Abstract
Floods stand out as one of the most significant disasters impacting human life, causing widespread economic and social damage across the globe. Numerous research studies have concentrated on comprehending the contributing factors of flooding. Despite the prevalence of morphometry-based basin flood susceptibility analyses in existing literature, a comprehensive examination that encompasses anthropogenic features in alluvial fans is notably lacking. This study aims to evaluate the flood susceptibility of alluvial fans and their catchments in urbanised areas, integrating individual and collective basin/fan (B/F) morphometry and land use characteristics with flood inventory data. The study area selected for this investigation is the basin and fan systems situated on the northern slope of the Uludağ Massif (2543 m), the highest point in the Marmara Region, northwestern Türkiye. Twelve basin morphometric parameters were applied to 5 m resolution Digital Elevation Model data, while six fan morphometric and anthropogenic parameters were applied to alluvial fans. In addition, the results were assessed using the Normalised Morphometric Flood Index method to mitigate subjectivity in result ranking. According to the integrated basin/fan flood susceptibility results obtained through bivariate analysis, B/F6 exhibits a very high susceptibility, while B/F1, B/F3, B/F2, B/F5, B/F4, and B11/F8 demonstrate high susceptibility. B7, 8, 9, 10/F7 display a moderate potential for generating floods. These findings align significantly with historical flood events in the basin/fan area.
Similar content being viewed by others
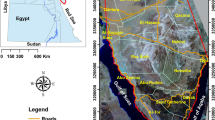
Avoid common mistakes on your manuscript.
1 Introduction
Floods are one of the most important disasters that affect human life and cause economic and social damage in almost every part of the world (CEOS 2003; Waqas et al. 2021). Floods between 2001 and 2018 led to the direct loss of 66,078 lives and indirectly impacted 1.4 billion people, resulting in an estimated economic damage of 309.4 billion US dollars (UN-Water 2020). When the population in urban areas is expected to increase from 55 to 66% in the 2050s (UN-Water 2020), there will also be an increase in the damages caused by floods in urban rivers.
Heavy rainfall is the primary factor contributing to floods, but it is essential to acknowledge that the disruption of natural processes in river basins (Liu et al. 2020), land use changes, and misapplications in channelisation (Tollan 2002; Washington 2016) can intensify the severity and scale of flooding events. In basin processes, these man-made changes and applications reduce or increase the ability to drain flows that are typically dependent on precipitation and cause flooding. In addition, linear, areal, and relief morphometric features of the basins and sub-basins also significantly impact the flood generation potential of rivers and tributaries (Ozdemir and Bird 2009). Considering their effects on flooding, quantitative morphometric analyses of drainage basins and understanding their contribution to the geomorphic processes of the basins have become important (Strahler 1952a, b, 1964; Horton 1932, 1945; Melton 1957; Shreve 1966).
Many researchers have used morphometric analyses over the past 50 years to understand basin processes and flood formation mechanisms in the basins (e.g. Patton and Baker (1976), Baumgardner (1987), Costa (1987), Gardiner (1990), Reddy et al. (2004), Ozdemir and Bird (2009), Abdel-lattif and Sherief (2012), Arnous et al.(2011), Özdemir (2011), Youssef et al. (2011), Pincot-Miller et al. (2012), Altaf et al. (2014), Ibrahim et al. (2014), Yunus et al. (2014), Farhan et al. (2015), Taha et al. (2017), Kabite et al. (2018), Alam et al. (2021)). Patton and Baker (1976) and Yunus et al. (2014) have undertaken comparative analyses of morphometric results in drainage basins of different sizes. Basin morphometric characteristics have diversified morphometric analyses in estimating flood peaks and calculating erosion levels in drainage basins in humid and semi-arid climatic regions (Baumgardner 1987; Gardiner 1990). Studies conducted in the United States have revealed differences in morphometric analyses in relatively small and large basins, particularly highlighting higher flood sensitivity in small drainage basins (Costa 1987). Reddy et al. (2004) focused on the morphometric analysis of drainage basins occurring on basaltic terrains and examined the influence of lithology on basin characteristics. Morphometric analyses were carried out on drainage networks obtained from topographic maps and DEM, and the results of different datasets were tested in different basins (Ozdemir and Bird 2009; Karabulut and Ozdemir 2019; Alam et al. 2021). Furthermore, some of these studies focus on the resolution and quality of different datasets (Abdel-lattif and Sherief 2012; Youssef et al. 2011; Ibrahim et al. 2014; Özdemir and Akbaş 2023), while others concentrate on basin potentials such as floods, erosion sensitivity, hydrological processes, and sediment transport (Pincot-Miller et al. 2012; Altaf et al. 2014; Farhan et al. 2015; Grelle et al. 2019; Ascione et al. 2022). Ozdemir and Akbas (2023) have demonstrated in their study that basin morphometry and hydraulic model results yield similar outcomes in terms of the flood-producing potential of basins.
Alluvial fans are critical geomorphological features preferred for settlement and agricultural activities and are frequently exposed to flooding within river basins (Bull 1964; Blair and McPherson 1994). Previously, studies on fans predominantly had a geomorphological content that revealed the basin-fan relationship (Bull 1964; Melton 1965; Harvey 1984; Blair and McPherson 1994; Woor et al. 2023). However, in recent years, research has shifted its focus towards floods occurring on fans and their impacts due to changes in river basin-fan systems as a result of intensive land use (Takahashi et al. 2001; Larsen et al. 2002; Santangelo et al. 2011; 2012; Santo et al. 2015; Kain et al. 2018; Mokarram et al. 2021, 2022). Since the return period of these flood events is very high (greater than 50 years), the people living in the region have not realised the actual hazard conditions, especially by local administrations (Santangelo et al. 2011). In the city of Carabellada, which was located on an alluvial fan in Venezuela, more than 19000 people lost their lives in the 1999 flood event (recurrence interval approximately 200 years), and 1.8 million tonnes of sediment covered the entire city (Larsen et al. 2002). In the Buccino region in southern Italy, a flood occurred (recurrence interval approximately 70–80 years) on the alluvial fans in front of the Vadursi and Matrura mountains, causing damage to buildings and agricultural areas, resulting in a financial loss of 2 million Euros (Santo et al. 2015). Flood and susceptibility studies have been conducted in various locations on alluvial fans in the Alps of Italy due to fan formation and their land use characteristics (Crosta and Frattini 2004; Santangelo et al. 2011, 2012; Scorpio et al. 2016; Vennari et al. 2016; Antronico et al. 2017). However, in these studies, the impact of feeder drainage basins on the fans has been only partially addressed (Santo et al. 2015). Studies investigating the flood susceptibility of alluvial fans and their basin in an integrated manner vary in the parameters and methods used for basins and fans. Karkani et al. (2021) have examined the geomorphological processes of the basins and human factors on the fans, particularly the impact of road alignments on flooding, and confirmed by the distribution of past flood events. Crosta and Frattini (2004) used 36 morphological, geological and land use parameters to demonstrate that basin morphology and land use significantly influence flood processes on the alluvial fans. The fact that the basins have primary processes, the fans have secondary processes, and human activities are concentrated on the fans reveals that flood susceptibility increases (Vincent et al. 2022). In addition, studies using hydrodynamic flood models have shown that even small geomorphologic differences on the fan lead to different flood susceptibility outcomes (Leenman et al. 2022). These studies emphasise the importance of a holistic approach to flood hazard analysis and detail the influence of geomorphological processes and human factors on flood hazard. Although the use of high-resolution data is generally recommended in recent alluvial fan studies (Scorpio et al. 2016; Karkani et al. 2021; Ghahraman and Nagy 2023), it has also been emphasised that the analysis results should be supported by an inventory of floods on the fans (Vennari et al. 2016). As mentioned in the literature, the number of studies that address both the morphological and human characteristics of basin/fan processes is still limited.
This study examines the flood susceptibility of the alluvial fans and basins underlying the city of Bursa (Türkiye), an area experiencing significant urbanisation and historical flooding, by analysing individual and integrated basin/fan morphometry and land use characteristics.
2 Study area
The study area consists of basin and fan systems on the northern slope of Uludag Massif (2543 m), the highest point of the Marmara Region in northwestern Türkiye. It is located approximately within 40° 3′- 40° 14′ north latitude, 29o1′–29o17′ east longitude and with altitudes ranging between 95 and 2543 m above sea level (Fig. 1). The basins in the study area consist of Gökdere (B1), Çukuryayla (B2), Devrengeç (B3), Akçaalan (B4), Kaplıkaya (B5), Erikli (B6), Kocabalıklı (B7), Küçükbalıklı (B8), Çardakseki (B9), Değirmenönü (B10) and Deliçay (B11) river basins from west to east. While each of these basins has individual alluvial fans, it is noteworthy that basins B7, B8, B9, and B10 collectively contribute to forming the Balıklıdere alluvial fan (F7). Therefore, a total of 8 alluvial fans and 11 river basins were considered in this study (Fig. 1).
The study area is located at the transition zone between the internally rigid Anatolian block and the north–south–extending Aegean (e.g., Şengör et al. 1981; Bozkurt 2001). The formation of the Uludağ Massif began during the Oligocene with the oblique-lateral slip of the Eskişehir Fault (Zattin et al. 2005; Uysal et al. 2006). During the Miocene, the development continued with the emergence of pull-apart basins created by the southern segment of the North Anatolian Fault (NAF) in the Marmara Region as a result of the collision between the Anatolian and African plates (e.g., Hubert-Ferrari et al. 2002; Şengör et al. 2005; Selim et al. 2013). Therefore, the geomorphology of the region is tectonically controlled by these faults, extending approximately in the E-W and NE-SW directions (Selim et al. 2013). In this respect, Uludağ Massif represents the highest relief in northwestern Türkiye. The study area exhibits a lithological diversity composed of metamorphic, volcanic, ophiolitic, plutonic, and sedimentary rocks spanning from the Precambrian to the Quaternary period. Rock types belonging to continental blocks and margin belts are tectonically related, although they present different lithology, age, and structural features (MTA 2009). Depending on the tectonic and lithological characteristics, the area consists of two main morphological units: high areas with mountains and plateaus and low areas with alluvial fans and plains (Ardel 1943; Ketin 1947). Glacial and periglacial landforms are observed at elevations above 1900 m (Zahno et al. 2010), while landforms resulting from river erosion are dominant below this elevation (Çorapçı 2022). The rivers and basins collecting their waters from the highlands deposited mixed materials such as boulders, gravel, sand, silt and clay on the plain floor and formed the alluvial fan morphology. The rest of the lowlands are plains consisting of clay, silt, sand, and gravel with increasing thickness from north to south between 80–200 m. (Tolun 1948; Sözen 1969; DSI 1973; Kutlukan 1973; Şenyuva 1991).
Climatically, the study area has Mediterranean and Humid climatic characteristics in the low and highlands, respectively (Öztürk 2010). Based on 30 year data from the meteorological stations (Fig. 1), the average annual precipitation is 674 mm in the low areas and 1453 mm in the high areas. Regarding extreme precipitation, the study area is located in the Northwest Anatolia and Central-Eastern Black Sea (NWA-CEBS) class, which occurs in the October and January months (Akbas 2023). The average temperature in the low areas is 14.5 °C, while the high area is 4.8 °C (Öztürk 2010). According to RCP 4.5 and 8.5 scenarios, an increase in precipitation is expected by 2100 in terms of the climatic characteristics of the area (Akbaş 2018). The vegetation in the area is predominantly forested at higher elevations, depending on lithology, morphology, and climatic factors, while at elevations above 1900 m, Alpine grassland vegetation prevails (Güngördü 1985).
Bursa is the fourth largest province in Türkiye, with a population of 3.214.571 according to the 2023 census (TÜİK 2023). Approximately 1,649,707 (51.59%) of this population are located on the alluvial fans where the city was settled and developed. According to the inventory data, 26 different flood events occurred in the study area between 1930 and 2023, resulting in the loss of six lives, one child and millions of Turkish Liras in economic losses (Akbaş et al. 2024). Due to flood events, channelisation and rehabilitation were carried out on the alluvial fans in most of the rivers.
3 Data and methods
In this study, 5 m resolution DEM data derived from 1:5000 scale contours using ANUDEM techniques (Hutchinson 1988), 10 m resolution ESRI land use data and high-resolution satellite images dated 2020, inventory flood data and fieldworks were used. Pre-processing analysis was applied initially to DEM data for basin and fan analysis (Bater and Coops 2009; Ozdemir and Bird 2009). This process includes fill and sink operation, flow direction and flow accumulation analysis based on the D8 method (Jenson and Domingue 1988; Martz and Jong 1988; Morris and Heerdegen 1988; Freeman 1991; Elbaşı and Özdemir 2018), river network generation based on 100 threshold value respectively (Fig. 2). For the drainage extraction, contour crenulation was applied, and a minimum accumulation threshold of 100 pixels in the head region was set by trial and error (Mark 1983; Ozdemir and Bird 2009). The alluvial fans and their feeder basins were extracted based on the apex point of each fan. After pre-processing, basin and fan analyses were conducted to determine the flood susceptibility of the study area. For each analysis, parameters commonly used in the literature have been preferred to reveal even the minimum differences in flood generation potential of each fan and its basin.
In basin analysis, linear morphometry includes bifurcation ratio, stream length ratio, and texture ratio; areal morphometry includes drainage density, elongation ratio, form factor, and stream frequency; relief morphometry includes basin relief, relief ratio, ruggedness number, time of concentration and hypsometric integral parameters (Patton and Baker 1976; Ritter et al. 2002) were utilised (Fig. 2; Table 1).
In fan analysis, the morphometric and anthropogenic characteristics of the fan were carried out in the study. While the flow process based on the Melton Ruggedness Index (MRI) (Melton 1965) and slope parameters (Santangelo et al. 2011, 2012; Santo et al. 2015; Kain et al. 2018; Mokarram et al. 2021, 2022) were used in the fan morphometric analysis, land use, bridges, river channelisation and pathway directions were used in the anthropogenic analysis of the fans (Fig. 2; Table 1).
In evaluating each parameter and the overall parameters for flood susceptibility in the basin and fan analyses, the Normalised Morphometric Flood Index (NMFI) method (Ozdemir and Akbas 2023) was used to reduce subjectivity in ranking (Eqs. 15–16).
where mi: i morphometric parameter value, mimin: the minimum value of i morphometric parameter, mimax: the maximum value of i morphometric parameter and n: number of morphometric parameters considered. The result of NMFI shows that the arithmetic means of the normalised parameters ranging from 0 to 1 were divided into four groups and classified as low (0–0.25), moderate (0.25–0.50), high (0.50–0.75) and very high (0.75–1).
There is a lack of detailed, accurate, and complete data on the location, distribution, causes, and effects of each small and big flood event that occurred in the last 100 years in Türkiye. For this reason, Akbaş et al. (2024) collected the flood inventory data of Türkiye between 1930 and 2023 by making use of the flood annals of the General Directorate of State Hydraulic Works-DSİ (1955–1997), parliamentary records of the Grand National Assembly of Türkiye (TBMM) between 1930 and 1955 when flood annals were not available, academically published papers, master and doctoral dissertations, media organisations with robust archive records such as Cumhuriyet and Milliyet newspapers, and internet news sources that started to be actively used after the 2000 year. The study area encompasses data from 26 flood events (Fig. 1), with 10 obtained from the DSİ and 16 from media outlets, offering limited information such as location (i.e. river and settlement name), total economic and social damage (i.e. the effect of people, houses, animals and vehicles), and main causative factor of the floods (i.e. heavy rainfall and snowmelt).
Finally, an integrated presentation of flood susceptibilities for the basin and fans was achieved through bivariate analysis, and these findings were subsequently compared with the existing inventory flood data.
4 Results and discussion
4.1 Flood susceptibility analysis of drainage basins
The values of variables in the selected basin morphometric parameters (Table 1) of each basin in the study area are given in Table 2 for the flood susceptibility. The following section explains the evaluation of each morphometric parameter of the drainage basins in terms of flood-generating potential based on NMFI results.
4.1.1 Linear morphometry
The morphometric parameters of bifurcation ratio (Rb), length ratio (RL), and texture ratio (T), which represent the linear characteristics of the river basins, have been examined in this section.
Rb is established according to Strahler stream index methods (Horton 1932; Strahler 1952a, b; Pike 2009). The low Rb values are often associated with high and sudden flow peaks in the river basin outlet, whereas the high Rb values are stated with more continuous and low flow characteristics (Paton and Baker 1976; Ozdemir and Akbas 2023). Rb values of the drainage basins in the study area were calculated between 2.00 and 6.75. When these findings are evaluated based on NMFI results of the basins, B2 (1.00), B6 (0.79), B1 (0.66), B3, B4, B9, B10 (0.57) and B5 (0.56) were found very high and high, and rest of the other basins were found low flood-generating potential (Fig. 3a).
RL is a calculation based on the lengths of the Strahler river orders (Horton 1945; Strahler 1964). This analysis, which indicates whether the river lengths in the given-order streams are sufficient relative to those in the upper-order streams, is vital in assessing the flood generation potential (Paton and Baker 1976). For this reason, the high RL values indicate the high flood generation potential of the basins. RL values of the basins were calculated to vary between 0.40 and 4.43. When these findings are evaluated based on NMFI results, B1 (1.00), B4 (0.78) and B8 (0.67) have been determined as very high and high, B7 (0.49), B5 (0.46), B11 (0.45), B2 (0.30), B10 (0.29) as moderate while others have been identified as low flood-generation potential (Fig. 3b).
T was developed depending on the ratio between the number of streams in the 1st Strahler order and the perimeter length of the basin (Horton 1945). This parameter expresses the ratio of the first-order streams that bring water to the main tributary within the basin. High values are associated with maximum flows, while low values indicate low flow generation potential (Horton 1932; Strahler 1952a, b; Pike 2009). T values of the study area vary between 0.42 and 5.84. When the basins are evaluated depending on NMFI results, B11 (1.00) and B1 (0.76) were obtained as very high, B5 (0.42) and B7 (0.26) basins as moderate, while the other basins as low flood generation potential (Fig. 3c).
4.1.2 Areal morphometry
In this section, the morphometric parameters of Drainage density (Dd), Stream frequency (Fs), Basin shape (Rf), and Elongation ratio (Re), which characterise the areal features of the river basins, have been analysed.
Dd is derived by dividing the total drainage length in a river basin by the basin area (Horton 1932; Strahler 1964). This metric serves as a significant criterion, elucidating the extent of fragmentation induced by rivers within drainage basins (Verstappen 1983). The drainage density is influenced by various factors, including soil and ground permeability, the degree of vegetation conditions, the distribution of relief features, and climate parameters. (Verstappen 1983; Reddy et al. 2004). Notably, river basins characterised by high drainage density are concurrently associated with high flood susceptibility (Ogden et al. 2011). Dd values of the basins were calculated to vary between 2.37 and 4.66. When these findings are evaluated based on NMFI results, B10 (1.00), B9 (0.69) and B1 (0.56) have been determined as very high and high, B3 (0.33), B5 (0.27), B8 (0.39), and B11 (0.31) as moderate while others have been identified as low flood-generation potential (Fig. 3d).
FS is determined by dividing the total number of streams within a basin by the area of the basin (Horton 1945). High Fs values indicate the existence of impermeable soils and areas marked by insufficient vegetation cover, simultaneously emphasising prominent relief features (Reddy et al. 2004). This situation ensures that the water coming with precipitation is minimally affected by losses such as infiltration, rapidly passes to surface flow and produces sudden peak flows, resulting in high flood susceptibility. Fs values of the basins were calculated to vary between 3.12 and 7.84. When these findings are evaluated based on NMFI results, B3 (1.00) has been determined as very high, B1 (0.40), B8 (0.34), and B10 (0.30) as moderate, while others have been identified as low flood-generation potential (Fig. 3e).
Rf is a parameter obtained by dividing the basin area by the square of the maximum basin length (Horton 1932). In basins with high Rf values, tributaries connect to a downstream tributary in a short time. These rapidly merging streams cause high amounts of flow. Accordingly, basins with high Rf values also have high flood susceptibility (Patton and Baker 1976). Rf values of the basins were calculated to vary between 0.14 and 0.43. When these findings are evaluated based on NMFI results, B11 (1.00) has been determined as very high, B1 (0.48), B4 (0.27), B5 (0.37) and B6 (0.31) as moderate, while others have been identified as low flood-generation potential (Fig. 3f).
Re is defined as the ratio between the diameter of a circle with the same area as the basin and the maximum length of the basin (Schumm 1956). Low Re values indicate areas with high permeability and low surface runoff, while high Re values define features with high erosive activities and extensive sediment transport, thus high flood susceptibility (Schumm 1956). The Re values of the basins were calculated to vary between 0.43 and 0.74. When these findings are evaluated based on NMFI results, B11 (1.00) and B1(0.54) have been determined as very high and high, B4 (0.33), B5 (0.43), B6 (0.37) and B9 (0.30) as moderate while others have been identified as low flood-generation potential (Fig. 3g).
4.1.3 Relief morphometry
The relief morphometric parameters such as Basin relief (Bh), Relief ratio (Rh), Ruggedness value (Rn), time of concentration (Tc) and Hypsometric integral (Hi) were analysed in this section.
Bh represents the difference between the maximum and minimum height of the basin (Schumm 1956). The high Bh value indicates that the slopes of the basin and riverbed are high in addition to the ruggedness value (Schumm 1956). Basins with high relief characteristics increase the surface flow rate and contribute positively to flood susceptibility (Özdemir 2011). Bh values of the basins were calculated to vary between 782 and 2365 m. When these findings are evaluated based on NMFI results, B11 (1.00) and B7 (0.80) have been determined as very high, B1 (0.58), B5 (0.63), B8 (0.53) high, B9(0.49), B10(0.27) as moderate while others have been identified as low flood-generation potential (Fig. 3h).
Rh is the maximum basin relief divided by the length of the basin parallel to the main river (Schumm 1956; Patton 1988). An increase in relief ratio directly leads to the steepening of slopes and increased surface runoff. A positive relationship between relief ratio and drainage density, riverbed slope and sediment has been verified (Horton 1932; Verstappen 1983; Patton 1988; Rana et al. 2016). Rh values of the basins were calculated to vary between 0.15 and 0.43. When these findings are evaluated based on NMFI results, B10 (1.00), B3 (0.99), B6 (0.97), B9 (0.81) and B2 (0.80) have been determined as very high, B4 (0.63), B8 (0.53) high while others have been identified as low flood-generation potential (Fig. 3i).
Rn is obtained by multiplying the basin relief and drainage density values (Melton 1957). It can also be considered as the degree of cleavage of the basin. As the ruggedness value of the basin increases, peak flows, erosional activities and sediment transport also increase. This situation also increases the flood susceptibility of the basins (Özdemir 2011). Rn values of the basins were calculated to vary between 1.86 and 7.26. When these findings are evaluated based on NMFI results, B11 (1.00), B9 (0.80) and B1 (0.80) have been determined as very high, B10 (0.70), B8 (0.64), B5 (0.64) and B7 (0.61) high while others have been identified as low flood-generation potential (Fig. 3j).
Tc represents the time it takes for the water to travel from the most distant point of a basin to the basin outlet (Kirpich 1940; Verstappen 1983). Conditions such as ruggedness on the topography, channel slope, channel shape, vegetation cover, and soil permeability are the main factors that change the time of concentration. There is an inverse relation between the time of concentration and flood susceptibility, especially in flash flood types (Fang 2007). Basins with low Tc values are more susceptible to flooding, while basins with high Tc values are less susceptible (Kirpich 1940; Fang 2007; Özdemir 2011). The basic principle here is that as time increases, the retention of water increases, and its effect decreases, but as time decreases, the flow accumulates rapidly and can cause sudden peaks and flooding. Tc values of the basins were calculated to vary between 4.33 (hours) and 21.39 (hours). When these findings are evaluated based on NMFI results, B2 (1.00), B3 (0.98), B6 (0.95), B4 (0.88) and B10 (0.88) have been determined as very high, B9 (0.75), B8 (0.64) and B1 (0.50) high, B5 (0.48), B7 (0.42) moderate. At the same time, B1 (0.00) have been identified as having low flood-generation potential (Fig. 3k).
Hi is one of the effective means of describing successive phases of landscape evolution, and it expresses, as a percentage, the volume of the original basin that remains (Miller et al. 1990; Ritter et al. 2002). High integral values indicate that most topography is high relative to the mean, such as smooth upland surface cut by deeply incised streams. Intermediate to low integral values are associated with more evenly dissected drainage basins. Hi and degree of dissection relationship permits it to be used as an indicator of a stage of the landscape in the Erosion Cycle (Keller and Pinter 2002). Hypsometric integral results < 0.30 indicates an old age, between 0.30–0.60 indicates a mature stage, and > 0.60 indicates a youthful stage of topography (Strahler 1952a, b). Hi values of the basins were calculated to vary between 0.48 and 0.67. Drainage basins B4, B5 and B7 are in the youth stage with values above 0.60. Drainage basins B8, B3, B11, B1, B2, B3, B6, and B9 are in the mature stage with integral values between 0.60 and 0.48. When these findings are evaluated based on NMFI results, B4 (1.00) has been determined as very high, B7 (0.72), B5 (0.68) and B8 (0.60) high, B3 (0.59), B11 (0.27) moderate while others have been identified as low flood-generation potential (Fig. 3l).
4.2 Flood susceptibility analysis of alluvial fans
4.2.1 Fan morphometry
Flow processes In order to determine the flood susceptibility of alluvial fans, it is necessary to correctly identify the river flow processes in the basin that forms the fan. River flow processes are categorised into two groups: debris flow (Df) and water flow (Wf) (Costa 1988). In alluvial fans, which are under the influence of Df, the flow is more viscous and a large amount of debris material is transported, compared Wf influenced fans (Hungr et al. 1984). Therefore, alluvial fans, especially in the Df group, have high hazard levels and flood susceptibility (Aulitzky 1980; Welsh and Davies 2011). To determine the flood susceptibility of an alluvial fan, the dominant environmental factors need to be accurately analysed. Many previous studies have compared the flood susceptibility of alluvial fan systems dominated by Wf and Df flows (De Scally et al. 2010; Santangelo et al. 2012). Based on the findings, the basin systems dominated by Df flows are smaller in size but have higher slope values. According to Melton (1965), not only the size of the incoming sediment but also the stream power and size of the drainage basins are adequate in the formation of the fan slope. He found that alluvial fans of Df character, where debris and high-viscosity flows occur, are associated with smaller-sized and high-energy basins. In this framework, he proposed a dimensionless index (Melton Index-R) which aims to classify the flow processes in alluvial fans based on basin ruggedness and size. This index has been used by many researchers in basin/fan analyses of different climate types and different sizes, especially in order to reveal the extent to which basin and fan morphometrics affect each other (Kostaschuk et al. 1986; Harvey 1988; Pasuto et al. 1992; Guzzetti et al. 1997; Melton 1957; Santangelo et al 2012). According to the Melton index results, in the case of R ≤ 0.30, the drainage basins are dominated by traditional river processes, i.e. mostly surface water flows (Wf), between 0.30 < R < 0.60, the drainage basins are dominated by surface water and intermittent debris flows (Wf and Df), and R ≥ 0.60 the drainage basins are dominated by debris flows (Df). As a result of the Melton index analysis for the study area, the R values were calculated to vary between 0.23 and 0.98. Wf flows are observed in the F1 and F8 alluvial fans, while Df and Wf flows can be mixed in the F5 alluvial fan. However, Df flows dominate in F2, F3, F4, F6 and F7 alluvial fans (Fig. 4a). When these findings are evaluated based on NMFI results, F3 (1.00), F2 (0.89), F6 (0.86) and F7 (0.76) have been determined as very high, F4 (0.62) high, while others have been identified as low flood-generation potential (Fig. 4c).
Fan slope Another morphometric factor affecting flood susceptibility in alluvial fans is the slope. Due to the variability of the slope factor, the concepts of "fan" and "cone" within the term alluvial fan have been one of the controversial issues in the scientific environment (Saito and Takashi Oguchi 2005). The main reason for this controversy over the concepts of "alluvial fan" and "alluvial cone" is that no precise accepted slope intervals have been determined (Saito and Takashi Oguchi 2005). Although fans and cones are named differently according to their slope values, they have similar processes in terms of formation mechanisms. When the studies in the literature are examined, there are different opinions about the slope values to be determined for the distinction of an alluvial fan or cone. According to Bull (1977), slope conditions below 20 degrees are sufficient for the mechanism to be an alluvial fan, whereas conditions above 20 degrees are defined as an alluvial cone. Erinç (2000) and Hoşgören (2010) propose the distinction that areas featuring slopes up to 10 degrees should be labelled as alluvial fans, whereas those with slopes ranging from 10 to 25 degrees are considered alluvial cones. However, according to Blair and Mcpherson (1994), it was emphasised that the areas with slopes between 1.5 and 25 degrees are alluvial fans, and above 25 degrees are alluvial cones. Erinç and Hoşgören's classification was preferred as the slope classification by analysing the slope values of the alluvial fan in the study area and making on-site investigations during field studies. Accordingly, F8, F1, F5 and F7 fans were classified as alluvial fans and F3, F4, F2 and F6 fans were classified as alluvial cones depending on the increasing level slope characteristics (Table 3, Fig. 4d). Alluvial cones have higher flash flood susceptibility than fans due to their slope conditions and flow processes (Santangelo et al. 2012).
4.2.2 Anthropogenic factors
In addition to morphometric analyses, anthropogenic factors are frequently used in flood susceptibility analysis of alluvial fans (Santangelo et al. 2011). In this context, anthropogenic factors such as land use, alignment of the streets on the fans, channelisation of the rivers and the presence of bridges were evaluated in terms of their effect on flooding.
Land use It is one of the most important anthropogenic factors in generating floods (Wiwandari Handayani et al. 2020; Zope et al. 2016; S. Suriya and Mudgal 2012). It has been revealed that even in rivers where the hazard is low under normal conditions, structures built by human interventions (e.g. levees, embankments, dams) increase the flood susceptibility of the area (Özdemir 2007; Sheng and Wilson 2009). Runoff and urbanisation effects are discussed in many research studies (Sheng and Wilson 2009; Fernandez and Lutz 2010; Du et al. 2012; Ozdemir and Elbasi 2015), and they concluded that the increase in urbanisation increases impervious surfaces, which in turn increases the flood generation potential (Suriya and Mudgal 2012; Zope et al. 2016; Wiwandari et al. 2020). Land use on alluvial fans was produced from satellite images in 2020, and 52 km2 (70%) of the 75 km2 alluvial fan area is heavily urbanised (Figs. 4b, 5b). However, the urbanisation rate in each alluvial fan is different. F1 and F2 have an urbanisation rate of 100%, F3 and F6 99%, F5 96%, F7 75% and F8, which has the largest area, 44% (Table 3). When these findings are evaluated according to the NMFI results, F2 (1.00), F1 (0.99), F6 (0.99), F3 (0.98), F5 (0.93), F4 (0.87) have very high, F7 (0.55) has high and F8 (0.00) has low flood generation potential (Figs. 4e, 5a).
Bridges Due to their critical role in enabling transport mobility and their vulnerable structure in the riverbed, they have been the focus of extensive research, particularly on flooding (Mitoulis et al. 2021; Loli et al. 2022). Bridges, which become a driving force in increasing the vulnerability of settlements during floods, need to be well-designed hydrologically and statically. The majority of bridges collapse due to floods, and it becomes a factor that increases the flood impact in urban areas (Setunge et al. 2014; Argyroudis and Mitoulis 2021). For instance, during the 2013 floods in the Lockyer Valley region of Australia, 43 out of 46 bridges were damaged due to the collapse of one bridge, which had a domino effect on other bridges during the floods. (Setunge et al. 2014). Therefore, the presence and number of bridges over rivers increases the impact of flooding on the fan. The number and frequency of bridges in the fans were used to determine the flood susceptibility of the fan. Considering the distance between the bridges on the fans, there are 18 bridges within 3384 m in F1, 11 at 2900 m in F5, 5 at 2235 m in F8 and 3 at 1876 m in F7 (Fig. 5b, Table 3). When these findings are evaluated according to the NMFI results, F1 (1.00), F5 (0.83) have very high, F8 (0.41) has moderate, and F7 (0.00) has low flood generation potential (Fig. 4f). There are no bridges over the F2, F3, F4 and F6 fans due to lack of natural channels. Therefore, the flood susceptibility analysis did not consider the bridge parameter for these fans.
The direction of the roads The topographic characteristics and alignment of roads directly influence the distribution of urban development and local drainage (Yin et al. 2016). In particular, roads along the slope facilitate surface runoff, while roads perpendicular to the slope increase the potential impact in case of flash flooding (Santangelo et al. 2012). In this context, the general pattern of the roads that will prevent surface flow in the fans is approximately parallel to each other in the east–west direction. Secondary roads cut these roads in the north–south direction, and since these secondary roads extend in the direction of the slope, they are interpreted as roads that facilitate the surface flow on the fans. The high number of roads perpendicular to the fan slope and the development of urbanisation on this axis will cause artificial barriers to water distribution during flooding. This results in increased susceptibility of fans to flash floods during heavy rainfall. Therefore, roads are categorised into two types regarding flood impacts: blocking and facilitating flow. The number of roads blocking the flow on alluvial fans are F5 (11), F1 (8), F7 (5), F6 (2), F2 (2), F3 (2), F4 (2), F8 (1) from high to low. When these findings were evaluated according to NMFI results, F5 (1.00) was determined as very high, F1 (0.70) as high, F7 (0.40) as medium and the others as low flood generation potential (Fig. 4g, Table 3).
River channelisation Besides urbanisation, the channelisation of natural riverbeds increases the impervious surfaces on the fans. Natural roughness decreases in the topography, going from permeable to impermeable, increasing the river's flow velocity and stream power (Helmio 2002). Unfortunately, in most of the channelisation, this process does not go beyond narrowing the cross-sections of the riverbeds and enclosing them in concrete channels. (Karakuyu 2002). Concreting accelerates the surface runoff and causes a decrease in the water infiltrated by soil and plants, disrupting the natural balance in the rainfall-runoff relationship. In the study area, the F1, F5, and F8 streams have a concrete channel throughout the fan. Natural river bed conditions are partially preserved in the streams in F7 fan (Fig. 5b). Since F2, F3, F4, and F6 fans have relatively small basins and have intensive urbanisation, a lack of natural channel formations is observed on the fan surface (Fig. 4b). This will cause surface runoff in F2, F3, F4 and F6 to flow directly over the urban settlement, leading to a similar situation in channelised rivers. When these findings are evaluated according to the NMFI results, all the fans have a very high flood generation potential, except the F7 (0.00) fan (Figs. 4h, 5c, d, Table 3).
4.3 Integrated flood susceptibility analysis (IFS)
Flood susceptibilities determined according to each parameter were analysed for basin, fan, and integrated basin/fan susceptibility using NMFI. The total flood susceptibility of the basins was analysed by evaluating 12 parameters consisting of Rb, Rl, T, Dd, Fs, Rf, Re, Bh, Rh, Rn, Tc and Hi in combination. According to results, B1 and B11 basins have high flood susceptibility with NMFI values of 0.54 and 0.51, while B2, B3, B4, B5, B6, B7, B8, B9, and B10 basins have medium flood susceptibility, respectively (0.50–0.25). No basins with low flood susceptibility were identified in the study area. The fact that high flood susceptibility values were found in basins B1 and B11, which constitute more than 60% of the study area, reveals that flood generation potential is high in most of the area.
In the alluvial fans, susceptibility parameters were determined and analysed to include anthropogenic factors and using some of the morphometric characteristics. Each parameter regarding flood susceptibility potentials in alluvial fans was evaluated using NMFI. Based on the results obtained, F6 was found to have a very high NMFI value of 0.79, F1 (0.65), F2 (0.70), F3 (0.71), F4 (0.62) and F5 (0.69) were found to have high, F7 (0.32) medium and F8 (0.23) low flood susceptibility. According to the results, flood susceptibility was higher in alluvial cones than in alluvial fans (Bull 1977). Alluvial cones also have the highest urbanisation rates among the alluvial fans. The fact that the drainage basins forming these alluvial cones are small and have moderate flood susceptibility does not mean that flooding will not occur on the alluvial cones. Indeed, the flood susceptibility of alluvial cones was found to be high and very high in the analysis results. Santangelo et al. (2011) found that during periods of extreme rainfall, very heavy and unexpected destruction on the alluvial cones in front of such small basins occurs, which supports the findings obtained. Studies on the size of alluvial fans have shown that as the fan area increases, the flood susceptibility decreases proportionally (Bull 1977; Harvey 1988). However, of the two large alluvial fans in our study area, the F8 fan supports this finding due to its partial nature, while the F1 fan has a high flood susceptibility due to the high anthropogenic impact and the consideration of these parameters in addition to morphometry. Fan F1 is highly susceptible to flooding due to its high urbanisation rate, main roads blocking the flow and river channelisation.
In order to examine the IFS of the basin and fans, normalised NMFI values were subjected to bivariate analysis in a GIS environment (Fig. 6b). The main emphasis is to evaluate and interpret the positive contribution of the variables in the basin to the flood susceptibility potential of the alluvial fan. According to the bivariate analysis of the basin/fan combination, flood susceptibility classes were found to be very high, high and medium (Fig. 6a). From the basin/fan analysis, B/F6 has a very high, B/F1, B/F3, B/F2, B/F5, B/F4 and B11/F8 have high, B7, 8, 9,10/F7 have moderate flood susceptibility (Fig. 6c). When this finding is compared with inventory flood data in the area, B/F1 has the highest number of flood events (11 events), followed by B11/F8 (8 events), B/F2 (4 events), B/F5 (2 events), and B/F4 (1 event), respectively (Akbaş et al. 2024). Depending on this comparison with the inventory data, river channelisation works on the fans, effective changes in climate and expansion area of the settlement in the last 20 years can be shown among the reasons for the decrease in flood events from the old settlements to the new ones.
5 Conclusion
The research was conducted out of 11 basins, with sizes varying from 1 to 107 km2, and 8 densely urbanised alluvial fans, ranging from 0.3 to 33.7 km2. This study also includes an integrated assessment of morphometric and anthropogenic parameters to perform flood susceptibility analyses within the context of basin and fan relationships. Basin morphometric parameters include Rb, Rl, T, Dd, Fs, Rf, Re, Bh, Rh, Rn, Tc and Hi, while fan morphometric analyses include slope and flow processes. The anthropogenic parameters used in the fans are land use, alignment of the streets on the fans, channelisation in the rivers and the presence of bridges. The findings obtained from the analyses were presented individually with basin and fan flood susceptibility values using NMFI. In addition, basin/fan flood susceptibility analyses were performed integrated using bivariate analysis and NMFI classification.
In the basin flood susceptibility results, the B1 (0.54) and B11 (0.51) basins are high, and the other basins have moderate flood generation potential. F6 fan (0.79) is very high, F3 (0.71), F2 (0.70), F5 (0.66), F1(0.65), F4 (0.62) is high, F7 (0.32) is moderate, and F8 (0.19) is low flood generating potential. In the IFS results, B/F6 has a very high, B/F1, B/F3, B/F2, B/F5, B/F4 and B11/F8 have high, B7, 8, 9, 10/F7 have moderate flood generating potential. It is evident that the analyses provide consistent results when compared with actual flood inventory data (Fig. 6a). The fact that the flood susceptibility of alluvial fans, especially small and densely urbanised, is high, and the inventory data supports these results is in line with the results of flood susceptibility of urbanised alluvial fans in the literature. The NMFI method proposed by Ozdemir and Akbas 2023 was found to be effective in the basin, alluvial fans and IFS analysis.
We believe that analyses can provide important guidance on the flood susceptibility of alluvial fans and contribute to decision-making processes used for future infrastructure development and urban planning. In addition, using flood inventory data in such studies can help formulate flood management and risk mitigation strategies more effectively, especially by providing more insights into analyses of factors such as the frequency and distribution of flood events. In future studies, diversification of the parameters in the basins and fans and especially increasing the resolution values are important issues to be investigated in terms of affecting the results of the flood susceptibility analysis.
References
Abdel-Lattif A, Sherief Y (2012) Morphometric analysis and flash floods of Wadi Sudr and Wadi Wardan, Gulf of Suez, Egypt: using digital elevation model. Arab J Geosci 2(5):181–195
Akbas A (2023) Seasonality, persistency, regionalisation, and control mechanism of extreme rainfall over complex terrain. Theoret Appl Climatol 152(3–4):981–997
Akbaş A, Görüm T, Özdemir H (2024) Türkiye’de Taşkınları Oluşturan Mekanizmaların ve Taşkın Tehlikenin Alansal ve Zamansal Dinamiklerinin Ortaya Çıkarılması. 121Y578 Nolu Tübitak Projesi
Akbaş A (2018) Marmara denizi drenaj havzasında yağış-akış ilişkileri. Doktora Tezi,İstanbul Üniversitesi Sosyal Bilimler Enstitüsü
Alam A, Ahmed B, Sammonds P (2021) Flash flood susceptibility assessment using the parameters of drainage basin morphometry in SE Bangladesh. Quatern Int 575:295–307
Altaf S, Meraj G, Romshoo SA (2014) Morphometry and land cover based multi-criteria analysis for assessing the soil erosion susceptibility of the western Himalayan watershed. Environ Monit Assess 186:8391–8412
Antronico L, Borrelli L, Coscarelli R (2017) Recent damaging events on alluvial fans along a stretch of the Tyrrhenian coast of Calabria (southern Italy). Bull Eng Geol Env 76:1399–1416
Ardel A (1943) Marmara bölgesinin güneydoğu havzalarının morfolojik karakterleri. Türk Coğrafya Dergisi 2:160–173
Argyroudis S, Mitoulis SA (2021) Vulnerability of bridges to individual and multiple hazards- floods and earthquakes. Reliab Eng Syst Saf 210:107564–107564. https://doi.org/10.1016/j.ress.2021.107564
Arnous MO, Aboulela HA, Green DR (2011) Geo-environmental hazards assessment of the northwestern Gulf of Suez, Egypt. J Coastal Conserv 15:37–50
Ascione A, Palumbo M, Lane SN, Santangelo N, Santo A, Valente E (2022) A new geomorphological method for the evaluation of debris flow magnitude: a case study from the southern Apennines (Italy) (No. ICG2022–404). Copernicus Meetings
Aulitzky H (1980) Preliminary two-fold classification of torrents. In: Proceedings International Symposium Interpraevent, Bad Ischl, 4, pp 285–309
Bater CW, Coops NC (2009) Evaluating error associated with lidar-derived DEM interpolation. Comput Geosci 35(2):289–300
Baumgardner RW (1987) Morphometric studies of subhumid and semi-arid drainage basins, Texas panhandle and Northeastern New Mexico (No. 163). Bureau of Economic Geology, University of Texas at Austin
Blair TC, McPherson JG (1994) Alluvial fans and their natural distinction from rivers based on morphology, hydraulic processes, sedimentary processes, and facies assemblages. J Sediment Res 64(3a):450–489
Bozkurt E (2001) Neotectonics of Turkey–a synthesis. Geodin Acta 14(1–3):3–30
Bull WB (1964) Geomorphology of segmented alluvial fans in western Fresno County. US Government Printing Office, California
Bull WB (1977) The alluvial-fan environment. Prog Phys Geogr 1(2):222–270
CEOS (2003) The use of earth observing satellites for hazard support: assessments and scenarios. Final Report of the CEOS Disaster Management Support Group (DMSG), November
Çorapçı F (2022) Uludağ Kütlesi Kuzeydoğusunda Şehirleşmenin Olduğu Alüvyal Fanlar Üzerinde Taşkın Duyarlılık Analizi.Yüksek lisans tezi, Bursa Uludağ Üniversitesi (Türkiye)
Costa JE (1987) Hydraulics and basin morphometry of the largest flash floods in the conterminous United States. J Hydrol 93(3–4):313–338
Costa JE (1988) Rheologic, geomorphic and sedimentologic differentiation of water floods, hyperconcentrated flows and debris flows. Flood Geomorphol 10:113–122
Crosta GB, Frattini P (2004) Controls on modern alluvial fan processes in the central Alps, northern Italy. Earth Surface Process Landforms J Br Geomorphol Res Group 29(3):267–293
De Scally FA, Owens IF, Louis J (2010) Controls on fan depositional processes in the schist ranges of the Southern Alps, New Zealand, and implications for debris-flow hazard assessment. Geomorphology 122(1–2):99–116
Devlet Su İşleri (Dsi) (1973) Bursa Ve Çayırköy Ovalarının Hidrojeolojik Etüt Raporu, Devlet Su İşleri Genel Müdürlüğü Jeoteknik Hizmetler ve Yeraltı Suları Dairesi Başkanlığı Bursa
Du J, Qian L, Rui H, Zuo T, Zheng D, Xu Y, Xu C-Y (2012) Assessing the effects of urbanisation on annual runoff and flood events using an integrated hydrological modeling system for Qinhuai River basin, China. J Hydrol 464–465:127–139. https://doi.org/10.1016/j.jhydrol.2012.06.057
Elbaşı E, Özdemir H (2018) Marmara denizi akarsu havzalarinin morfometrik analizi. J Geography-Coğrafya Dergisi 36:63–84
Erinç S, Ertek A, Güneysu C (2000) Jeomorfoloji I. Der Yayınları, İstanbul
Fang X, Thompson DB, Cleveland TG, Pradhan P (2007) Variations of time of concentration estimates using NRCS velocity method. J Irrig Drain Eng 133(4):314–322
Farhan Y, Anbar A, Enaba O, Al-Shaikh N (2015) Quantitative analysis of geomorphometric parameters of Wadi Kerak, Jordan, using remote sensing and GIS. J Water Resour Prot 7(06):456
Fernández DS, Lutz MA (2010) Urban flood hazard zoning in Tucumán Province, Argentina, using GIS and multicriteria decision analysis. Eng Geol 111(1–4):90–98
Freeman TG (1991) Calculating catchment area with divergent flow based on a regular grid. Comput Geosci 17(3):413–422
Gardiner V (1990) Drainage basin morphometry. In: Goudie AS (ed) Geomorphological techniques. Unwin Hyman, London, pp 71–81
Ghahraman K, Nagy B (2023) Flood risk on arid alluvial fans: a case study in the Joghatay Mountains, Northeast Iran. J Mt Sci 20(5):1183–1200
Grelle G, Rossi A, Revellino P, Guerriero L, Guadagno FM, Sappa G (2019) Assessment of debris-flow erosion and deposit areas by morphometric analysis and a GIS-based simplified procedure: a case study of Paupisi in the southern Apennines. Sustainability 11(8):2382
Güngördü M (1985) Güney marmara bölümü (doğu kesimi) bitki örtüsünün coğrafi şartları. Coğrafya Dergisi 1:77–94
Guzzetti F, Marchetti M, Reichenbach P (1997) Large alluvial fans in the north-central Po Plain (Northern Italy). Geomorphology 18(2):119–136
Harvey AM (1988) Controls of alluvial fan development: the alluvial fans of the Sierra de Carrascoy, Murcia, Spain. Catena Supplement 13:123–137
Harvey AM (1984) Debris flows and fluvial deposits in Spanish Quaternary alluvial fans: implications for fan morphology, pp 123–132
Helmio T (2002) Unsteady 1D flow model of compound channel with vegetated floodplains. J Hydrol 269(1–2):89–99
Horton RE (1932) Drainage basin characteristics. Am Geophys Union 13:350–361
Horton RE (1945) Erosional development of streams and their drainage basins: hydrophysical approach to quantitative morphology. Bull Geol Soc Am 56:275–370
Hoşgören MY (2010) Jeomorfoloji'nin ana çizgileri I. Çantay kitabevi, İstanbul
Hubert–Ferrari A, Armijo R, King G, Meyer B, Barka A (2002) Morphology, displacement, and slip rates along the north anatolian fault, turkey. J Geophys Res Solid Earth 107(B10):ETG-9
Hungr O, Morgan GC, Kellerhals R (1984) Quantitative analysis of debris torrent hazards for design of remedial measures. Can Geotech J 21(4):663–677
Hutchinson MF (1988) Calculation of hydrologically sound digital elevation models. In: Proceedings of the third international symposium on spatial data handling, Vol. 133, pp 117–133
Ibrahim-Bathis K, Ahmed SA (2014) Evaluation of morphometric parameters–a comparative study from Cartosat DEM, SRTM and SOI Toposheet in Karabayyanahalli sub-watershed. Karnataka Int J Res 1:679–688
Jenson SK, Domingue JO (1988) Extracting topographic structure from digital elevation data for geographic information system analysis. Photogramm Eng Remote Sens 54(11):1593–1600
Kabite G, Gessesse B (2018) Hydro-geomorphological characterisation of Dhidhessa River basin, Ethiopia. Int Soil Water Conserv Res 6(2):175–183
Kain CL, Rigby EH, Mazengarb C (2018) A combined morphometric, sedimentary, GIS and modelling analysis of flooding and debris flow hazard on a composite alluvial fan, Caveside, Tasmania. Sed Geol 364:286–301
Karabulut MS, Özdemir H (2019) Comparison of basin morphometry analyses derived from different DEMs on two drainage basins in Turkey. Environ Earth Sci 78(18):574
Karakuyu M (2002) Şehirleşmenin küresel iklim sapmaları ve taşkınlar üzerindeki etkisi. Marmara Coğrafya Dergisi 6:97–108
Karkani A, Evelpidou N, Tzouxanioti M, Petropoulos A, Santangelo N, Maroukian H, Lakidi L (2021) Flash flood susceptibility evaluation in human-affected areas using geomorphological methods—the case of 9 August 2020, Euboea Greece. A GIS-based approach. GeoHazards 2(4):366–382
Keller EA, Pinter N (2002) Active tectonics: earthquakes, uplift, and landscape. (No Title)
Ketin İ (1947) Uludağ masifinin tektoniği hakkında. Türkiye Jeoloji Bülteni 1(1):60–88
Kirpich ZP (1940) Time of concentration of small agricultural watersheds. Civ Eng 10(6):362
Kostaschuk RA, MacDonald GM, Putnam PE (1986) Depositional process and alluvial fan-drainage basin morphometric relationships near Banff, Alberta. Canada Earth Surface Process Landforms 11(5):471–484
Kutlukan E (1973) Bursa Ovası Sismik Etüdü, Mta Yayını No:5070, Ankara
Larsen MC, Wieczoreck GF, Eaton LS, Morgan BA, Torres-Sierra H (2002) Natural hazards on alluvial fans: the Venezuela debris flow and flash flood disaster, US Geological Survey No. 103–01
Leenman AS, Eaton BC, MacKenzie LG (2022) Floods on alluvial fans: implications for reworking rates, morphology and fan hazards. J Geophys Res Earth Surface. https://doi.org/10.1029/2021jf006367
Liu J, Shao W, Xiang C, Mei C, Li Z (2020) Uncertainties of urban flood modeling: Influence of parameters for different underlying surfaces. Environ Res 182:108929. https://doi.org/10.1016/j.envres.2019.108929
Loli M, Kefalas G, Dafis S, Mitoulis SA, Schmidt F (2022) Bridge-specific flood risk assessment of transport networks using GIS and remotely sensed data. Sci Total Environ 850:157976–157976. https://doi.org/10.1016/j.scitotenv.2022.157976
Mark DM (1983) Relations between field-surveyed channel networks and map-based geomorphometric measures, Inez, Kentucky. Ann Assoc Am Geogr 73(3):358–372
Martz LW, De Jong E (1988) catch: a fortran program for measuring catchment area from digital elevation models. Comput Geosci 14(5):627–640
Melton MA (1957) An analysis of the relations among elements of climate, surface properties, and geomorphology. Columbia Univ, New York
Melton MA (1965) The geomorphic and paleoclimatic significance of alluvial deposits in southern Arizona. J Geol 73(1):1–38
Miller JR, Ritter DF, Kochel RC (1990) Morphometric assessment of lithologic controls on drainage basin evolution in the Crawford Upland, south-central Indiana. Am J Sci 290(5):569–599
Mokarram M, Pourghasemi HR, Tiefenbacher JP (2021) Morphometry of AFs in upstream and downstream of floods in Gribayegan. Iran Natural Hazards 108:425–450
Mokarram M, Pourghasemi HR, Tiefenbacher JP (2022) Identification of morphometric features of alluvial fan and basins in predicting the erosion levels using ANN. Environ Earth Sci 81(3):95
Morris DG, Heerdegen RG (1988) Automatically derived catchment boundaries and channel networks and their hydrological applications. Geomorphology 1(2):131–141
Mitoulis SA, Argyroudis SA, Loli M, Imam B (2021) Restoration models for quantifying flood resilience of bridges. Eng Struct 238:112180
MTA (2009) Bursa İli Kentsel Alanların (İl-İlçe Merkezleri) Yerbilim Verileri, Mta Rapor No: 11163:52
Ogden FL, Pradhan NR, Downer CW, Zahner JA (2011) Relative importance of impervious area, drainage density, width function, and subsurface storm drainage on flood runoff from an urbanised catchment. Water Res Res. https://doi.org/10.1029/2011wr010550
Özdemir H, Akbaş A (2023) Sayısal Yükseklik Modellerindeki Mekânsal Çözünürlük Değişkenliğinin Taşkın Tehlike Analizine Etkileri. J Geogr 46:46
Ozdemir H, Akbas A (2023) Is there a consistency in basin morphometry and hydrodynamic modelling results in terms of the flood generation potential of basins? A case study from the Ulus River Basin (Türkiye). J Hydrol 625:129926
Ozdemir H, Bird D (2009) Evaluation of morphometric parameters of drainage networks derived from topographic maps and DEM in point of floods. Environ Geol 56:1405–1415
Ozdemir H, Elbaşı E (2015) Benchmarking land use change impacts on direct runoff in ungauged urban watersheds. Phys Chem Earth Parts a/b/c 79:100–107
Özdemir H (2007) Farklı Senaryolara Göre Taşkın Risk Analizi: Havran Çayı Örneği (Balıkesir), Tmmob Afet Sempozyumu ss155–165
Özdemir H (2011) Havza Morfometrisi ve Taşkınlar, Fiziki Coğrafya Araştırmaları; Sistematik ve Bölgesel (s. 457–474) Türk Coğrafya Kurumu Yayınları, İstanbul
Öztürk M (2010) Uludağ (Zirve) ve Bursa Meteoroloji İstasyonlarının Karşılaştırmalı İklimi. Türk Coğrafya Dergisi 55:13–24
Pasuto A, Marchi L, Tecca PR (1992) Tipologia del trasporto solido torrentizio su conoidi alluvionalo nell’alto bacino dell’Avisio (Dolomiti). Boll Soc Geol Ital 111(1):41–51
Patton PC (1988) Drainage basin morphometry and floods. Flood geomorphology. Wiley, New York, pp 51–64
Patton PC, Baker VR (1976) Morphometry and floods in small drainage basins subject to diverse hydrogeomorphic controls. Water Resour Res 12(5):941–952
Pike RJ, Evans IS, Hengl T (2009) Geomorphometry: a brief guide. Dev Soil Sci 33:3–30
Pincott-Miller D, McGarry D, Fairweather H, Srivastava SK (2012) Review and framework development for addressing flash flood potential using GIS assisted spatial-hydrologic modelling. In: Papers and Presentations of Queensland Surveying and Spatial Conference, Vol. 2012, pp 1–16
Rana N, Singh S, Sundriyal YP, Rawat GS, Juyal N (2016) Interpreting the geomorphometric indices for neotectonic implications: an example of Alaknanda valley, Garhwal Himalaya, India. J Earth Syst Sci 125:841–854
Reddy GPO, Maji AK, Gajbhiye KS (2004) Drainage morphometry and its influence on landform characteristics in a basaltic terrain, Central India–a remote sensing and GIS approach. Int J Appl Earth Obs Geoinf 6(1):1–16
Ritter DF, Kochel RC, Miller JR (2002) Fluvial processes. Process geomorphology, 4th edn. McGraw Hill, New York, pp 189–231
Saito K, Oguchi T (2005) Slope of alluvial fans in humid regions of Japan, Taiwan, and the Philippines. Geomorphology 70(1–2):147–162. https://doi.org/10.1016/j.geomorph.2005.04.006
Santangelo N, Santo A, Di Crescenzo G, Foscari G, Liuzza V, Sciarrotta S, Scorpio V (2011) Flood susceptibility assessment in a highly urbanised alluvial fan: the case study of Sala Consilina (southern Italy). Nat Hazard 11(10):2765–2780
Santangelo N, Daunis-i-Estadella J, Di Crescenzo G, Di Donato V, Faillace PI, Martín-Fernández JA, Scorpio V (2012) Topographic predictors of susceptibility to alluvial fan flooding, Southern Apennines. Earth Surface Processes Landforms 37(8):803–817
Santo A, Santangelo N, Di Crescenzo G, Scorpio V, De Falco M, Chirico GB (2015) Flash flood occurrence and magnitude assessment in an alluvial fan context: the October 2011 event in the Southern Apennines. Nat Hazards 78:417–442
Schumm SA (1956) Evolution of drainage systems and slopes in badlands at Perth Amboy, New Jersey. Geol Soc Am Bull 67(5):597–646
Scorpio V, Santangelo N, Santo A (2016) Multiscale map analysis in alluvial fan flood-prone areas. J Maps 12(2):382–393
Selim HH, Tüysüz O, Karakaş A, Taş KÖ (2013) Morphotectonic evidence from the southern branch of the North Anatolian Fault (NAF) and basins of the south Marmara sub-region, NW Turkey. Quatern Int 292:176–192
Şengör AC, Yilmaz Y (1981) Tethyan evolution of Turkey: a plate tectonic approach. Tectonophysics 75(3–4):181–241
Şengör AMC, Tüysüz O, Imren C, Sakınç M, Eyidoğan H, Görür N, Rangin C (2005) The north anatolian fault: a new look. Annu Rev Earth Planet Sci 33:37–112
Şenyuva TK (1991) Bursa Ovası’nın Hidrojeoloji İncelemesi, İ.Ü. Fen Bilimleri Enstitüsü, Doktora Tezi, İstanbul
Setunge S, Lokuge W, Mohseni H, Karunasena W (2014) Vulnerability of road bridge infrastructure under extreme flood events. In: AFAC & Bushfire & Natural Hazards CRC Conference 2014. University of Southern Queensland
Sheng J, Wilson J (2009) Watershed urbanisation and changing flood behavior across the Los Angeles metropolitan region. Nat Hazards 48(1):41–57. https://doi.org/10.1007/s11069-008-9241-7
Shreve RL (1966) Statistical law of stream numbers. J Geol 74(1):17–37
Sözen M (1969) Bursa Ve Çayırköy Ovaları Jeofizik Rezistivite Etüd Raporu,Dsi Jeoteknik Hizmetleri Ve Yeraltısuları Dairesi, Rapor No:0351/1a-B, Ankara
Strahler AN (1952a) Hypsometric (area-altitude) analysis of erosional topography. Geol Soc Am Bull 63(11):1117–1142
Strahler AN (1952b) Dynamic basis of geomorphology. Geol Soc Am Bull 63(9):923–938
Strahler AN (1964) Quantitative Geomorphology of Drainage Basins and Channel Networks. In: Chow VT (Ed.), Handbook of Applied Hydrology, New York, pp 440–474
Suriya S, Mudgal BV (2012) Impact of urbanisation on flooding: the thirusoolam sub watershed – a case study. J Hydrol 412–413:210–219. https://doi.org/10.1016/j.jhydrol.2011.05.008
Taha MM, Elbarbary SM, Naguib DM, El-Shamy IZ (2017) Flash flood hazard zonation based on basin morphometry using remote sensing and GIS techniques: a case study of Wadi Qena basin, Eastern Desert. Egypt Remote Sensing Appl Soc Environ 8:157–167
Takahashi T, Nakagawa H, Satofuka Y, Kawaike K (2001) Flood and sediment disasters triggered by 1999 rainfall in Venezuela: a river restoration plan for an alluvial fan. J Nat Dis Sci 23(2):65–82
Tollan A (2002) Land-use change and floods: what do we need most, research, or management? Water Sci Technol 45(8):183–190. https://doi.org/10.2166/wst.2002.0176
Tolun N (1948) Bursa Ovası’nın Hidrolojisi Hakkında Not, Mta Rapor No: 1799, Ankara
TÜİK (2023) Population census of 2023. https://www.tuik.gov.tr/ (Access date: 03.05.2024)
Uysal IT, Mutlu H, Altunel E, Karabacak V, Golding SD (2006) Clay mineralogical and isotopic (K–Ar, δ18O, δD) constraints on the evolution of the North Anatolian Fault Zone. Turkey Earth Planetary Sci Lett 243(1–2):181–194
Vennari C, Parise M, Santangelo N, Santo A (2016) A database on flash flood events in Campania, southern Italy, with an evaluation of their spatial and temporal distribution. Nat Hazard 16(12):2485–2500
Verstappen HT (1983) Applied geomorphology: geomorphological surveys for environmental development. (No Title)
Vincent LT, Eaton BC, Leenman AS, Jakob M (2022) Secondary geomorphic processes and their influence on alluvial fan morphology, channel behavior and flood hazards. J Geophys Res Earth Surface. https://doi.org/10.1029/2021jf006371
Waqas H, Lu L, Tariq A, Li Q, Baqa MF, Xing J, Sajjad A (2021) Flash flood susceptibility assessment and zonation using an integrating analytic hierarchy process and frequency ratio model for the Chitral District, Khyber Pakhtunkhwa. Pakistan Water 13(12):1650
Washington U (2016) Effects of Urban Development on Floods. Usgs.gov. https://pubs.usgs.gov/fs/fs07603/.Accessed 26 June 2023
Water UN (2020) Water and climate change. The United Nations World Water Development Report; UNESCO: Paris, France. Accessed 12 September 2023
Welsh A, Davies T (2011) Identification of alluvial fans susceptible to debris-flow hazards. Landslides 8(2):183–194
Wiwandari Handayani, Uchendu Eugene Chigbu, Iwan Rudiarto, & Intan Mutiara Putri (2020) Urbanization and increasing flood risk in the northern coast of central java—Indonesia: an assessment towards better land use policy and flood management. Land 9(10):343–343. https://doi.org/10.3390/land9100343
Woor S, Thomas DS, Parton A, Leenman A (2023) Morphology and controls of the mountain front fan systems of the Hajar Mountains, south-east Arabia. Earth-Sci Rev. https://doi.org/10.1016/j.earscirev.2023.104316
Yin J, Yu D, Yin Z, Liu M, He Q (2016) Evaluating the impact and risk of pluvial flash flood on intra-urban road network: a case study in the city center of Shanghai, China. J Hydrol 537:138–145. https://doi.org/10.1016/j.jhydrol.2016.03.037
Youssef AM, Pradhan B, Hassan AM (2011) Flash flood risk estimation along the St. Katherine Road, southern Sinai, Egypt using GIS based morphometry and satellite imagery. Environ Earth Sci 62(3):611–623
Yunus AP, Oguchi T, Hayakawa YS (2014) Morphometric analysis of drainage basins in the Western Arabian Peninsula using multivariate statistics. Int J Geosci. https://doi.org/10.4236/ijg.2014.55049
Zahno C, Akçar N, Yavuz V, Kubik PW, Schlüchter C (2010) Chronology of late pleistocene glacier variations at the uludağ mountain, nw turkey. Quat Sci Rev 29(9–10):1173–1187
Zattin M, Okay AI, Cavazza W (2005) Fission-track evidence for late Oligocene and mid-Miocene activity along the North Anatolian Fault in south-western Thrace. Terra Nova 17(2):95–101
Zope PE, Eldho TI, Jothiprakash V (2016) Impacts of land use–land cover change and urbanisation on flooding: a case study of Oshiwara River Basin in Mumbai, India. CATENA 145:142–154. https://doi.org/10.1016/j.catena.2016.06.009
Funding
Open access funding provided by the Scientific and Technological Research Council of Türkiye (TÜBİTAK). The authors declare that no funds, grants, or other support were received during the preparation of this manuscript.
Author information
Authors and Affiliations
Contributions
All authors contributed to the study conception and design. Material preparation, data collection and analysis were performed by Furkan Corapci. The first draft of the manuscript was written by Furkan Corapci and Hasan Ozdemir commented on previous versions of the manuscript. All authors read and approved the final manuscript.
Corresponding author
Ethics declarations
Conflict of interests
The authors have no relevant financial or non-financial interests to disclose.
Additional information
Publisher's Note
Springer Nature remains neutral with regard to jurisdictional claims in published maps and institutional affiliations.
Rights and permissions
Open Access This article is licensed under a Creative Commons Attribution 4.0 International License, which permits use, sharing, adaptation, distribution and reproduction in any medium or format, as long as you give appropriate credit to the original author(s) and the source, provide a link to the Creative Commons licence, and indicate if changes were made. The images or other third party material in this article are included in the article's Creative Commons licence, unless indicated otherwise in a credit line to the material. If material is not included in the article's Creative Commons licence and your intended use is not permitted by statutory regulation or exceeds the permitted use, you will need to obtain permission directly from the copyright holder. To view a copy of this licence, visit http://creativecommons.org/licenses/by/4.0/.
About this article
Cite this article
Corapci, F., Ozdemir, H. A new approach to flood susceptibility analysis of urbanised alluvial fans: the case of Bursa City (Türkiye). Nat Hazards (2024). https://doi.org/10.1007/s11069-024-06723-w
Received:
Accepted:
Published:
DOI: https://doi.org/10.1007/s11069-024-06723-w