org/10.4039/Ent101412-4
Schroers HJ (2001) A monograph of Bionectria (Ascomycota, Hypocreales, Bionectriaceae) and its Clonostachys anamorphs. Stud Mycol 46:1–214
Seifert KA, Breuil C, Rossignol L, Best M, Saddler JN (1988) Screening for microorganisms with the potential for biological control of sapstain on unseasoned lumber. Mater Org 23:81–96
Staines CL (1984) Distribution of Xylosandrus germanus (Blandford) (Coleoptera: Scolytidae) in Maryland. Proc Entomol Soc Wash 86:702
Sun ZB, Li SD, Ren Q, Xu JL, Lu X, Sun MH (2020) Biology and applications of Clonostachys rosea. J Appl Microbiol 129:486–495. https://doi.org/10.1111/jam.14625
Taerum SJ, de Beer ZW, Marincowitz S, Jankowiak R, Wingfield MJ (2018) Ophiostoma quercus: an unusually diverse and globally widespread tree-infecting fungus. Fungal Biol 122:900–910. https://doi.org/10.1016/j.funbio.2018.05.005
Toledo AV, Virla E, Humber RA, Paradell SL, Lastra CCL (2006) First record of Clonostachys rosea (Ascomycota: Hypocreales) as an entomopathogenic fungus of Oncometopia tucumana and Sonesimia grossa (Hemiptera: Cicadellidae) in Argentina. J Invertebr Pathol 92:7–10. https://doi.org/10.1016/j.jip.2005.10.005
Tuncer C, Kushiyev R, Erper I (2018) Determination of fungal flora on Anisandrus dispar Fabricius and Xylosandrus germanus Blandford (Coleoptera: Curculionidae: Scolytinae). Acta Hortic 1226:391–398. https://doi.org/10.17660/ActaHortic.2018.1226.60
Upadhyay HP (1981) A monograph of Ceratocystis and Ceratocystiopsis. University of Georgia Press, Athens, Georgia, USA
Villanueva-Lozano H, Treviño-Rangel RJ, Renpenning-Carrasco EW, González GM (2017) Successful treatment of Talaromyces amestolkiae pulmonary infection with voriconazole in an acute lymphoblastic leukemia patient. J Infect Chemother 23:400–402. https://doi.org/10.1016/j.jiac.2016.12.017
Walker JA, Maude RB (1975) Natural occurrence and growth ofGliocladium roseum on mycelium and sclerotia of Botrytis allii. Trans Br Mycol Soc 65:335–338. https://doi.org/10.1016/S0007-1536(75)80024-6
Wang Y, Tang DX, Duan DE, Wang YB, Yu H (2020) Morphology, molecular characterization, and virulence of Beauveria pseudobassiana isolated from different hosts. J Invertebr Pathol 172:107333. https://doi.org/10.1016/j.jip.2020.107333
Wang Y, Fan Q, Wang D, Zou WQ, Tang DX, Hongthong P, Yu H (2022) Species diversity and virulence potential of the Beauveria bassiana complex and Beauveria scarabaeidicola complex. Front Microbiol 13:841604. https://doi.org/10.3389/fmicb.2022.841604
Weber BC, McPherson JE (1983) World list of host plants of Xylosandrus germanus (Blandford) (Coleoptera: Scolytidae). Coleopt Bull 37:114–134
Weber BC, McPherson JE (1984) The ambrosia fungus of Xylosandrus germanus (Coleoptera: Scolytidae). Can Entomol 116:281–283
White TJ, Bruns T, Lee S, Taylor J (1990) Amplification and direct sequencing of fungal ribosomal RNA genes for phylogenetics. In: Innis MA, Gelfand DH, Sninsky JJ, White TJ (eds) PCR protocols: a guide to methods and applications. Academic, New York, pp 315–322. https://doi.org/10.1016/B978-0-12-372180-8.50042-1
Wichmann HE (1957) Einschleppungsgeschichte und Verbreitung des Xylosandrus germanus Blandf. in Westdeutschland. J Appl Entomol 40:82–99. https://doi.org/10.1111/j.1439-0418.1957.tb00854.x
Yang QF, Ye HZ, Zhang M (2008) Composition and variety of the ambrosia fungi associated with ambrosia beetle, Xylosandrus germanus (Blandford) (Coleoptera: scolytidae). Acta Entomol Sin 51:595–600
Yilmaz N, Visagie CM, Houbraken J, Frisvad JC, Samson RA (2014) Polyphasic taxonomy of the genus Talaromyces. Stud Mycol 78:175–341. https://doi.org/10.1016/j.simyco.2014.08.001
Yilmaz N, Hagen F, Meis JF, Houbraken J, Samson RA (2016) Discovery of a sexual cycle in Talaromyces amestolkiae. Mycologia 108:70–79. https://doi.org/10.3852/15-014
Yu H, Sutton JC (1997) Morphological development and interactions of Gliocladium roseum and Botrytis cinerea in raspberry. Can J Plant Pathol 19:237–246. https://doi.org/10.1080/07060669709500518
Funding
This research was financially supported by the Scientific Grant Agency of the Ministry of Education of the Slovak Republic and of Slovak Academy of Sciences, grant number VEGA 2/0122/22, by the Slovak Research and Development Agency under the contracts No. APVV-22-0545, and by the Ministry of Agriculture and Rural Development of the Slovak Republic based on the item No. 08V0301 (Promoles).
Open access funding provided by The Ministry of Education, Science, Research and Sport of the Slovak Republic in cooperation with Centre for Scientific and Technical Information of the Slovak Republic
Author information
Authors and Affiliations
Contributions
All authors contributed to the study conception and design. Samples were collected and processed by Katarína Baková, Michal Lalík and Juraj Galko; isolation of in vitro strains and microscopic analyses were performed by Katarína Baková, Andrej Kunca, Martin Pavlík, Martin Pastirčák and Marek Barta; molecular analyses were performed by Katarína Adamčíková, Katarína Pastirčáková and Marek Barta. The first draft of the manuscript was written by Katarína Pastirčáková and Marek Barta and all authors commented on previous versions of the manuscript. All authors read and approved the final manuscript.
Corresponding author
Ethics declarations
Ethical approval
No approval of research ethics committees was required to accomplish the goals of this study.
Conflict of interest
On behalf of all authors, the corresponding author states that there is no conflict of interest.
Additional information
Publisher’s Note
Springer Nature remains neutral with regard to jurisdictional claims in published maps and institutional affiliations.
Rights and permissions
Open Access This article is licensed under a Creative Commons Attribution 4.0 International License, which permits use, sharing, adaptation, distribution and reproduction in any medium or format, as long as you give appropriate credit to the original author(s) and the source, provide a link to the Creative Commons licence, and indicate if changes were made. The images or other third party material in this article are included in the article’s Creative Commons licence, unless indicated otherwise in a credit line to the material. If material is not included in the article’s Creative Commons licence and your intended use is not permitted by statutory regulation or exceeds the permitted use, you will need to obtain permission directly from the copyright holder. To view a copy of this licence, visit http://creativecommons.org/licenses/by/4.0/.
About this article
Cite this article
Pastirčáková, K., Baková, K., Adamčíková, K. et al. Fungi associated with ambrosia beetle Xylosandrus germanus in Slovakia. Biologia (2024). https://doi.org/10.1007/s11756-024-01712-7
Received:
Accepted:
Published:
DOI: https://doi.org/10.1007/s11756-024-01712-7