Abstract
This study aims to determine whether the application of cattle manure and associated bioturbation by dung beetles and other invertebrates can increase oak seedling establishment in the high biodiversity Mediterranean silvopastoral systems known as “dehesas”, which are comprised of scattered oaks and a grassland layer and are used for livestock rearing. A two-year study was conducted in a representative dehesa in southern Spain. Firstly, the effect of cattle dung pads on acorn consumption by livestock and wild predators was monitored, along with dung beetle abundance and plot bioturbation. Secondly, the indirect effects of bioturbation on acorn burial, acorn predation by rodents and oak seedling establishment were assessed under the condition of livestock exclusion. The results consistently demonstrate a high positive indirect effect of the presence of cattle manure on the persistence of acorns, as well as on seedling establishment, as a result of reduced predation by rodents and improved microhabitat conditions for the acorns and seedlings. These processes were mediated by bioturbation. Tunneler dung beetles, which move a great amount of soil material, were dominant in our records, which suggest their importance for passive acorn burial, without disregarding the influence of other edaphic invertebrates present. We conclude that the presence of cattle manure significantly improves oak seedling establishment in Mediterranean dehesas through the passive burial of acorns by bioturbation, which acts to protect them from predation by rodents, desiccation and other damage. Passive acorn burial by dung beetles and other invertebrates thus emerges as an important ecosystem service that has been little-studied to date. Appropriate rotational management of livestock could therefore contribute to addressing the bottleneck of oak regeneration in Mediterranean dehesas.
Similar content being viewed by others
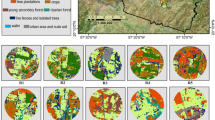
Avoid common mistakes on your manuscript.
Background
Dung beetles (coprophilous species of the Scarabaeoidea superfamily (Martín-Piera and López-Colón 2000)) usually provide many ecological functions and ecosystem services such as improvement of soil nutrient cycling, aeration and water infiltration, among others (Miranda 2006; Nichols et al. 2008; Aarons et al. 2009; Manning et al. 2016). Another important function of dung beetles is their role as secondary seed dispersers by promoting vertical and horizontal displacement of small seeds contained in herbivore dung, a process known as diplochory (Vander Wall and Longland 2004; Santos-Heredia et al. 2010). This secondary seed dispersal has been described as essential for plant recruitment because it diminishes seed predation and pathogen infection risk and provides suitable physical conditions for seed germination (Vander Wall and Longland 2004, but see D´hondt et al. 2008). Seed characteristics, particularly size, are also important for seed deposition patterns in the secondary dispersal process (Andresen and Levey 2004; Braga et al. 2017; Griffiths et al. 2016). Moreover, the effect of dung beetles on the movement of seeds embedded in dung and on soil properties is generally accepted to depend upon their own reproductive and feeding behaviors, which are commonly used to establish different functional groups (Carpaneto et al. 2007; Halffter and Matthews 1966, but see de Castro-Arrazola et al. 2020). Dung beetles are thus classified into three groups according to their main nesting strategy: paracoprids (tunnelers), which dig vertical chambers to bury brood balls; telecoprids (rollers), which transport balls horizontally before burying them; and endocoprids (dwellers), which brood their young inside the dung mass (Nichols et al. 2008; Halffter and Edmonds 1982). However, tunneler and roller dung beetles also produce a high level of soil bioturbation through the digging process, with upward displacement of soil particles to the soil surface. The consequent accumulation of excavated soil may passively bury the non-endozoochorous seeds that drop and which would otherwise remain on the soil surface exposed to high desiccation and predation risk. This process is likely to be particularly important for the survival of large-sized seeds that do not tolerate being chewed by herbivores. To our knowledge, however, this passive seed burial process has received little attention.
The Mediterranean area of the western and southwestern Iberian Peninsula hosts a silvopastoral ecosystem known as a “dehesa” in Spain. Dehesas are created by thinning of the Mediterranean forests through clearing and browsing, and are used for extensive livestock rearing. They cover 3.5–4 million ha (Olea et al. 2005), thus constituting the largest agroforestry ecosystem in Europe (Eichhorn et al. 2010). Dehesas are composed of scattered oaks in a grassland matrix in which shrubs are generally absent or very scarce. The major tree species are holm oak (Quercus ilex L. subsp. ballota) and cork oak (Quercus suber L.) in monospecific or mixed combinations, but other oak species can also occur under more humid conditions, e.g. the pyrenean oak (Q. pyrenaica Willd.) in central Spain (Costa et al. 2006 and 2017). The grassland component is comprised of Mediterranean annuals. In addition to their productive value, dehesas host an important biodiversity. They are protected under the EU Habitat Directive and are recognized as a Biosphere Reserve by UNESCO.
One major threat to the long-term persistence of these silvopastoral ecosystems is their poor oak regeneration (Pulido et al. 2013). This, in combination with a high local incidence of root rot producing exotic pathogens such as Phytophthora cinnamomi (Duque-Lazo et al. 2018), could compromise their long-term persistence. With regard to the low levels of oak regeneration, livestock overstocking and non-rotational management are usually recognized as important bottlenecks due to acorn overconsumption and seedling trampling (Gómez et al. 2003; Faust et al. 2011; Leiva and Vera 2015). Prevention of shrub encroachment by the livestock also helps to maintain an open structure, which implies a lack of suitable sites for the natural dispersal of acorns (Pulido and Díaz 2005; Leiva and Vera 2015). Mice are also frequent and they are major predators of wild acorns in the dehesas (Pulido and Díaz 2005; Leiva and Vera 2015; Leiva and Diaz-Maqueda 2016) and other predators, including birds (Columba palumbus, Cyanopica cyanus, Grus grus, Pica pica), wild ungulates (Cervus elaphus, Sus scrofa), or mid-sized mammals (Meles meles, Oryctolagus cuniculus) can also occur. Some of these acorn predators may also act as dispersers, with differing relative importance of the predator vs. disperser roles. Mice predominantly act as acorn predators (Gómez et al. 2019), although they may also act as dispersers since they can cache the acorns when they find suitable shelter conditions (Gómez et al. 2008). However, the foraging decisions of the mice are largely dictated by the low shrub cover in the dehesas, which generally increases acorn consumption relative to caching (Morán-López et al. 2015). Thus, any spontaneous biological process that can protect acorns from predation, provide suitable sites in which acorns can avoid desiccation and promote ontogenetic development can contribute to oak seedling establishment and thus the persistence of the dehesas.
Many processes related to dehesa functioning and the strengths and weakness of its conservation have been investigated to date. Livestock overgrazing and its derived detrimental effects on biodiversity and soil degradation have often been evaluated (FAO 2020; Leiva and Vera 2015); however, the ecological role of livestock in the dehesa through their interactions with other wild components of these ecosystems is not fully understood, despite the importance of livestock to their economic and social viability. This study focuses on the potential indirect effects of livestock on oak seedling establishment. We hypothesize that cattle feces may have a positive effect on oak seedling establishment through passive acorn burial mediated by dung beetles, which could provide safe sites for both acorns and seedlings. These processes are studied in a Mediterranean dehesa in southern Spain. The specific aims of this study are: (I) to determine whether acorns that fall on cattle dung pads more successfully escape predation by regular consumers of acorns in the dehesa compared to those that fall directly on the ground; and (II) to assess whether dung beetle-mediated bioturbation brings about passive acorn burial and whether such burial improves escape of rodent predation and subsequent oak seedling establishment in the two major oak species present in the dehesas: Q. suber and Q. ilex subsp. ballota.
Materials and methods
Site description
The study was conducted in a representative Mediterranean dehesa stand, located close to the Atlantic coastline in the Doñana Natural Park of southwestern Spain (37º 14′ 33′′ N; 6º 19′ 37′′ W). The topography of the study site is flat and mean elevation is 39 m a.s.l. The climate is Mediterranean, with wet, mild winters and long, dry summers. Mean annual temperature is 17 ºC and mean annual precipitation is 590 mm, although rainfall events are very irregular (García-Barrón et al. 2011). The soils are sandy, derived from quarzitic sands originating from marine regression. The vegetation is a savannah-like formation composed of scattered cork oaks (Quercus suber L.) and holm-oaks (Q. ilex L. subsp. ballota (Desf.) Samp) present at a mean density of 30 trees ha−1. The latter species is mainly found where fine soil elements are more abundant than in the surrounding area (clay + silt is 4 to 14% higher in the holm oak location, see details in Ibañez et al. 2021). The understory comprises Mediterranean annual grasslands in which the herbaceous plant species germinate in autumn and complete their reproductive cycle and die in spring. Bromus hordeaceus L., Chamaemelum mixtum L., Erodium moschatum L., and Ornithopus sativus Brot. are among the most representative of these species. The stand is grazed by free-range cattle (autochthonous Retinta breed, 110 individuals grazing on 350 ha), which tend to concentrate and forage beneath the oak canopies during the acorn dropping season (mid-autumn to end-winter period, Leiva and Diaz-Maqueda 2016), especially at the beginning of this period, when the acorns constitute the best available resource in the field due to poor pasture development at this time. In addition to the livestock, mice (wood mouse Apodemus sylvaticus and the Algerian mouse Mus spretus) are major wild predators of the acorns. These rodents can consume > 90% of the experimental acorns when livestock is excluded. Other, less abundant, wild acorn predators present in the area are the rabbits (Oryctolagus cuniculus) and badgers (Meles meles) (Leiva and Vera 2015). Avian predators or predator-dispersers of acorns, such as cranes (Grus grus), pigeons (Columba palambus), jays (Garrulus glandarius) or magpies (Pica pica), have never been recorded in the area.
Experimental design
This study was conducted over two years. Firstly (year 2017–2018), a field trial was developed in order to assess whether the presence of dung pads has any effect on acorn consumption by livestock and wild predators. The presence of dung beetles in the stand and plot bioturbation were also monitored. Secondly (year 2018–2019), a field trial was conducted under livestock exclusion in order to assess the potential indirect effect of bioturbation on acorn persistence and oak seedling establishment. A schematic summary of the different trials is presented in Table 1.
Effect of cattle dung pads on acorn persistence under livestock presence. Bioturbation and dung beetle assemblage assessment. (First year trial)
In a 10 ha meadow, we selected and marked 16 fresh cattle dung pads of diameter ca. 30 cm (hereafter, dung pad plots). Eight of these dung pad plots were each beneath the canopy of a Q. suber tree and the other eight were each beneath the canopy of a Q. ilex subsp. ballota tree. The same number of plots, identical in size but free of cattle dung pads (hereafter, ground plots), were similarly established beneath the canopies of Q. suber and Q. ilex subsp. ballota trees. The trial thus included four treatments, each with eight replicates. Minimum distance between plots was 50 m. Eight fresh Q. suber or Q. ilex subsp. ballota acorns (depending on the treatment) were marked with a white spot for identification and placed on the soil surface in each plot in order to mimic naturally fallen acorns. The experimental acorns were regularly monitored every three days for two weeks and weekly during the next fortnight (one month in total), recording the number of persistent undamaged acorns (i.e., acorns that had not been gnawed or partially destroyed). The acorns that were not immediately visible, due to potential burial, were carefully detected by inserting a metal rod into the soil at regular intervals in the plots. This trial was carried out from November 16th to December 14th in the case of Q. ilex subsp. ballota, and from December 14th to January 12th in the case of Q. suber, following the species-specific differences in peak acorn-dropping (Gómez-Casero et al. 2007).
Plot bioturbation was assessed concurrently with the acorn persistence monitoring. For this procedure, a metal grid (49 cells, mesh size 5 × 5 cm) on a wooden frame was temporarily placed in each plot, fixing the corners in place with tent pegs. The state of the soil surface in each cell was recorded, distinguishing between disturbed (those that presented soil mounds, holes or any other disturbance) and intact (those that were covered by dung or presented undisturbed soil) cells. Sampling was repeated every three days for two weeks.
Dung beetles were also sampled at the same time as the bioturbation assessment. For this, a pitfall trap was placed ca. 3 m in distance from each experimental dung pad plot (one trap beneath each of eight Q. ilex subsp. ballota trees and one trap beneath each of eight Q. suber trees; 16 pitfall traps in total). These traps were at a minimum distance of 50 m apart (the same distance as between the experimental plots) in order to minimize the possibility of capturing beetles that had moved between plots (Larsen and Forsyth 2005). The pitfall traps were each baited with a 200 g cattle manure ball. These balls were suspended on top of 750 cm3 plastic cups that were buried to ground level. An acetate funnel was inserted at depth 7 cm within the cups in order to prevent escape of the captured specimens (suspended bait grid method, modified from Veiga et al. 1989). A small amount of moist soil was also placed in the bottom of each cup in order to keep the captured specimens alive (according to Favila and Halffter 1997). The pitfall traps were checked every three days and were kept for two weeks. At each sampling, the cup contents were collected and sieved (1 cm2 mesh size) and the captured dung beetles classified according to morphology and size, identified according to Martín Piera and López Colón (2000), recorded, and released back to the field. A few specimens of each type were stored in glass bottles filled with 75% ethanol solution and transported to the laboratory to ensure accurate identification. Only relatively large-sized dung beetles (> 10 mm in size) were considered in this study because of the importance of large body size to enable movement of a large amount of soil (Horgan, 2001).
Effect of cattle manure and dung beetle bioturbation on acorn escape of rodent predation and seedling establishment under livestock exclusion. (Second year trial)
In order to avoid livestock, 32 exclosures (1.4 m2 base area × 1.20 m height) were established in the same stand as in the first year trial. These exclosures were made of iron mesh (mesh size 4 × 10 cm), which allowed access for the rodents but not for mid- or large-sized acorn predators. As in the first year trial, half of the exclosures were placed beneath the canopies of Q. ilex subsp. ballota trees and the other half beneath Q. suber trees. In this case, we established two different positions at which to place the exclosures: (I) beneath the tree canopy border (where conditions are more exposed due to the discontinuous canopy layer characteristic in the dehesas), and (II) at distance 0.5 m from the trunk (where canopy cover is denser and the rodents may be more sheltered). Thus, there were four microhabitats: Q. ilex subsp. ballota border, Q. ilex subsp. ballota trunk, Q. suber border and Q. suber trunk (hereafter, Q. ilex B, Q. ilex T, Q. suber B and Q. suber T), each with eight replicates. Four treatments were applied in the different microhabitats inside the exclosures. Two of these allowed the rodents to access the experimental plots (uncaged treatments) while the other two excluded the rodents (caged treatments) (Schematic representation of the experimental design in the second year in Figure S1).
Uncaged treatments (rodent access allowed)
The uncaged treatments were designed to assess whether cattle manure and the associated dung beetle activity play a role in acorn burial, acorn predation by rodents and seedling establishment. For this, two round plots (diameter 25 cm) were established within each exclosure; one was supplied with a surface layer of fresh cattle manure of 2 cm in depth, while the other received no manure (hereafter, uncaged manured and uncaged unmanured treatments, respectively). Six marked (white-spotted) acorns were added to each of the plots (Q. suber or Q. ilex subsp. ballota acorns, depending on the treatment), placing these on the plot surface. The acorns were supplied in mid-November and monitored monthly until mid-January in order to record persistence (i.e., number of undamaged acorns remaining in the plots either on, or buried below, the surface). Acorn vertical position was also monitored, distinguishing between buried and unburied acorns (i.e. acorns completely covered by soil material that were imperceptible at a glance, and those that were entirely or partially exposed on the plot soil surface, respectively). As in the first year trial, buried acorns were carefully detected by inserting a rod (ca. 6 cm in depth) at regular points into the plots. The treatment conditions were maintained as described for two months. However, as expected, many acorns were predated during this period. Given that we aimed to assess the potential effect of acorn burial on rodent predation and seedling establishment, we continued the trial with some additional manipulation (Figure S1).
The new manipulation consisted of removing all of the remnant acorns from the previous phase and placing new acorns evenly (4 acorns/plot) in mid-January. The number of acorns placed was lower than in November due to the late acorn dropping season (i.e., second half of dropping season, Leiva and Díaz-Maqueda 2016). The new acorns were marked with colored plastic strips (2 × 17 cm) attached to the acorn coats (method modified from Leiva and Vera 2015). In the manured treatment, where plots remained highly disturbed, we artificially buried the acorns 1.5–2 cm under the soil mounds in order to mimic bioturbation-mediated burial. The colored strips were left unburied on the soil surface to indicate acorn position. In the unmanured treatment where the plots remained undisturbed, the acorns were placed on the soil surface. These were monitored monthly to record persistence until the end of May, including careful inspection of the area surrounding the plots to detect potentially moved acorns. At the end of the trial (end of May) the ontogenetic development of propagules remaining in the plots (seedlings with at least two expanded leaves, non-germinated acorns and germinated but subsequently dead epicotyls and roots) was recorded by careful extraction of the remaining undeveloped and buried acorns.
Caged treatments (rodents excluded)
These treatments were applied in a single phase, beginning in mid-November and concluding at the end of May (Figure S1). As with the uncaged treatments, two identical 25 cm diameter plots were established within each exclosure. One plot was manured and the other was not (caged manured and caged unmanured treatment, respectively). After supplying six marked (white spotted) acorns to each plot, the plots were protected against rodents by installing cylindrical cages (30 cm in diameter × 30 cm in height) of wire mesh (mesh size 10 × 10 mm) that were pegged to the ground. The cages were removed at the end of May and the number and ontogenetic development of propagules recorded.
Data analysis
All data analyses were performed using IBM SPSS Statistic v26 software. We applied survival analysis (Kaplan–Meier and log-rank test) to the data pertaining to acorn persistence with no exclusion of livestock or acorn predators (first year trial). These analyses were applied to the whole set of acorns in each treatment (i.e., 64 acorns distributed in eight plots per treatment). Treatment effect (plot surface type: dung pad vs. ground plots) was analyzed for each oak species separately since the treatments were not simultaneously applied. The acorns that remained in the plots at the end of the trial were managed as censored. Temporal changes in plot bioturbation (number of disturbed cells), as well as in dung cover (number of dung covered cells), were analyzed using Friedman tests for k related samples and a Wilcoxon test for two related samples (when it was only possible to compare two dates).
Data from the second year trial were analyzed using GLM-Logistic models. In the uncaged treatment, cumulated values of predated, buried and unburied acorns were used as response variables in the early experimental period (mid-November to mid-January), while microhabitat (i.e., Q. ilex B, Q. ilex T, Q. suber B and Q. suber T) and plot surface type (i.e., manured and unmanured) were the predictor variables. Plot surface type was nested within microhabitat in the model. Acorns were considered to have undergone predation when they were lost from the plots or gnawed, since there is negligible dispersal of acorns by rodents in the area (Leiva and Vera 2015). The GLM-Logistic model was also used to analyze the results after the second experimental manipulation (late phase: mid-January to the end of May). Acorn persistence at the end of May was the response variable and microhabitat and plot surface type were the predictor variables. As in the previous case, plot surface type was nested within microhabitat in the model. The kinetic of acorn persistence was analyzed using a Generalized Linear Mixed Model (GLMM) with monthly acorn persistence used as repeated measures (mid-January to end-May values).
Data pertaining to seedling establishment at the end of the trial were also analyzed using GLM-Logistic models. The number of established seedlings at the end of the trial was the response variable, while microhabitat type and treatment were the predictor variables. Treatment was nested within microhabitat in the model. Only three treatments (uncaged manured, caged manured and caged unmanured) were included since no seedlings were established in the uncaged unmanured treatment.
Results
Effect of cattle dung pads on acorn persistence under livestock presence. Bioturbation and assessment of dung beetle assemblage (First year trial)
Important differences in acorn persistence were observed between the dung pad and ground plots in both Q. ilex subsp. ballota and Q. suber plots (Fig. 1), with faster disappearance of acorns recorded in the ground than in the dung pad plots. For Q. ilex subsp. ballota, the mean acorn persistence values were 7.0 and 1.5 days in dung pad and ground plots, respectively, while in Q. suber these values were 16.1 and 2.0 days, respectively. Accordingly, the Kaplan–Meier survival analysis and log-rank test indicated significant differences among treatments in the two oak species (X2 = 52.19, df = 1, P < 0.001 in Q. ilex subsp. ballota and X2 = 79.33, df = 1, P < 0.001 in Q. suber). However, the final acorn persistence in the dung pad plots was quite high for Q. suber (40% at the end of the trial, Fig. 1), but quite low for Q. ilex subsp. ballota (6.3%).
Cell disturbance (Fig. 2) was overwhelmingly associated with the dung pad plots, with soil mounds and tunnel mouths being the major disturbances observed. No bioturbation was observed in the ground plots during the field trial development. Consequently, only data from the dung pad plots are presented. Soil mounds and tunnel mouths were also found in adjacent, non-experimentally controlled fresh dung pads. Direct sampling (tunnel excavation) in some of the adjacent dung pads revealed the presence of dung beetles in the shaft base, suggesting that coprophilous beetle activity was one of the major bioturbation sources in our dung pad plots.. The number of disturbed cells per plot increased significantly over time in the two oak species plots (X2 = 29.1 and 35.8, P < 0.001, for Q. ilex subsp. ballota and Q. suber, respectively) but the maximum bioturbation was presented in the Q. suber dung pad plots (ca. 40 disturbed cells on average from the total 49 cells). In parallel, the dung-covered cells significantly decreased in number over time, but this decrease was more marked in the Q. suber plots (Z = -3.65, P < 0.001 and X2 = 26.5, P < 0.001 for Q. suber and Q. ilex subsp. Ballota, respectively). These results again suggest the importance of fecal material displacement as a major source of bioturbation in the dung pad plots.
First year trial: Temporal changes in dung cover (mean ± SE bars), expressed as persistence of the dung covered cells (%)and, in bioturbation, expressed as the absolute number of cells presenting disturbance (i.e., soil mounds and tunnel mouths) in dung pad plots under Q. ilex subsp. ballota (a) and Q. suber (b). Different letters denote significant temporal differences (P < 0.05)
The assemblage of dung beetles recorded in this study (i.e., specimens > 10 mm in length) included four species: Typhaeus momus, Ceratophyus hoffmanseggi, Copris hyspanus and Bubas bison (Table 2). The most abundant species was T. momus, with an average of 11.7 ± 2.5 (mean ± SE) cumulated specimens per trap, followed by C. hoffmanseggi with 5.6 ± 1.8. These two species together accounted for 75% of all captured specimens. In contrast, B. bison, with 0.3 ± 0.1 cumulated specimens per trap, was the least abundant species. The species assemblage at the Q. ilex subsp. ballota and the Q. suber pitfall traps was very similar (Table 2), except for a few individuals of B. bison, which were restricted to a few Q. suber traps. However, the Q. suber traps exhibited maximal beetle abundance in all cases, except for the trap Q. ilex subsp. ballota 7, which presented a high abundance of T. momus. Temporal changes in beetle abundance (Table 3) were also evident, with more specimens captured per trap at the beginning of the sampling period than later. These temporal differences were highly significant in the Q. suber traps (X2 = 18.8, P < 0.001), but were small and non-significant in Q. ilex subsp. ballota traps (X2 = 4.1; P < 0.25).
Second year trial. Acorn fate in different treatments
Uncaged treatments (rodents allowed)
Overall, 25.3% of the acorns were predated by rodents in the uncaged treatments by the end of the early experimental phase (i.e., mid-January). The GLM for acorn predation (Table 4a), indicated no significant effect of microhabitat, but a highly significant effect of plot surface type (i.e., manured vs. unmanured). On average, acorn predation in the manured treatment was half (16.7%) that found in the unmanured treatment (33.8%) (Fig. 3). Comparing the effect of plot surface type on different microhabitats, the highest and significant coefficient (β absolute value) was for Q. suber B (Table 4a). In general, the Q. suber microhabitats exhibited higher coefficients than those of Q. ilex subsp. ballota. Acorn burial (Fig. 3), was greater in the manured treatment where 23.4% of the acorns were buried under the soil mounds and galleries that occurred in these plots. Consequently, the GLM model was applied only to the manured plot surface type, while microhabitat was the only predictor variable. Microhabitat had a significant effect on acorn burial (Table 4b), while the model coefficient was only significant for Q. suber B, with the highest acorn burial (Fig. 3). Unburied acorns represented 54 to 71% of the acorns supplied in the different microhabitats; however, the model was non-significant in this case (Table 4c).
Second year trial: Percentages of acorns that were gnawed by rodents or found to be missing from the plots (predation), completely buried due to bioturbation (buried) or remaining on the plot surface (unburied) in: a) manured and b) unmanured plots with rodent access allowed (uncaged treatments). Different letters denote significant difference (P = 0.012) according to pairwise comparisons. Data are cumulated for the period mid-November to mid-January, before additional manipulation. B = border microhabitats; T = microhabitats near the trunk
In the uncaged treatments, the results from the late experimental phase (beginning in mid- January, after new manipulation, and finishing at the end of May: Figure S1) were also similar to these from the first experimental phase (Fig. 4 and Table 4d). Overall, 38.8% of the acorns persisted to the end of the trial in the different microhabitats, but persistence in the manured treatment (70.8%) was ca. ten times higher than in the unmanured treatment (6.8%). As in the previous case, the effect of microhabitat on final acorn persistence (Table 4d), was non-significant but the effect of plot surface type was highly significant. The model coefficients were positive and significant in all microhabitats. Temporal changes in acorn persistence were tested in the manured treatment (Table 5) but not in the unmanured treatment due to the high number of zero values (66% of plots exhibited zero values by February and this number increased with time). Consequently, very steep decreases in acorn persistence were observed over time in the unmanured treatment (Fig. 4) compared to only slight decreases in the manured treatment. This latter treatment presented some kinetic differences among microhabitats, despite the generally low temporal changes in acorn persistence. In the microhabitats located under the canopy border, the temporal changes were highly significant (Table 5a), and more acorns persisted during the first two or three months compared to later, as indicated by the significant model coefficients for January and February (May being the reference category) in Q. ilex B, and for January, February and March in Q. suber B (Table 5b). In the microhabitats located close to the tree trunk, the temporal changes were non-significant (Q. ilex T, Table 5a) or were significant in January only (Q. suber T, Table 5b), indicating that the acorns underwent rapid predation and subsequently remained unchanged for the remainder of the study period.
Second year trial: Monthly persistence of the acorns in the uncaged treatments (mean ± SE bars), under manured (where the acorns were also buried: burial) = diamonds and unmanured (where the acorns were not buried: non-burial) = squares. Values in May are the reference categories for kinetic changes according to the GLMM, with black color (manured treatments) indicating significant model coefficients. Values in May are also the response variable included in the GLM-Logistic model. Q. ilex = Q. ilex subsp. ballota
Caged treatments (rodents excluded)
Regarding both the manured and unmanured caged treatments, all of the acorns persisted to the end of the trial (100% persistence), as was expected under rodent exclusion. However, the caged manured treatment exhibited a high level of bioturbation and relatively large-sized (> 1 cm in length) dung beetles were repeatedly observed inside the cages. The cages therefore did not exclude the dung beetles, which probably accessed the plots via galleries excavated under the edge of the cages.
Second year trial. Final seedling establishment
Important differences were found in seedling establishment between microhabitats and treatments at the end of the trial (Fig. 5). In general, there was much greater seedling establishment in the uncaged manured (overall, 34%) and caged manured (overall, 26%) than in the caged unmanured (overall, 3.7%) treatments. No seedlings were established in the uncaged unmanured treatment. The predictor variables microhabitat and treatment were both significant (X2 = 12.67 P = 0.005 and 47.7; P < 0.001 for microhabitat and treatment, respectively). The Q. suber microhabitats generally presented higher seedling establishment than those of Q. ilex subsp. ballota (Fig. 5). The model coefficients for the manured treatments (uncaged and caged) (Table 6) were positive and significant in all microhabitats, indicating a positive effect relative to the reference category of caged unmanured. However, the caged manured treatment in Q. ilex B was an exception, with no significant coefficient.
Second year trial: Final seedling establishment (mean ± SE values) in the different treatments (except for the uncaged unmanured, in which no seedlings were established). * = acorn burial applied. Different letters denote significat differences between michrohabitats (P < 0.05) according to the pairwise comparisons. Q. ilex = Q. ilex subsp. ballota
Discussion
The results of the different trials included in this study consistently demonstrate a high and positive effect of spontaneous or experimental cattle manure addition on the survival of acorns that drop onto the manure, as well as on the subsequent establishment of oak seedlings, in the two major oak species present in the dehesas. However, different mechanisms, acting simultaneously or sequentially, appear to influence these results.
Dung pad effects on acorn persistence with access permitted to livestock and wild acorn predators (first year trial)
The positive, significant effect of cattle dung pads on acorn persistence (Fig. 1) found in the first year trial, especially in the Q. suber plots, support the well-known behavior of cattle and other large herbivores, which avoid feeding in the immediate vicinity of feces (Moe and Wegge 2008; Gillet et al. 2010), thus reducing the risk of parasite infection (Hutchings et al. 1999). The same behavior could occur with the dung-contaminated experimental acorns placed on the dung pads. However, we cannot overlook the fact that a high level of bioturbation was found in these dung pad plots (Fig. 2) where the acorns were found to persist for longer than they did on the ground.
Dung beetle abundance, composition and nesting behavior type (first year trial)
Dung beetle abundance in the proximity of the dung pad plots (Table 2) exhibited the same pattern of variation as the levels of plot bioturbation (Fig. 2) and acorn persistence (Fig. 1), with these three variables being high in the Q. suber dung pad plots but quite low in those of Q. ilex subsp. ballota. These results suggest that, in addition to the potential rejection of dung-contaminated acorns by cattle and wild acorn predators, bioturbation could protect the acorns through passive burial in the plots. The dung beetle species found in this study (Typhaeus momus, Ceratophyus hoffmanseggi, Copris hyspanus and Bubas bison: Table 2) are all paracoprids (tunnelers) (Martín Piera and López Colón 2000), which excavate tunnels beneath or beside dung pats and move considerable quantities of dung (Mittal 1993; Milotić et al. 2017). The soil mounds and tunnel mouths observed in the dung pad plots, and the dung beetle specimens repeatedly found in the shaft bases, suggest the importance of these relatively large-sized (> 1 cm) beetle species in terms of generating bioturbation in the plot. Dung beetle body size and mass have usually been considered as surrogate traits associated with dung burial, which in turn generates bioturbation, although it has been shown that other dung beetle traits are also important (de Castro-Arrazola et al. 2020). Nevertheless, we cannot disregard the contribution of smaller dung beetles (e.g. the Aphodiinae, Dellacasa et al. 2001), or that of other groups of edaphic fauna such as the earthworms (Milotić et al. 2017; Bacher et al. 2018).
The aforementioned differences in dung beetle abundance are probably related to temporal sampling differences between the Q. ilex subsp. ballota and Q. suber dung pad plots. Although the four species recorded in this study are seasonal, with an activity period from autumn to spring (Martin Piera and López Colón 2000), maximal population density can vary among species (Agoglitta et al. 2012). For instance, in our case, T. momus tends to be more active towards winter than in the autumn (Lobo 1992). Furthermore, a more sandy soil such as that found at the Q. suber pitfall traps (see Ibañez et al. 2020 for soils descriptions in the same study site) is often more favorable for dung beetles than the heavier soil found at the Q. ilex subsp. ballota pitfall traps), particularly for tunneler dung beetle species (Sowig 1995, Davies 1996; Osberg et al. 2012).
Manure reduced acorn predation by rodents through acorn burial
Rodents were the only acorn consumers allowed to enter the uncaged plots during the second year trial. These animals were therefore responsible for the significantly higher levels of acorn predation in the unmanured plots (ca. 34%) compared to those found in the manured treatment (ca. 17%) during the early experimental phase. In addition, the more conspicuous protective effect of manure on the Q. suber acorns compared to those of Q. ilex subsp ballota (higher model coefficient absolute values in the former species, Table 4a) agrees with the differential preferences of mice regarding the acorns of these two oak species. In laboratory experiments, Del Arco et al. (2018) demonstrated that the mice species present in our study site, A. sylvaticus and M. spretus, exhibit a higher preference for Q. ilex subsp. ballota acorns than for those of Q. suber.
Importantly, during the early experimental phase, we found that 23.4% of the acorns were completely buried as a result of bioturbation (Fig. 3). To our knowledge, this is the first time that this passive acorn burial process has been experimentally recorded. However, it should be distinguished from the active vertical displacement by dung beetles of the seeds embedded in herbivorous dung (Vander Wall and Longland 2004; Santos-Heredia et al 2010; Griffiths et al. 2016; Braga et al. 2017), and from the direct acorn burial carried out by some dung beetle species in the genera Thorectes (T. lusitanicus, T. baraudi) and the tropical genera Mycotrupe (M. lethrorides) which can also partially consume the acorns (Verdú et al. 2007 and 2010). Thorectes species were not found in our pitfall traps.
The overwhelming effect of plot surface type (manured vs. unmanured) on acorn persistence in our uncaged treatments during the late experimental phase (Fig. 4 and Table 4d) is inseparable from the acorn burial effect (since artificial burial was applied in the late experimental phase to mimic the bioturbation observed in the early phase (Figure S1). This positive effect of acorn burial in terms of decreased acorn predation has been found in several studies (Johnson et al. 2019; Pulido and Díaz 2005; Gómez 2004). Although mice were the most important wild consumers of acorns, and their activity was prevented by acorn burial in our second year trial, similar results were found in a study where the major acorn consumers were the red deers (Cervus elaphus) (Herrera 1995). We hypothesize that at similar results might be found with other important acorn consumers, including birds (Columba palumbus, Grus grus, Garrulus glandarius, Pica pica) and mid-sized mammals (Meles meles, Oryctolagus cuniculus) since burial is recognized as a general protective mechanism against seed predation (Vander Wall and Longland 2004).
Regarding the microhabitat effect, it has been found that habitat structure (fragmented and open vs. non-fragmented forests) is a very important driver in decision-making regarding foraging in rodents (Morán-López et al. 2015). The slower acorn decrease found in this study under the canopy border microhabitats (significant effect of temporal changes and model coefficient for January, February and March: Table 5) compared to the microhabitats near the trunks (no significant temporal changes or significant for a short time only: January) suggests higher rodent predatory activity in the sheltered conditions near the trunks. However, in our case, this was a transient effect, being not significant at the end of the trial (Table 4d).
Seedling establishment at the end of the trial
Cattle manure notably improved seedling establishment in our second year trial (Fig. 5 and Table 6), regardless of whether or not rodents were excluded. These results suggest the importance of cattle manure in reducing the risk of acorn desiccation and viability loss, as well as the previously discussed decreased incidence of predation. Desiccation sensitivity is a characteristic of most temperate oak species, including Q. suber and Q. ilex subsp. ballota (Leiva and Diaz-Maqueda 2016, and references therein). The importance of acorn burial in preserving acorn viability and promoting ontogenetic development has been reported in previous studies (Garcia-Orth and Martínez-Ramos 2007; Ramos-Palacios and Badano 2014). Certainly the application of manure in our trial changes not only the physical characteristics associated with bioturbation but also other soil properties, notably the availability of nutrients (Nichols et al. 2008; Aarons et al. 2009). However, the effect of increased nutrient availability on seedling establishment was probably relatively low since Q. ilex subsp. ballota and Q. suber are large-seeded species with seedlings that depend highly on their cotyledon reserves during early development (Bonfil 1998; Kabeya and Sakai 2003).
Our results regarding seedling establishment were obtained under the experimental conditions applied in the second year study. However, to extrapolate these results to natural field conditions, some corrections must be applied. The artificial 100% acorn burial that we applied in the uncaged manured treatment in the late experimental phase (Figure S1) was much higher than the overall 23.4% acorn burial found in the early phase (Fig. 3). Our recording of acorn burial was conservative and only included the completely buried acorns and excluded those that were only partially buried, which nevertheless could also benefit from improved soil conditions. However, to follow the conservative approach, we can use combined probabilities. Therefore, burial rate x seedling establishment rate provides a seedling establishment ranging from 4.4% to 10.3% in the different microhabitats. These values are still quite high compared to those of oak seedling establishment mediated by wild dispersers that actively bury the acorns. For instance, Gómez et al. (2008) reported 1.3% viability in acorns of Q. ilex following dispersal and caching by rodents, while Martinez-Baroja et al. (Martínez-Baroja et al. 2019) found that 0.6 to 2.4% of Q. ilex acorns cached by magpie (Pica pica) resulted in seedlings, and Pérez-Ramos et al. (2007) reported maximum seedlings establishment values of 6 and 15% for Q. canariensis and Q. suber, respectively, after acorns had been actively dispersed and buried by the balanophagous-coprophagous beetle Thorectes lusitanicus.
Implications for management
Acorns from Q. ilex subsp. ballota and Q. suber, the dominant oak species in the Mediterranean dehesas, have shown that they can benefit from the presence of cattle manure and the associated bioturbation, increasing seedling establishment 4.4 to 26 fold compared to situations in which cattle dung is absent. The open structure of Mediterranean dehesas with scattered oak trees and lack of shrub cover in the understory precludes active acorn dispersal by rodents, which cannot find suitable shelter for acorn caching (Morán-López et al. 2015). The prevention of shrub encroachment by livestock has been considered to indirectly contribute to the lack of oak self-regeneration in dehesas; however, our results indicate that this situation could be partially reversed by proper livestock management such as rotational grazing. For instance, enclosing the animals in a paddock for a short time during the early- to mid-acorn dropping season could give the acorns the opportunity for passive burial, thus decreasing seed desiccation risk and escaping predation by rodents. These processes would enhance seedling establishment under the oak canopies, a microhabitat that is favorable for oak seedling survival in the seasonally dry and hot Mediterranean environment (Caldeira et al. 2014). This passive and spontaneous acorn burial is associated with the seed dropping sites and does not necessarily imply expansion of the oak tree population. However, it may contribute to oak population turnover, a key process in Mediterranean dehesas where the lack of new recruits constitutes a bottleneck for long-term persistence.
Data availability
The datasets used during the current study are available from the corresponding author on reasonable request.
References
Aarons SR, O’Connor CR, Hosseini HM, Gourley CJP (2009) Dung pads increase pasture production, soil nutrients and microbial biomass carbon in grazed dairy systems. Nutr Cycl Agroecosyst 84:81–92. https://doi.org/10.1007/s10705-008-9228-5
Agoglitta R, Moreno CE, Zunino M et al (2012) Cumulative annual dung beetle diversity in Mediterranean seasonal environments. Ecol Res 27:387–395. https://doi.org/10.1007/s11284-011-0910-8
Andresen E, Levey DJ (2004) Effects of dung and seed size on secondary dispersal seed predation and seedling establishment of rain forest trees. Oecologia 139(1):45–54. https://doi.org/10.1007/s00442-003-1480-4
Bacher MG, Fenton O, Bondi O et al (2018) The impact of cattle dung pats on earthworm distribution in grazed pastures. BMC Ecol 18:59. https://doi.org/10.1186/s12898-018-0216-6
Bonfil C (1998) The effects of seed size, cotyledon reserves, and herbivory on seedling survival and growth in Quercus rugosa and Q. laurina (fagaceae). Am J Botany 85:79–87
Braga RF, Carvalho R, Andresen E, Anjos DV, Alves-Silva E, Louzada J (2017) Quantification of four different post-dispersal seed deposition patterns after dung beetle activity. J Tropic Ecol 33(6):407–410. https://doi.org/10.1017/S0266467417000335
Carpaneto GM, Mazziotta A, Valerio L (2007) Inferring species decline from collection records: roller dung beetles in Italy (Coleoptera, Scarabaeidae). Divers Distrib 13:903–919. https://doi.org/10.1111/j.1472-4642.2007.00397.x
Costa JC, Martín-Vicente A, Fernández-Alés R, Estirado-Oliet M (2006) Dehesas de Andalucía Caracterización Ambiental. Consejería de Medio Ambiente-Junta de Andalucía, Sevilla
Costa A, Villa S, Alonso P, García-Rodríguez JA et al (2017) Can native shrubs facilitate the early establishment of contrasted co-occurring oaks in Mediterranean grazed areas? JVS 28:1047–1056. https://doi.org/10.1111/jvs.12550
Davies ALV (1996) Seasonal dung beetles activity and dung dispersal in selected South African habitats: implications for pasture improvement in Australia. Agric Ecosyst Environ 58:157–169
deCastro-Arrazola I, Hortal J, Noriega JA, Sánchez-Piñero F (2020) Assessing the functional relationship between dung beetle traits and dung removal, burial, and seedling emergence. Ecology 101(10):e03138
Del Arco JM, Beltrán D, Martínez-Ruíz C (2018) Risk for the natural regeneration of Quercus species due to the expansion of rodent species (Microtus arvalis). Behav Ecol Sociobiol 72:160. https://doi.org/10.1007/s00265-018-2575-6
Dellacasa G, Bordat P, Dellacasa M (2001) A revisional essay of world genus-group taxa of Aphodiinae. Mem. Soc. ent. ital. Genova 79:1–482
Dhondt B, Bossuyt B, Hoffmann M, Bonte D (2008) Dung beetles as secondary seed dispersers in a temperate grassland. Basic Appl Ecol 9:542–549
Duque-Lazo J, Navarro-Cerrillo RM, Gils H, Groen TA (2018) Forecasting oak decline caused by Phytophthora cinnamomi in Andalusia: Identification of priority areas for intervention. Forest Ecol Manag 417:122–136. https://doi.org/10.1016/j.foreco.2018.02.045
Eichhorn MP, Paris P, Herzog F et al (2010) Silvoarable systems in Europe - Past, present and future prospects. Agrofor Syst 67:29–50
Faust C, Eichberg C, Storm C, Schwabe A (2011) Post-dispersal impact on seed fate by livestock trampling—a gap of knowledge. Basic Appl Ecol 12:215–226
FAO (2020) Biodiversity and the livestock sector – Guidelines for quantitative assessment – Version 1. Rome, Livestock Environmental Assessment and Performance Partnership (FAO LEAP). https://doi.org/10.4060/ca9295en
Favila ME, Halffter G (1997) The use of indicator groups for measuring biodiversity as related to community structure and function. Acta Zool Mex (n.s.) 72:1–25
García-Barrón L, Aguilar M, Sousa A (2011) Evolution of annual rainfall irregularity in the southwestof the Iberian Peninsula. Theor Appl Climatol 103:13–26
Garcia-Orth X, Martínez-Ramos M (2007) Seed dynamics of early and late successional tree species in tropical abandoned pastures: seed burial as a way of evading predation. Restor Ecol 16:435–443. https://doi.org/10.1111/j.1526-100X.2007.00320.x
Gillet F, Kholer F, Vandenberghe C, Buttler A (2010) Effect of dung deposition on small-scale patch structure and seasonal vegetation dynamics in mountain pasture. Agric Ecosyst Environ 135:34–41
Gómez JM, Garcia D, Zamora R (2003) Impact of vertebrate acorn- and seedling-predators on a Mediterranean Quercus pyrenaica forest. Forest Ecol Man 180:125–134
Gómez JM (2004) Importance of microhabitat and acorn burial on Quercus ilex early recruitment: non-additive effects on multiple demographic processes. Plant Ecol 172:287–297
Gómez JM, Puerta-Piñero C, Schupp EW (2008) Effectiveness of rodents as local seed dispersers of Holm oaks. Oecologia 155:529–537. https://doi.org/10.1007/s00442-007-0928-3
Gómez JM, Schupp EW, Jordano P (2019) Synzoochory: the ecological and evolutionary relevance of a dual interaction. Biol Rev 94:874–902. https://doi.org/10.1111/brv.12481
Gómez-Casero MT, Galán C, Domínguez-Vilches E (2007) Flowering phenology of Mediterranean Quercus species in different locations (Córdoba, SW Iberian Peninsula). Acta Bot Malac 32:127–146
Griffiths HM, Bardgett RD, Louzada J, Barlow J (2016) The value of trophic interactions for ecosystem function: dung beetle communities influence seed burial and seedling recruitment in tropical forests Proc. R Soc B. https://doi.org/10.1098/rspb.2016.1634
Halffter G, Edmond D (1982) The nesting behavior of dung beetles. An ecological and evolutive approach. Instituto de Ecología. México D.F. 176 pp
Halffter G, Matthews E (1966) The natural history of dung beetles of the subfamily Scarabaeinae (Coleoptera, Scarabaeidae). Folia Entomologica Mexicana 12–14:1–312
Herrera J (1995) Acorn predation and seedling production in a low density population of cork oak (Quercus suber L.). For Ecol Manage 76:197–201
Horgan FG (2001) Burial of bovine dung by coprophagous beetles (Coleoptera: Scarabaeidae) from horse and cow grazing sites in El Salvador. Eur J Soil Biol 37:103–111. https://doi.org/10.1016/S1164-5563(01)01073-1
Hutchings MR, Kyriazakis I, Gordon IJ, Jackson F (1999) Trade of between nutrient intake and fecal avoidance in herbivore foraging decisions: the effect of animal parasite status level on feeding motivation and sward nitrogen content. J Anim Ecol 68:310–323
Ibañez M, Leiva MJ, Chocarro C, Aljazairi S, Ribas À, Sebastià MT (2021) Tree—open grassland structure and composition drive greenhouse gas exchange in holm oak meadows of the Iberian peninsula. Agronomy 11:50. https://doi.org/10.3390/agronomy11010050
Johnson PS, Shifley SR, Roger R, Dey DC, Kabrick JM (2019) Regeneration ecology I. Flowering, fruiting and reproductive characteristics. In: Johnson PS, Shifley SR, Roger R, Dey DC, Kabrick JM (eds). The Ecology and Silviculture of Oaks. 3rd edn CABI
Kabeya D, Sakai S (2003) The role of roots and cotyledons as storage organs in early stages of establishment in Quercus crispula: a quantitative analysis of nonstructural carbohydrates in cotyledons and roots. Ann Bot 92:537–545. https://doi.org/10.1093/aob/mcg165
Larsen TH, Forsyth A (2005) (2005) Trap spacing and transect design for dung beetle biodiversity studies. Biotropica 37:322–325
Leiva MJ, Vera M (2015) Effect of artificial shelters of dead branches on acorn survival and dispersal in shrub-lacking Mediterranean dehesas. New for 46:965–978. https://doi.org/10.1007/s11056-015-9486-4
Leiva MJ, Diaz-Maqueda A (2016) Fast-growing seeds and delayed rodent predatory activity in the seeding season: A combined mechanism to escape and survive rodent predation in Quercus ilex subsp. ballota L. acorns and seedlings. Forest Ecol Manag 380:23–30
Lobo JM (1992) Los Escarabeidos Coprófagos: un grupo de insectos con posibilidades. Zapateri Revta Aragon Ent 1:73–78
Manning P, Slade EM, Beynon SA, Lewis OT (2016) Functionally rich dung beetle assemblages are required to provide multiple ecosystem services. Agric Ecosyst Environ 218:87–94. https://doi.org/10.1016/j.agee.2015.11.007
Martín Piera IF, López Colón JI (2000) Fauna Ibérica vol 14: Coleptera Scarabaeoidea I. 526 pp Madrid, ISBN: 84-00-07997-31
Martínez-Baroja L, Pérez-Camacho L, Villar-Salvador P et al (2019) Massive and effective acorn dispersal into agroforestry systems by an overlooked vector, the Eurasian magpie (Pica pica). Ecosphere 10(12):e02989. https://doi.org/10.1002/ecs2.2989
Milotić T, Quidé S, Van Loo T et al (2017) Linking functional group richness and ecosystem functions of dung beetles: an experimental quantification. Oecologia 183:177–190. https://doi.org/10.1007/s00442-016-3756-5
Miranda CHB (2006). Contribución del escarabajo estercolero africano en la mejoría de la fertilidad del suelo. In: Memórias do Primer Simposio Internacional de Generación de Valor en la Producción de Carne. Universide CES, Medellín, Colombia. pp 187–200
Mittal I (1993) Natural manuring and soil conditioning by dung beetles. Trop Ecology 34:150–159
Moe SR, Wegge P (2008) Effect of deposition of deer dung on nutrient redistribution and on soil and plant nutrients on intensively grazed grasslands in lowland Nepal. Ecol Res 23:227–234
Morán-López T, Fernández M, Alonso CL et al (2015) Effects of forest fragmentation on the oak–rodent mutualism. Oikos 124:1482–1491. https://doi.org/10.1111/oik.02061
Nichols E, Spector S, Louzada J et al (2008) Ecological functions and ecosystem services provided by Scarabaeinae dung beetles. Biol Conserv 141:1461–1474. https://doi.org/10.1016/j.biocon.2008.04.011
Olea L, López-Bellido RJ, Poblaciones M (2005) Europe types of silvopastoral systems in the Mediterranean area: Dehesa. In Mosquera-Losada MR, Rigueiro-Rodríguez A, McAdam J (eds) Silvopastoralism and sustainable land management. Proceedings of an international congress on silvopastoralism and sustainable management held in Lugo, Spain, April 2004 pp 30–35
Osberg DC, Doube BM, Hanrahan SA (2012) Habitat specificity in African dung beetles: the effect of soil type on the survival of dung beetle immatures (Coleoptera Scarabaeidae). Trop Zool 7:1–10. https://doi.org/10.1080/03946975.1994.10539236
Pérez-Ramos IM, Marañón T, Lobo J, Verdú JR (2007) Acorn removal and dispersal by the dung beetle Thorectes lusitanicus: Ecological implications. Ecol Entomol 32:349–356
Pulido FJ, Díaz M (2005) Regeneration of a Mediterranean oak: a whole cycle approach. Ecoscience 12:92–102
Pulido F, McCreary D, Cañellas I, McClaran M, Plieninger T (2013) Oak regeneration: ecological dynamics and restoration techniques. In Campos P et al (eds) Mediterranean Oak Woodland working landscapes. Landscape Series, vol 16. Springer, Dordrecht. pp 3–23. https://doi.org/10.1007/978-94-007-6707-2_5
Ramos-Palacios CR, Badano I (2014) The relevance of burial to evade acorn predation in an oak forest affected by habitat loss and land use changes. Bot Sci 92:299–308
Santos-Heredia C, Andresen E, Zárate DA (2010) Secondary seed dispersal by dung beetles in a Colombian rain forest: Effects of dung type and defecation pattern on seed fate. J of Trop Ecol 26:355–364. https://doi.org/10.1017/S0266467410000192
Sowig P (1995) Habitat selection and offspring survival rate in three paracoprid dung beetles: the influence of soil type and soil moisture. Ecography, 18:147–154. http://www.jstor.org/stable/3682763
Verdú JR, Lobo JM, Numa C, Pérez-Ramos IM, Marañón T et al (2007) Acorn preference by the dung beetle, Thorectes lusitanicus, under laboratory and field conditions. Anim Behav 74:1697–1704
Verdú JR, Casas JL, Lobo JM, Numa C (2010) Dung Beetles Eat Acorns to Increase Their Ovarian Development and Thermal Tolerance. PLoS ONE 5(4):e10114. https://doi.org/10.1371/journal.pone.0010114
Vander Wall SB, Longland WS (2004) Diplochory: are two seed dispersers better than one? Trends Ecol Evol 19:155–161
Veiga CM, Lobo JM, Martín-Piera F (1989) Las trampas pitfall con cebo, sus posibilidades en el estudio de las comunidades coprófagas de Scarabaeoidea (Col.).II.Análisis de efectividad. Revue D´ecologie Du Sol 26:91–109
Acknowledgements
Funding was provided by the Regional Government of Andalucía, Ministry of Economy and Revenues (2017/00000634). Special thanks go to Dehesa de Gato S.L. for their support and provision of facilities. Thanks to our colleagues who collaborated with the field and laboratory work: J. Carmelo del Castillo, Montserrat Corral and Manuel Ojedo. Keith McMillan provided English language editing.
Funding
Open Access funding provided thanks to the CRUE-CSIC agreement with Springer Nature. Partial financial support was provided by the Regional Government of Andalucía, Ministry of Economy and Revenues (2017/00000634).
Author information
Authors and Affiliations
Contributions
MJL conceived the ideas, designed the methodology, collected and analyzed the data, and wrote the manuscript. GS-M designed the methodology, collected and analyzed the data on dung beetles, executed the project and reviewed the manuscript. Both authors contributed critically to the drafts and gave their final approval for publication.
Corresponding author
Ethics declarations
Conflict of interest
The authors declare no conflicts of interest relevant to the content of this article.
Ethical approval
The authors state that this study was conducted without compromising animal welfare.
Additional information
Publisher's Note
Springer Nature remains neutral with regard to jurisdictional claims in published maps and institutional affiliations.
Supplementary Information
Below is the link to the electronic supplementary material.
Rights and permissions
Open Access This article is licensed under a Creative Commons Attribution 4.0 International License, which permits use, sharing, adaptation, distribution and reproduction in any medium or format, as long as you give appropriate credit to the original author(s) and the source, provide a link to the Creative Commons licence, and indicate if changes were made. The images or other third party material in this article are included in the article's Creative Commons licence, unless indicated otherwise in a credit line to the material. If material is not included in the article's Creative Commons licence and your intended use is not permitted by statutory regulation or exceeds the permitted use, you will need to obtain permission directly from the copyright holder. To view a copy of this licence, visit http://creativecommons.org/licenses/by/4.0/.
About this article
Cite this article
Leiva, M.J., Sobrino-Mengual, G. Cattle dung and bioturbation by dung beetles improve oak seedling establishment in Mediterranean silvopastoral ecosystems. New Forests 54, 289–309 (2023). https://doi.org/10.1007/s11056-022-09922-0
Received:
Accepted:
Published:
Issue Date:
DOI: https://doi.org/10.1007/s11056-022-09922-0