Abstract
Energy harvesting (EH) from radio frequency realizes simultaneous wireless information delivery and energy transfer, which greatly reduces energy consumption in the energy-constrained Internet of things (IoT) networks. On the other hand, cognitive radio (CR) enables the unlicensed secondary user (SU) to access the spectrum resource authorized to the licensed primary user (PU) to improve spectrum efficiency. Thererfore, the integration between EH and CR can address two critical problems of energy limitation and spectrum scarcity in massive IoT wireless networks. In the state-of-art power splitting-based overlay spectrum sharing strategy, the secondary transmitter superimposes its own signals on the primary signals received and forwards the combined signals with the harvested power from the primary transmitter. However, due to low energy harvested only from one primary transmitter for the combined signal, PU system performance e.g., outage probability (OP) and system throughput, declines seriously. Moreover, a perfect direct link between the secondary transmitter and the secondary receiver must also be required in the scenario. An alternative spectrum sharing strategy based on time switching can increase harvested energy, but cooperative communication cannot be conducted at the EH period, which also reduces the overall system throughput. Based on the consideration, a Two-User Relay protocol in this paper is presented that an energy-constrained relay node, as a center hub, harvests energy from two transmitter, i.e., primary transmitter and secondary transmitter, and perform cooperative communication concurrently for PU and SU systems satisfying the PU’s performance requirements (e.g., required target rate and OP). The expressions of OP and system throughput are derived and numerical simulations are made under the different system parameters, e.g., power splitting factor, power assignment factor, transmitting signal-to-noise ratio, channel fading coefficient, and required target rate. The results prove that our proposed Two-User Relay protocol improve spectrum sharing performance. Besides, the relay node can coordinate the harvested energy distribution between PU’s signals and SU’s signals to achieve the maximum system performance. Generally, the paper provides a new relaying concept for two-user communications in the future IoT wireless networks.









Similar content being viewed by others
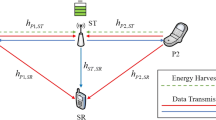
References
Gerhard, P., Hancke, B., de Carvalho, S., Gerhard, P., & Hancke, J. (2013). The role of advanced sensing in smart cities. Sensors, 13(1), 393–425.
Lee, K., Yoon, C., Jo, O., & Lee, W. (2018). Joint optimization of spectrum sensing and transmit power in energy harvesting-based cognitive radio networks. IEEE Access, 6, 30653–30662.
Chu, T. M., Chinh, H., & PhanZepernick, H. J. (2014). Hybrid interweave-underlay spectrum access for cognitive cooperative radio networks. IEEE Transactions on Communications, 62(7), 2183–2197.
Kumar, B., Sanjay Kumar, D., & Isaac, W. (2018). A survey of overlay and underlay paradigms in cognitive radio networks. International Journal of Communication Systems, 31(2), e3443.
Gurjar, D. S., Nguyen, H. H., & Tuan, H. D. (2018). Wireless information and power transfer for IoT applications in overlay cognitive radio networks. IEEE Internet of Things Journal, 6(2), 3257–3270.
Aslam, S., Ejaz, W., & Ibnkahla, M. (2018). Energy and spectral efficient cognitive radio sensor networks for internet of things. IEEE Internet of Things Journal, 5(4), 3220–3233.
Liu, L., Zhang, R., & Chua, K. C. (2013). Wireless information transfer with opportunistic energy harvesting. IEEE Transactions on Wireless Communications, 12(1), 288–300.
Xu, J., Liu, L., & Zhang, R. (2014). Multiuser MISO beamforming for simultaneous wireless information and power transfer. IEEE Transactions on Signal Processing, 62(18), 4798–4810.
Nasir, A. A., Zhou, X., Durrani, S., & Kennedy, R. A. (2013). Relaying protocols for wireless energy harvesting and information processing. IEEE Transactions on Wireless Communications, 12(7), 3622–3636.
Li, T., Fan, P., & Letaief, K. B. (2016). Outage probability of energy harvesting relay-aided cooperative networks over rayleigh fading channel. IEEE Transactions on Vehicular Technology, 65(2), 972–978.
Lee, H., Song, C., Choi, S., & Lee, I. (2017). Outage probability analysis and power splitter designs for SWIPT relaying systems with direct link. IEEE Communications Letters, 21(3), 648–651.
Men, J., Ge, J., Zhang, C., & Li, J. (2015). Joint optimal power allocation and relay selection scheme in energy harvesting asymmetric two-way relaying system. IET Communications, 9(11), 1421–1426.
Guanyao, Du., Ke, X., Zhang, Yu., & Zhengding, Q. (2014). Outage analysis and optimization for time switching-based two-way relaying with energy harvesting relay node. Ksii Transactions on Internet & Information Systems, 9(2), 1–19.
Peng, C., Li, F., & Liu, H. (2017). Optimal power splitting in two-way decode-and-forward relay networks. IEEE Communications Letters, 21(9), 2009–2012.
Yin, S., Zhang, E., Qu, Z., Yin, L., & Li, S. (2014). Optimal cooperation strategy in cognitive radio systems with energy harvesting. IEEE Transactions on Wireless Communications, 13(9), 4693–4707.
Kalamkar, S. S., & Banerjee, A. (2017). Interference-aided energy harvesting: cognitive relaying with multiple primary transceivers. IEEE Transactions on Cognitive Communications & Networking, 3(3), 313–327.
Jain, N., & Bohara, V. A. (2015). Energy harvesting and spectrum sharing protocol for wireless sensor networks. IEEE Wireless Communication Letters, 4(6), 697–700.
Wang, Z., Chen, Z., Xia, B., Luo, L., & Zhou, J. (2016). Cognitive relay networks with energy harvesting and information transfer: Design, analysis, and optimization. IEEE Transactions on Wireless Communications, 15(4), 2562–2576.
Yan, J., & Liu, Y. (2017). A dynamic SWIPT approach for cooperative cognitive radio networks. IEEE Transactions on Vehicular Technology, 66(12), 11122–11136.
Van Nguyen, B. (2018). Performance analysis of a cognitive radio network with an energy harvesting secondary transmitter under nakagami-m fading. IEEE Access, 6(2), 4135–4144.
Zhang, S., & Liew, S. C. (2009). Channel coding and decoding in a relay system operated with physical layer network coding. IEEE Journal on Selected Areas in Communications, 27(5), 788–796.
Wang, R., Tao, M., & Liu, Y. (2013). Optimal linear transceiver designs for cognitive two-way relay networks. IEEE Transactions on Signal Process, 61(4), 992–1005.
Yan, J., Zhang, C., & Gao, Z. (2014). Distributed relay selection protocols for simultaneous wireless information and power transfer. In IEEE 25th Annual International Symposium on Personal, Indoor, and Mobile Radio Communication (PIMRC), Washington, DC, (pp. 474–479).
Gradshteyn, I., & Ryzhik, I. (2007). Tables of Integrals, Series, and Products (7th ed.). Academic.
Deivanayagampillai, N. (2012). Generalized incomplete gamma function and its application. Intenational Journal of Mathematics and analysis., 4, 79–87.
Funding
This research was funded by the Science and Technology Plan of Fujian Province, China in 2020 under Grant 2020J01933, and the Faculty Research Grants of Fujian Jiangxia University, Fuzhou, China under Grant JXZ2019008.
Author information
Authors and Affiliations
Contributions
Z.L. wrote the manuscript. G.L. partially revised the paper. J.L. reviewed the paper prior to submission. Z.L. provided the concept and simulation design of the study. All authors discussed the results, analyzed the data and commented on the manuscript.
Corresponding author
Ethics declarations
Conflicts of interest
The authors declare no conflict of interest.
Additional information
Publisher's Note
Springer Nature remains neutral with regard to jurisdictional claims in published maps and institutional affiliations.
Appendix
Appendix
Here we derive the PDF of Z = Z1 + Z2.
Because Z1 and Z2 are independent, we have
If \(\phi \lambda_{1} - \lambda_{2} = 0\), we have
else
This ends the proof.□
Rights and permissions
About this article
Cite this article
Lin, Z., Li, G. & Li, J. Two-User Relay Protocol Based on Energy Harvesting and Cognitive Radio Techniques. Wireless Pers Commun 121, 3061–3083 (2021). https://doi.org/10.1007/s11277-021-08864-8
Accepted:
Published:
Issue Date:
DOI: https://doi.org/10.1007/s11277-021-08864-8