Abstract
Sb2Te3 is an endpoint of the GeTe-Sb2Te3 quasi-binary tie-line that represents phase-change alloys widely used in optical and non-volatile phase-change memory devices. In the crystalline form it is also a prototypical topological insulator with a layered structure where covalently bonded quintuple layers are held together by weak van der Waals forces. One of the ways to fabricate a crystalline phase is solid-state crystallization of an amorphous film, whereby the three-dimensional (3D) structure relaxes to the two-dimensional (2D) crystalline phase. The mechanism of the 3D-2D transformation remains unclear. In this work, we performed a study of relaxation processes in thin Sb2Te3 films in both amorphous and crystalline phases. We found that both phases possess two kinds of relaxators (type I and type II), where the type I fragments are identical in the two phases, while the relaxation of type II fragments are shifted to lower temperature in the amorphous phases. The activation energies of the associated relaxation processes and the values of the Havriliak–Negami parameters were determined. The differences between the relaxation processes in the two phases are discussed. The obtained result will contribute to better understanding of the 3D-2D transformation during the crystallization of van der Waals solids.








Similar content being viewed by others
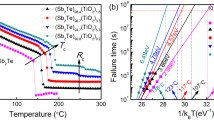
Data availability
The data that support the findings of this study are available from the corresponding author upon reasonable request.
References
K. Rajasekar, L. Kungumadevi, A. Subbarayan, R. Sathyamoorthy, Ionics 14, 69 (2008). https://doi.org/10.1007/s11581-007-0146-3
L.M. Goncalves, C. Couto, P. Alpuim, D.M. Rowe, J.H. Correia, Sens. Actuators 130–131, 346 (2006). https://doi.org/10.1016/j.sna.2005.10.014
J. Boguslawski, J. Sotor, G. Sobon, J. Tarka, J. Jagiello, W. Macherzynski, L. Lipinska, K.M. Abramski, Laser Phys. 24, 105111 (2014). https://doi.org/10.1088/1054-660X/24/10/105111
T. Ohta, J. Optoelectron. Adv. Mater. 3, 609 (2001)
J. Choe, Intel 3D XPoint memory die removed from Intel Optane™ PCM (phase change memory). (TechInsights Inc., 2017), https://www.techinsights.com/blog/intel-3d-xpoint-memory-die-removed-intel-optanetm-pcm-phase-change-memory.
A.M. Farid, H.E. Atyia, N.A. Hegab, Vacuum 80, 284 (2005). https://doi.org/10.1016/j.vacuum.2005.05.003
J.Y. Yang, W. Zhu, X.H. Gao, X.A. Fan, S.Q. Bao, X.K. Duan, Electrochim. Acta 52, 3035 (2007). https://doi.org/10.1016/j.electacta.2006.09.045
A.E. Abken, O.J. Bartelt, Thin Solid Films 403, 216 (2002). https://doi.org/10.1016/S0040-6090(01)01527-9
İY. Erdoğan, Ü. Demir, J. Electroanal. Chem. 633, 253 (2009). https://doi.org/10.1016/j.jelechem.2009.06.010
H.S. Soliman, S. Yaghmour, H.G. Al-Solami, Eur. Phys. J. Appl. Phys. 44, 59 (2008). https://doi.org/10.1051/epjap:2008132
J.E. Boschker, J. Momand, V. Bragaglia, R. Wang, K. Perumal, A. Giussani, B.J. Kooi, H. Riechert, R. Calarco, Nano Lett. 14, 3534 (2014). https://doi.org/10.1021/nl5011492
R. Venkatasubramanian, T. Colpitts, E. Watko, M. Lamvik, N. El-Masry, J. Cryst. Growth 170, 817 (1997). https://doi.org/10.1016/S0022-0248(96)00656-2
K. Ulutas, D. Deger, S. Yakut, J. Phys, J. Phys.Conf. Ser. 417, 012040 (2013). https://doi.org/10.1088/1742-6596/417/1/012040
Y. Saito, S. Hatayama, Y. Shuang, P. Fons, A.V. Kolobov, Y. Sutou, Sci. Rep. 11, 4782 (2021). https://doi.org/10.1038/s41598-020-80301-5
Y. Saito, P. Fons, A.V. Kolobov, K. Mitrofanov, K. Makino, J. Tominaga, S. Hatayama, Y. Sutou, M. Hase, J. Robertson, J. Phys. D Appl. Phys. 53, 284002 (2020). https://doi.org/10.1088/1361-6463/ab850b
S. Havriliak, S. Negami, Polymer 8, 161 (1967). https://doi.org/10.1016/0032-3861(67)90021-3
B.S. Lee, S.G. Bishop, Phase Change Mater. (2009). https://doi.org/10.1007/978-0-387-84874-7_9
R. Diaz-Calleja, Macromolecules 33, 8924 (2000). https://doi.org/10.1021/ma991082i
C. Chen, P. Jost, H. Volker, M. Kaminski, M. Wirtssohn, U. Engelmann, M. Wuttig, Phys. Rev. B 95, 094111 (2017). https://doi.org/10.1103/PhysRevB.95.094111
P. Debye, Ann. Phys. 39, 789 (1912). https://doi.org/10.1002/andp.19123441404
A.K. Jonsher, Dielectric Relaxation in Solids (Chelsea Dielectric Press, London, 1983).
Y. Zheng, M. Xia et al., Nano Res. 9, 3453 (2016). https://doi.org/10.1007/s12274-016-1221-8
T.H. Lee, S.R. Elliott, Adv. Mater. 32, 2000340 (2020). https://doi.org/10.1002/adma.202000340
F.C. Mocanu, K. Konstantinou, J. Mavračić, S.R. Elliott, Phys. Status Solidi RRL 15, 2000485 (2020). https://doi.org/10.1002/pssr.202000485
Funding
The reported study was performed within a Russia-Japan joint project funded by the Russian foundation for Basic Research (project number 20-52-50012) and the Japan Society for the Promotion of Science (project number JPJSBP120204815).
Author information
Authors and Affiliations
Corresponding author
Ethics declarations
Conflict of interest
The authors declare that they have no conflicts of interest.
Additional information
Publisher's Note
Springer Nature remains neutral with regard to jurisdictional claims in published maps and institutional affiliations.
Rights and permissions
About this article
Cite this article
Kononov, A.A., Castro, R.A., Saito, Y. et al. Dielectric relaxation in amorphous and crystalline Sb2Te3 thin films. J Mater Sci: Mater Electron 32, 14072–14078 (2021). https://doi.org/10.1007/s10854-021-05986-4
Received:
Accepted:
Published:
Issue Date:
DOI: https://doi.org/10.1007/s10854-021-05986-4