Abstract
Continuous-wave heterogeneous sonochemical method was developed to synthesize 3D assemblies composed by QDs of metastable (cubic) modification of CdSe with sphalerite structural type. 3D QD assemblies were deposited in thin film form and as bulk precipitates. Structure, surface morphology, optical absorption as well as charge carriers’ and phonon confinement effects were studied in details. The average nanocrystal radius, calculated by the Scherrer and Williamson–Hall methods, diminishes from 2.7 nm for samples synthesized by conventional colloidal chemical route to 2.0 nm for samples synthesized by sonochemical route. Upon post-deposition thermal annealing this value increases to 12.0 nm. QD size decrease is followed by increase of the uni-directional lattice strain and dislocation density (as defined by Hirsch), as well as by decrease of the lattice constant value. Previous findings were justified by analysis of X-ray diffraction peaks shape, which were found to be dominated by the particle size-distribution, instead of non-uniform strain. The energy of the fundamental direct \(\Gamma_{8}^{v} \to \Gamma_{6}^{c}\) interband electronic transition, computed from optical absorption data within parabolic approximation for the dispersion relation, shifts from 2.67 eV for sonochemically deposited samples and 2.08 eV for chemically deposited ones, to 1.77 eV upon post-deposition thermal treatment (close to the bulk value of 1.74 eV). The type of “interband” transitions does not change upon dimensionality reduce. Enhanced band gap energy blue shift upon average QD size decrease is followed by oscillator strength increase. Such trends were rationalized in terms of 3D confinement effects on charge carriers’ motion. Experimentally observed band gap energies agree very well with the predictions of the effective mass model of Brus. The next direct interband electronic transition, from the spin–orbit split component of the valence band to the conduction band, has been detected and analyzed. The 1LO bands in the Raman spectra of chemically and sonochemically deposited samples appear at 206 and 204 cm−1, as compared to the bulk value of 210 cm−1. The extent of homogeneous broadening of the corresponding band is larger in sonochemically deposited samples, indicating enhanced frequency of phonon–phonon collisions in smaller QDs. The observed frequency shifts are dominated by phonon-dispersion terms, instead of lattice contraction.








Similar content being viewed by others
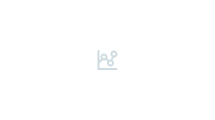
References
D.E. Kim, M.A. Islam, L. Avila, I.P. Herman, J. Phys. Chem. B 107, 6318 (2003)
B.S. Kim, M.A. Islam, L.E. Brus, I.P. Herman, J. Appl. Phys. 89, 8127 (2001)
M.V. Artemyev, A.I. Bibik, L.I. Gurinovich, S.V. Gaponenko, H. Jaschinski, U. Woggon, Phys. Stat. Sol. B 224, 393 (2001)
M.V. Artemyev, U. Woggon, H. Jaschinski, L.I. Gurinovich, S.V. Gaponenko, J. Phys. Chem. B 104, 11617 (2000)
Y. Lin, A. Böker, J. He, K. Sill, H. Xiang, C. Abetz, X. Li, J. Wang, T. Ernrick, S. Long, Q. Wang, A. Balazs, T.P. Russell, Nature 434, 55 (2005)
Z.L. Wang, J.S. Yin, Mater. Sci. Eng., A 286, 39 (2000)
W.U. Dittmer, F.C. Simmel, Appl. Phys. Lett. 85, 633 (2004)
G.U. Kulkarni, P.J. Thomas, C.N.R. Rao, Pure Appl. Chem. 74, 1581 (2002)
C.F. Landes, S. Link, M.B. Mohamed, B. Nikoobakht, M.A. El-Sayed, Pure Appl. Chem. 74, 1675 (2002)
Y.A. Vlasov, N. Yao, D.J. Norris, Adv. Mater. 11, 165 (1999)
E. Rabani, D.R. Reichman, P.L. Geissler, L.E. Brus, Nature 426, 271 (2003)
U. Landman, W.D. Luedtke, Faraday Discuss. 124, 1 (2004)
A.D. Yoffe, Adv. Phys. 50, 1 (2001)
A.D. Yoffe, Adv. Phys. 51, 799 (2002)
C. Burda, X. Chen, R. Narayanan, M.A. El-Sayed, Chem. Rev. 105, 1025 (2005)
R.F. Khairutdinov, Russ. Chem. Rev. 67, 109 (1998)
T. Trinade, P. O’Brien, N.L. Pickett, Chem. Mater. 13, 3843 (2001)
A.K. Pal, A. Mondal, S. Chaudhuri, Vacuum 41, 1460 (1990)
T. Cruszecki, B. Holmström, Solar Energy Mater. Solar Cells 31, 227 (1993)
S. Ericsson, T. Gruszecki, P. Carlsson, B. Holmström, Thin Solid Films 269, 14 (1995)
A. Van Calster, F. Vanfleteren, I. De Rycke, J. De Baets, J. Appl. Phys. 64, 3282 (1988)
N.G. Patel, C.J. Panchal, K.K. Makhijia, Cryst. Res. Technol. 29, 1013 (1994)
V.A. Smyntyana, V. Gerasutenko, S. Kashulis, G. Mattogno, S. Reghini, Sens. Actuators 19, 464 (1994)
B. Bonello, B. Fernandez, J. Phys. Chem. Solids 54, 209 (1993)
J.C. Schottmiller, R.W. Francis, C. Wood, U.S. patent 3,884,688, 1975
R. Lozada-Morales, M. Rubin-Falfán, O. Portillo-Moreno, J. Pérez-Álvarez, R. Hotos-Cabrera, C. Avelino-Flores, O. Zelaya-Angel, O. Guzmán-Mandujano, P. del Angel, J.L. Martinez-Montes, Baños-López. J. Electrochem. Soc. 146, 2546 (1999)
S. Gorrer, G. Hodes, J. Phys. Chem. 36, 4215 (1987)
R.C. Kainthla, D.K. Pandya, K.L. Chopra, J. Electrochem. Soc. 127, 277 (1980)
R.C. Kainthla, D.K. Pandya, K.L. Chopra, J. Electrochem. Soc. 129, 99 (1980)
N. Samarth, H. Luo, J.K. Furdyone, S.B. Quadvi, Y.R. Lee, A.K. Ramdas, N. Otsuka, Appl. Phys. Lett. 54, 2162 (1989)
L.P. Colletti, B.H. Flowers, J.L. Stickney, J. Electrochem. Soc. 145, 1442 (1998)
T. Hayashi, R. Saeki, T. Suzuki, M. Fukaya, Y. Ema, J. Appl. Phys. 38, 5719 (1990)
G.M. Fofanov, G.A. Kitaev, Russ. J. Inorg. Chem. 14, 322 (1969)
O. Portillo-Moreno, R. Lozda-Morales, M. Rubín-Falfán, J.A. Pérez-Álvarez, O. Zelaya-Angel, L. Baños-Lórez, J. Phys. Chem. Solids 61, 1751 (2000)
B. Pejova, A. Tanuševski, I. Grozdanov, J. Solid State Chem. 172, 381 (2003)
K.S. Suslick, G.J. Price, Annu. Rev. Mater. Sci. 29, 295 (1999)
K.S. Suslick, in Encyclopedia of Physical Science and Technology, 3rd edn., ed. by R.A. Meyers (Academic Press, Inc., San Diego, 2001), pp. 1–22
T.G. Leighton, The Acoustic Bubble (Academic Press, London, 1994)
Y.T. Didenko, K.S. Suslick, Nature 418, 394 (2002)
W.B. McNamara, Y.T. Didenko, K.S. Suslick, Nature 401, 772 (1999)
G. Dantsin, K.S. Suslick, J. Am. Chem. Soc. 122, 5214 (2000)
W.B. McNamara, Y.T. Didenko, K.S. Suslick, J. Phys. Chem. B 107, 7303 (2003)
T.J. Mason, J.P. Lorimer, Sonochemistry: Theory, Applications and Uses of Ultrasound in Chemistry (Wiley, Chichester, UK, 1988)
A. Gedanken, Ultrason. Sonochem. 11, 47 (2004)
B. Pejova, I. Grozdanov, Thin Solid Films 515, 5203 (2007)
B. Pejova, A. Tanuševski, I. Grozdanov, J. Solid State Chem. 177, 4785 (2004)
B. Pejova, I. Grozdanov, J. Solid State Chem. 158, 49 (2001)
Microsoft Excel 1985–2003, Microsoft Corporation
Microcal Origin, Version 5.0, 1991–1997 Microcal Software, Inc
S. Gorer, G. Hodes, J. Phys. Chem. 98, 5338 (1994)
B. Pejova, in Progress in Solid State Chemistry Research, ed. by R.W. Buckley (Nova Science Publishers, New York, 2007), pp. 55–115
A.C. Zettlemoyer, Nucleation (Marcel Dekker, New York, 1969)
M. Ohring, The Materials Science of Thin Films (Academic Press, New York, 1992)
N.N. Greenwood, A. Earnshaw, Chemistry of the Elements, 2nd edn. (Buterworth & Heinemann, London, 1997)
S. Xu, H. Wang, J.-J. Zhu, H.-Y. Chen, J. Cryst. Growth 234, 263 (2002)
J. Zhu, Y. Koltypin, A. Gedanken, Chem. Mater. 12, 73 (2000)
Y. Xie, X. Zheng, X. Jiang, J. Lu, L. Zhu, Inorg. Chem. 41, 387 (2002)
JCPDS-International Center for Diffraction Data 75-1462
B. Pejova, I. Bineva, J. Phys. Chem. C 117, 7303 (2013)
B. Pejova, B. Abay, J. Phys. Chem. C 115, 23241 (2011)
B. Pejova, I. Grozdanov, D. Nesheva, A. Petrova, Chem. Mater. 20, 2551 (2008)
H. Hofmeister, D. Nesheva, Z. Levi, S. Hopfe and S. Matthias, in Proceeding of the EUREM 12, Brno, ed. by C.L. Frank, F. Ciampor, Czechoslovak Society for Electron Microscopy, Brno (2000), p. 365
S. Enzo, G. Fagherazzi, A. Benedetti, S. Polizzi, J. Appl. Cryst. 21, 536 (1988)
A. Benedetti, G. Fagherazzi, S. Enzo, M. Battagliarin, J. Appl. Cryst. 21, 543 (1988)
M.T. Weller, Inorganic Materials Chemistry (Oxford University Press, Oxford, 1997)
G.K. Williamson, W.H. Hall, Acta Met. 1, 22 (1955)
P. Atkins, J. De Paula, Atkins’ Physical Chemistry, 8th edn. (Oxford University Press, Oxford, 2006)
P.B. Hirsch, Prog. Math. Phys. 6, 236 (1956)
M.A.S.M. Yunos, Z.A. Talib, W.M.M. Yunus, J. Chem. Eng. Mater. Sci. 2, 103 (2011)
A. Baldereshi, N.G. Lipari, Phys. Rev. B 3, 439 (1971)
S. Adachi, Phys. Rev. B 12, 9569 (1991)
N. Chestnoy, R. Hull, L.E. Brus, J. Chem. Phys. 85, 2237 (1986)
B. Pejova, J. Solid State Chem. 181, 1961 (2008)
C.F. Klingshirn, Semiconductor Optics (Springer, Berlin, 1997)
C.R. Kagan, C.B. Murray, M. Nirmal, M.G. Bawendi, Phys. Rev. Lett. 76, 1517 (1996)
C.R. Kagan, C.B. Murray, M.G. Bawendi, Phys. Rev. B 54, 8633 (1996)
F. Gindele, R. Westphäling, U. Woggon, L. Spanhel, V. Ptatschek, Appl. Phys. Lett. 71, 2181 (1997)
B. Pejova, Semicond. Sci. Technol. 29, 045007 (2014)
B. Pejova, J. Solid State Chem. 207, 147 (2013)
L.E. Brus, J. Chem. Phys. 80, 4403 (1984)
L.E. Brus, J. Chem. Phys. 90, 2555 (1986)
M.L. Steigerwald, L.E. Brus, Annu. Rev. Mater. Sci. 19, 471 (1989)
M.L. Steigerwald, L.E. Brus, Acc. Chem. Res. 23, 183 (1990)
Y. Wang, A. Suna, W. Mahler, R. Kasowski, J. Chem. Phys. 87, 7315 (1987)
A.B. Novoselova (ed.), Physical and Chemical Properties of Semiconductors—Handbook (Wiley, Moscow, 1978)
B. Pejova, I. Grozdanov, Mater. Chem. Phys. 90, 35 (2005)
O.I. Micic, S.P. Ahrenkiel, A.J. Nozik, Appl. Phys. Lett. 78, 4022 (2001)
B.S. Kim, L. Avila, L.E. Brus, I.P. Herman, Appl. Phys. Lett. 76, 3715 (2000)
Y. Wang, N. Herron, J. Phys. Chem. 95, 525 (1991)
P.Y. Yu, Solid State Comm. 19, 1087 (1976)
C. Trallero-Giner, A. Debernardi, M. Cardona, E. Menéndez-Proupin, A.I. Ekimov, Phys. Rev. B 57, 4664 (1998)
B. Pejova, J. Phys. Chem. C 177, 19689 (2013)
A.V. Gomonnai, YuM Azhniuk, V.O. Yukhymchuk, M. Kranjčec, V.V. Lopushansky, Phys. Statut. Solidi B 239, 490 (2003)
G. Scamarcio, M. Lugará, D. Manno, Phys. Rev. B 45, 13792 (1992)
Y.-N. Hwang, S. Shin, H.L. Park, S.-H. Park, U. Kim, H.S. Jeong, E.-J. Shin, D. Kim, Phys. Rev. B 54, 15120 (1996)
E.S.F. Neto, N.O. Dantas, S.W. da Silva, P.C. Morais, M.A. Pereira-da-Silva, J. Raman Spectrosc. 41, 1302 (2010)
M.P. Chamberlain, C. Trallero-Giner, M. Cardona, Phys. Rev. B 51, 1680 (1995)
Acknowledgments
This study has been supported under the bilateral agreement between the Bulgarian Academy of Sciences and Macedonian Academy of Sciences and Arts, project “Investigation of the surface morphology of nanostructured thin films by scanning probe microscopy”.
Author information
Authors and Affiliations
Corresponding author
Rights and permissions
About this article
Cite this article
Pejova, B., Bineva, I. Sonochemically assisted colloidal route to CdSe quantum dot assemblies: an alternative way to further fine-tune the size-dependent properties. J Mater Sci: Mater Electron 27, 10600–10615 (2016). https://doi.org/10.1007/s10854-016-5155-4
Received:
Accepted:
Published:
Issue Date:
DOI: https://doi.org/10.1007/s10854-016-5155-4