Abstract
The Ti/Y modified CuO-based negative temperature coefficient (NTC) thermistors, Cu0.988-2yY0.008Ti y O (TYCO; y = 0.01, 0.015, 0.03, 0.05 and 0.07), were synthesized through a wet-chemical method followed by a traditional ceramic sintering technology. The related phase component and electrical properties were investigated. XRD results show that the TYCO ceramics have a monoclinic structure as that of CuO crystal. The TYCO ceramics can be obtained at the sintering temperature 970°C-990°C, and display the typical NTC characteristic. The NTC thermal-sensitive constants of TYCO thermistors can be adjusted from 1112 to 3700 K by changing the amount of Ti in the TYCO ceramics. The analysis of complex impedance spectra revealed that both the bulk effect and grain boundary effect contribute to the electrical behavior and the NTC effect. Both the band conduction and electron-hopping models are proposed for the conduction mechanisms in the TYCO thermistors.
Similar content being viewed by others
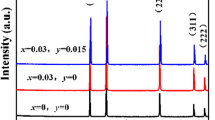
References
Feteira A. Negative temperature coefficient resistance (NTCR) ceramic thermistors: an industrial perspective. Journal of the American Ceramic Society, 2009, 92(5): 967–983
Muralidharan M N, Rohini P R, Sunny E K, et al. Effect of Cu and Fe addition on electrical properties of Ni–Mn–Co–O NTC thermistor compositions. Ceramics International, 2012, 38(8): 6481–6486
Golestani-Fard F, Azimi S, Mackenzie K J D. Oxygen evolution during the formation and sintering of nickel–manganese oxide spinels for thermistor applications. Journal of Materials Science, 1987, 22(8): 2847–2851
Feltz A, Pölzl W. Spinel forming ceramics of the system FexNiyMn3–x–yO4 for high temperature NTC thermistor applications. Journal of the European Ceramic Society, 2000, 20(14): 2353–2366
Fang D L, Chen C S, Winnubst A J A. Preparation and electrical properties of FexCu0.10Ni0.66Mn2.24–xO4 (0≤x≤0.90) NTC ceramics. Journal of Alloys and Compounds, 2008, 454(1): 286–291
Park K, Han I H. Effect of Al2O3 addition on the microstructure and electrical properties of (Mn0.37Ni0.3Co0.33–xAlx)O4 (0≤x≤0.03) NTC thermistors. Materials Science and Engineering B, 2005, 119(1): 55–60
Elilarassi R, Chandrasekaran G. Structural, optical and electron paramagnetic resonance studies on Cu-doped ZnO nanoparticles synthesized using a novel auto-combustion method. Frontiers of Materials Science, 2013, 7(2): 196–201
Macklen E D. Electrical conductivity and cation distribution in nickel manganite. Journal of Physics and Chemistry of Solids, 1986, 47(11): 1073–1079
Jung J, Töpfer J, Mürbe J, et al. Microstructure and phase development in NiMn2O4 spinel ceramics during isothermal sintering. Journal of the European Ceramic Society, 1990, 6(6): 351–359
Fau P, Bonino J P, Demai J J, et al. Thin films of nickel manganese oxide for NTC thermistor applications. Applied Surface Science, 1993, 65: 319–324
Basu A, Brinkman A W, Schmidt R. Effect of oxygen partial pressure on the NTCR characteristics of sputtered NixMn3–xO4+δ thin films. Journal of the European Ceramic Society, 2004, 24(6): 1247–1250
Xue D, Zhang H, Li Y, et al. Electrical properties of hexagonal BaTi1–xFexO3–δ (x = 0.1, 0.2, 0.3) ceramics with NTC effect. Journal of Materials Science: Materials in Electronics, 2012, 23 (7): 1306–1312
Nobre M A L, Lanfredi S. Negative temperature coefficient thermistor based on Bi3Zn2Sb3O14 ceramic: an oxide semiconductor at high temperature. Applied Physics Letters, 2003, 82(14): 2284–2286
Wang J, Zhang H, Xue D, et al. Electrical properties of hexagonal BaTi0.8Co0.2O3–δ ceramic with NTC effect. Journal of Physics D: Applied Physics, 2009, 42(23): 235103–235109
Ouyang P, Zhang H, Xue D, et al. NTC characteristic of SnSb0.05O2–BaTi0.8Fe0.2O3 composite materials. Journal of Materials Science: Materials in Electronics, 2013, 24(10): 3932–3939
Upadhyay S, Parkash O, Kumar D. Synthesis, structure and electrical behaviour of lanthanum-doped barium stannate. Journal of Physics D: Applied Physics, 2004, 37(10): 1483–1491
Zhang J, Zhang H, Yang B, et al. Temperature sensitivity of Fesubstituted SnO2-based ceramics as negative temperature coefficient thermistors. Journal of Materials Science: Materials in Electronics, 2016, 27(5): 4935–4942
Ouyang P, Zhang H, Zhang Y, et al. Zr-substituted SnO2-based NTC thermistors with wide application temperature range and high property stability. Journal of Materials Science: Materials in Electronics, 2015, 26(8): 6163–6169
Zhang Y, Wu Y, Zhang H, et al. Characterization of negative temperature coefficient of resistivity in (Sn1–xTix)0.95Sb0.05O2 (x≤0.1) ceramics. Journal of Materials Science: Materials in Electronics, 2014, 25(12): 5552–5559
Ghijsen J, Tjeng L H, van Elp J, et al. Electronic structure of Cu2O and CuO. Physical Review B: Condensed Matter and Materials Physics, 1988, 38(16): 11322–11330
Dubal D P, Gund G S, Holze R, et al. Mild chemical strategy to grow micro-roses and micro-woolen like arranged CuO nanosheets for high performance supercapacitors. Journal of Power Sources, 2013, 242: 687–698
Chen W, Zhang H, Ma Z, et al. High electrochemical performance and lithiation–delithiation phase evolution in CuO thin films for Li-ion storage. Journal of Materials Chemistry A: Materials for Energy and Sustainability, 2015, 3(27): 14202–14209
Sumikura S, Mori S, Shimizu S, et al. Photoelectrochemical characteristics of cells with dyed and undyed nanoporous p-type semiconductor CuO electrodes. Journal of Photochemistry and Photobiology A: Chemistry, 2008, 194(2–3): 143–147
Anandan S, Wen X, Yang S. Room temperature growth of CuO nanorod arrays on copper and their application as a cathode in dyesensitized solar cells. Materials Chemistry and Physics, 2005, 93 (1): 35–40
He H, Bourges P, Sidis Y, et al. Magnetic resonant mode in the single-layer high-temperature superconductor Tl2Ba2Cu6+δ. Science, 2002, 295(5557): 1045–1047
Ramirez-Ortiz J, Ogura T, Medina-Valtierra J, et al. A catalytic application of Cu2O and CuO films deposited over fiberglass. Applied Surface Science, 2001, 174(3–4): 177–184
Patil S J, Patil A V, Dighavkar C G, et al. Semiconductor metal oxide compounds based gas sensors: A literature review. Frontiers of Materials Science, 2015, 9(1): 14–37
Yang B, Zhang H, Zhang J, et al. Electrical properties and temperature sensitivity of B-substituted CuO-based ceramics for negative temperature coefficient thermistors. Journal of Materials Science: Materials in Electronics, 2015, 26(12): 10151–10158
Shannon R D. Revised effective ionic radii and systematic studies of interatomic distances in halides and chalcogenides. Acta Crystallographica Section A: Crystal Physics, 1976, 32(5): 751–767
Prasad N V, Prasad G, Bhimasankaram T, et al. Synthesis and electrical properties of SmBi5Fe2Ti3O18. Modern Physics Letters B, 1998, 12(10): 371–381
Martínez R, Kumar A, Palai R, et al. Impedance spectroscopy analysis of Ba0.7Sr03TiO3/La0.7Sr0.3MnO3 heterostructure. Journal of Physics D: Applied Physics, 2011, 44(10): 105302–105310
Azam A, Ahmed A S, Ansari M S, et al. Study of electrical properties of nickel doped SnO2 ceramic nanoparticles. Journal of Alloys and Compounds, 2010, 506(1): 237–242
Behera B, Nayak P, Choudhary R N P. Structural and impedance properties of KBa2V5O15 ceramics. Materials Research Bulletin, 2008, 43(2): 401–410
Jonscher A K. The “universal” dielectric response. Nature, 1977, 267(5613): 673–679
Author information
Authors and Affiliations
Corresponding author
Rights and permissions
About this article
Cite this article
Yang, B., Zhang, H., Guo, J. et al. Electrical properties and thermal sensitivity of Ti/Y modified CuO-based ceramic thermistors. Front. Mater. Sci. 10, 413–421 (2016). https://doi.org/10.1007/s11706-016-0355-7
Received:
Accepted:
Published:
Issue Date:
DOI: https://doi.org/10.1007/s11706-016-0355-7