Abstract
The fabrication of niobium-based devices requires precision and reliability and, consequently, the characterization and description of their mechanical properties. However, there are few studies in the literature on mechanical properties characterization, even less so at the nanoscale, where new technologies require this knowledge. In this work, we study the dependence of the morphology, nanomechanical properties, and friction of niobium films on the pulsed frequency during DC sputtering. Niobium films without oxide in their bulk and bcc crystal structure were deposited at room temperature on Si (100) substrate under sputtering pulse frequencies of 20, 100, and 350 kHz. As the pulsed frequency increased, the morphology changed from granular to flake-like, indicating a change in the atomic accommodation due to the increase in the niobium ion energy during the plasma sputtering, which resulted in an increase in the elastic modulus of films. Elastic modulus maps into an area of 1 µm × 1 µm revealed local variations for films with flake-like morphology. Increasing the pulsed frequency in plasma sputtering at 100 and 350 kHz resulted in films with higher elastic modulus flakes and higher attenuation of the ultrasonic wave during nanomechanical mapping, which was related to dissipative forces due to lateral friction, while the granular morphology and lower elastic modulus of films deposited at 20 kHz was associated with friction at interfaces.
Graphical abstract








Similar content being viewed by others
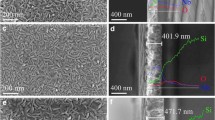
Data availability
The raw data can be accessed on request from the corresponding author.
References
Bagheri S, Strohfeldt N, Ubl M, Berrier A, Merker M, Richter G, Siegel M, Giessen H (2018) Niobium as alternative material for refractory and active plasmonics. ACS Photonics 5:3298–3304. https://doi.org/10.1021/acsphotonics.8b00530
Wilde S, Valizadeh R, Malyshev OB, Stenning GBG, Sian T, Chesca B (2018) dc magnetometry of niobium thin film superconductors deposited using high power impulse magnetron sputtering. Phys Rev Accel Beams 073101:1–12. https://doi.org/10.1103/PhysRevAccelBeams.21.073101
Seifried F, Leiste H, Schwaiger R, Ulrich S, Seifert HJ, Stueber M (2018) Structure, morphology and selected mechanical properties of magnetron sputtered (Mo, Ta, Nb) thin films on NiTi shape memory alloys. Surf Coat Technol 347:379–389. https://doi.org/10.1016/j.surfcoat.2018.05.014
Li M, Pu G, Luo Y, Ye Z, Wei J, Chen S, Wu A, Yang L (2021) The investigation of chemical vapor deposited copper-based niobium films. Mater Res Express 8:046402–046415
Ramana CV, Makeswaran N, Zade V, Das D, Tan S, Xu S, Beyerlein IJ (2022) Fabrication and characterization of high-quality epitaxial nanocolumnar Niobium films with abrupt interfaces on YSZ(001). Phys Chem C. https://doi.org/10.1021/acs.jpcc.1c08738
Bagheri S, Zgrabik CM, Gissibl T, Tittl A, Sterl F, Walter R, De Zuani S, Berrier A, Stauden T, Hu EL, Giessen H (2015) Large-area fabrication of TiN nanoantenna arrays for refractory plasmonics in the mid- infrared by femtosecond direct laser writing and interference lithography [Invited ]. Opt Mater Express 5:7998–8006. https://doi.org/10.1364/OME.5.002625
Guler U, Technologies R, Naik G, Kildishev AV (2012) Performance analysis of nitride alternative plasmonic materials for localized surface plasmon applications Performance analysis of nitride alternative plasmonic materials for localized surface plasmon applications. Appl Phys B Lasers Opt 107:5708–5713. https://doi.org/10.1007/s00340-012-4955-3
West PR, Ishii S, Naik GV, Emani NK, Shalaev VM (2010) Searching for better plasmonic materials. Laser Photon Rev 808:795–808. https://doi.org/10.1002/lpor.200900055
Kurter C, Abrahams J, Shvets G, Anlage SM (2013) Plasmonic scaling of superconducting metamaterials. Phys Rev 180510:1–5. https://doi.org/10.1103/PhysRevB.88.180510
Skliarova H, Azzolini O, Cherenkova-Dousset O, Johnson RR, Palmieri V (2014) Niobium-based sputtered thin films for corrosion protection of proton-irradiated liquid water targets for [18F] production. J Phys D Appl Phys 47:45306–45317. https://doi.org/10.1088/0022-3727/47/4/045306
Berti G, Torres-Castanedo CG, Goronzy DP, Bedzyk MJ, Hersam MC, Kopas C, Marshall J, Iavarone M (2023) Scanning tunneling microscopy and spectroscopy characterization of Nb films for quantum applications. Appl Phys Lett 122:192605–192611. https://doi.org/10.1063/5.0145090
Gontad F, Lorusso A, Panareo M, Monteduro AG, Maruccio G (2015) Nanomechanical and electrical properties of Nb thin films deposited on Pb substrates by pulsed laser deposition as a new concept photocathode for superconductor cavities. Nucl Instrum Methods Phys Res A 804:132–136. https://doi.org/10.1016/j.nima.2015.09.064
Audronis M, Leyland A, Kelly PJ, Matthews A (2006) The effect of pulsed magnetron sputtering on the structure and mechanical properties of CrB2 coatings. Surf Coat Technol 201:3970–3976. https://doi.org/10.1016/j.surfcoat.2006.08.006
Albrecht G, Ubl M, Kaiser S, Giessen H, Hentschel M (2018) Comprehensive study of plasmonic materials in the visible and near- infrared: linear, refractory, and nonlinear optical properties. ACS Photonics 5:1058–1067. https://doi.org/10.1021/acsphotonics.7b01346
Park JH, Han SE, Nagpal P, Norris DJ (2016) Observation of thermal beaming from tungsten and molybdenum Bull’s eyes. ACS Photonics 3:494–500. https://doi.org/10.1021/acsphotonics.6b00022
Nečas D, Klapetek P (2012) Gwyddion: an open-source software for SPM data analysis. Cent Eur J Phys 10:181–188. https://doi.org/10.2478/s11534-011-0096-2
Stan G, Solares SD (2014) Frequency, amplitude, and phase measurements in contact resonance atomic force microscopies. Beilstein J Nanotechnol 5:278–288. https://doi.org/10.3762/bjnano.5.30
Flores-Ruiz FJ, Espinoza-Beltrán FJ, Diliegros-Godines CJ, Siqueiros JM, Herrera-Gómez A (2016) Atomic force acoustic microscopy: influence of the lateral contact stiffness on the elastic measurements. Ultrasonics 71:271–277. https://doi.org/10.1016/j.ultras.2016.07.003
Rabe U (2006) Atomic force acoustic microscopy. In: Bhushan B, Fuchs H (Eds), Appl. Scanning Probe Methods II Scanning Probe Microsc. Tech., Springer Berlin Heidelberg, Berlin, Heidelberg, pp 37–90. https://doi.org/10.1007/3-540-27453-7_2
Espinoza-Beltrán FJ, Geng K, Muñoz Saldaña J, Rabe U, Hirsekorn S, Arnold W (2009) Simulation of vibrational resonances of stiff AFM cantilevers by finite element methods. New J Phys 11:083034–083056. https://doi.org/10.1088/1367-2630/11/8/083034
Garduño-Medina A, Muñoz-Pineda E, Vázquez-Delgado MA, Garcia-Vazquez V, Espinosa-Luna R, Flores-Ruiz FJ (2021) AFM measurement of the cuticle of the orchid bee Euglossa sp.: elastic properties under dehydrated and hydrated conditions. Measurement 185:110045–110060. https://doi.org/10.1016/j.measurement.2021.110045
Taleb S, Badillo M, Flores-Ruiz FJ, Acuautla M (2023) From synthesis to application: high-quality flexible piezoelectric sensors fabricated from tetragonal BaTiO3/ P(VDF-TrFE) composites. Sens Actuators A Phys 361:114585–114596. https://doi.org/10.1016/j.sna.2023.114585
Vlcek J, Pajdarov AD, Musil J (2004) Pulsed dc magnetron discharges and their utilization in plasma surface engineering. Plasma Phys 44:426–436. https://doi.org/10.1002/ctpp.2004100
Bradley JW, Backer H, Aranda-Gonzalvo Y, Kelly PJ, Arnell RD (2002) The distribution of ion energies at the substrate in an asymmetric bi-polar pulsed DC magnetron discharge. Plasma Sour Sci Technol 11:165–174
Ma Q, Rosenberg RA (2003) Angle-resolved X-ray photoelectron spectroscopy study of the oxides on Nb surfaces for superconducting r. f. cavity applications. Appl Surf Sci 206:209–217
Ma Q, Ryan P, Freeland JW, Rosenberg RA (2004) Thermal effect on the oxides on Nb (100) studied by synchrotron-radiation X- ray photoelectron spectroscopy. J Appl Phys 96:7675–7680. https://doi.org/10.1063/1.1809774
Sharahi HJ, Janmaleki M, Tetard L, Kim S, Sadeghian H, Verbiest GJ (2021) Acoustic subsurface-atomic force microscopy: three- dimensional imaging at the nanoscale. J Appl Phys 129:030901–030922. https://doi.org/10.1063/5.0035151
Rabe U, Kopycinska-Müller M, Hirsekorn S (2013) Atomic force acoustic microscopy. In: Acoust Scanning Probe Microsc, pp 315–350. https://doi.org/10.1007/978-3-642-27494-7
Khamseh S, Alibakhshi E, Ramezanzadeh B, Sari MG, Nezhad AK (2020) Developing a graphite like carbon: niobium thin film on GTD-450 stainless steel substrate. Appl Surf Sci 511:145613–145623. https://doi.org/10.1016/j.apsusc.2020.145613
Hurley DC, Shen K, Jennett NM, Turner JA (2003) Atomic force acoustic microscopy methods to determine thin-film elastic properties. J Appl Phys 94:2347–2354. https://doi.org/10.1063/1.1592632
Xu Z, Yate L, Qiu Y, Aperador W, Coy E, Jiang B, Moya S, Wang G, Pan H (2019) Potential of niobium-based thin films as a protective and osteogenic coating for dental implants: the role of the nonmetal elements. Mater Sci Eng C 96:166–175. https://doi.org/10.1016/j.msec.2018.10.091
Li X, Cao WH, Tao XF, Ren LL, Zhou LQ, Xu GF (2016) Structural and nanomechanical characterization of niobium films deposited by DC magnetron sputtering. Appl Phys A 122:505–511. https://doi.org/10.1007/s00339-016-9990-1
Zgheib E, Alhussein A, Slim MF, Khalil K, François M (2019) Multilayered models for determining the Young’s modulus of thin films by means of Impulse Excitation Technique. Mech Mater 137:103143–103160. https://doi.org/10.1016/j.mechmat.2019.103143
Kopycinska-Müller M, Striegler A, Köhler B, Wolter K-J (2011) Mechanical characterization of thin films by use of atomic force acoustic microscopy. Adv Eng Mater 13:312–318. https://doi.org/10.1002/adem.201000245
Liu Y, Varghese S, Ma J, Yoshino M, Lu H, Komanduri R (2015) Orientation effects in nanoindentation of single crystal copper. Int J Plast 24:1990–2015. https://doi.org/10.1016/j.ijplas.2008.02.009
Lin J, Wu ZL, Zhang XH, Mishra B, Moore JJ, Sproul WD (2009) A comparative study of CrNx coatings synthesized by dc and pulsed dc magnetron sputtering. Thin Solid Films 517:1887–1894. https://doi.org/10.1016/j.tsf.2008.09.093
Acknowledgements
The authors acknowledge CONAHCyT for the grants received through the projects FOINS-CONACyT 2016-01-2488, CF-2023-I-1429, and VIEP-BUAP projects ID:00169 and ID:00224. The authors thank Dr. Ulises Salazár Kuri and the XRD-IFUAP Laboratory for their support in the XRD measurements.
Author information
Authors and Affiliations
Contributions
JGM was involved in writing—original draft, visualization, conceptualization, investigation. JRB, OCM, and AEG helped in validation, investigation, data curation. FJFR contributed to funding acquisition, visualization, investigation, supervision.
Corresponding author
Ethics declarations
Competing interest
The authors declare that they have no known competing financial interests or personal relationships that could have appeared to influence the work reported in this paper. On behalf of all authors, the corresponding author states that there is no conflict of interest.
Ethical approval
This manuscript does not contain any human or animal experiments.
Additional information
Handling Editor: M. Grant Norton.
Publisher's Note
Springer Nature remains neutral with regard to jurisdictional claims in published maps and institutional affiliations.
Rights and permissions
Springer Nature or its licensor (e.g. a society or other partner) holds exclusive rights to this article under a publishing agreement with the author(s) or other rightsholder(s); author self-archiving of the accepted manuscript version of this article is solely governed by the terms of such publishing agreement and applicable law.
About this article
Cite this article
Medrano, J.G., Raboño-Borbolla, J., Cortazar-Martínez, O. et al. Nanomechanical properties of Nb films deposited by pulsed frequency magnetron sputtering. J Mater Sci 58, 14556–14569 (2023). https://doi.org/10.1007/s10853-023-08928-z
Received:
Accepted:
Published:
Issue Date:
DOI: https://doi.org/10.1007/s10853-023-08928-z