Abstract
A facile synthetic route for preparing silver-doped maghemite (Ag–γ-Fe2O3) nanocomposite via a modified co-precipitation method was developed. The prepared magnetic nanocomposite was characterized by means of thermal analysis, transmission electron microscope, X-Ray diffraction, vibrating sample magnetometer and Fourier transform infrared techniques. The characterization results showed that the prepared Ag–γ-Fe2O3 nanocomposite is nanocrystalline and 6–8 nm in size with superparamagnetic behavior. The synthesized Ag–γ-Fe2O3 nanocomposite showed exceptional catalytic activities towards reduction of nitroaromatic compounds with specific activities parameters of 1441.7 and 904.2 s− 1 gAg−1 for both 4-nitrophenol and 2-nitroaniline, respectively. Besides, it shows a superior activity for catalytic degradation of methyl orange. All the three catalytic reactions were carried out in aqueous medium at room temperature and in the presence of reducing agent NaBH4. The magnetic behavior of the synthesized Ag–γ-Fe2O3 enables the ease of separation of the nanocomposite from the reaction medium for further reuse.
Graphical Abstract













Similar content being viewed by others
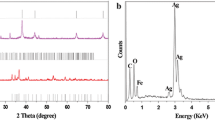
References
Barman BK, Nanda KK (2015) Rapid reduction of GO by hydrogen spill-over mechanism by in situ generated nanoparticles at room temperature and their catalytic performance towards 4-nitrophenol reduction and ethanol oxidation. Appl Catal A 491:45–51
Zhang J, Chen G, Chaker M, Rosei F, Ma D (2013) Gold nanoparticle decorated ceria nanotubes with significantly high catalytic activity for the reduction of nitrophenol and mechanism study. Appl Catal B 132:107–115
Evangelista V, Acosta B, Miridonov S, Smolentseva E, Fuentes S, Simakov A (2015) Highly active Au-CeO2@ZrO2 yolk–shell nanoreactors for the reduction of 4-nitrophenol to 4-aminophenol. Appl Catal B 166–167:518–528
Petrova B, Budinova T, Tsyntsarski B, Kochkodan V, Shkavro Z, Petrov N (2010) Removal of aromatic hydrocarbons from water by activated carbon from apricot stones. Chem Eng J 165(1):258–264
Shen X-E, Shan X-Q, Dong D-M, Hua X-Y, Owens G (2009) Kinetics and thermodynamics of sorption of nitroaromatic compounds to as-grown and oxidized multiwalled carbon nanotubes. J Colloid Interface Sci 330(1):1–8
Ren M, Song Y, Xiao S, Zeng P, Peng J (2011) Treatment of berberine hydrochloride wastewater by using pulse electro-coagulation process with Fe electrode. Chem Eng J 169(1):84–90
Zhao L, Zhao H (2018) Magnetic N-doped Co-carbon composites derived from metal organic frameworks as highly efficient catalysts for 4-nitrophenol reduction reaction. Dalton Trans 47:3321–3328
Chakrabarti S, Ganguli D, Chaudhuri S (2004) Optical properties of γ-Fe2O3 nanoparticles dispersed on sol–gel silica spheres. Physica E 24(3):333–342
Ichiyanagi Y, Kimishima Y (2002) Structural, magnetic and thermal characterizations of Fe2O3 nanoparticle systems. J Thermal Anal Calorim 69(3):919–923
Ye X, Lin D, Jiao Z, Zhang L (1998) The thermal stability of nanocrystalline maghemite. J Phys D 31(20):2739
Innocenzi P (2003) Infrared spectroscopy of sol–gel derived silica-based films: a spectra-microstructure overview. J Non-Cryst Solids 316(2):309–319
Theivasanthi T, Alagar M (2011) Electrolytic synthesis and characterizations of silver nanopowder. arXiv preprint arXiv:1111.0260.
Dong Z, Le X, Li X, Zhang W, Dong C, Ma J (2014) Silver nanoparticles immobilized on fibrous nano-silica as highly efficient and recyclable heterogeneous catalyst for reduction of 4-nitrophenol and 2-nitroaniline. Appl Catal B 158:129–135
Moon B-H, Park Y-B, Park K-H (2011) Fenton oxidation of orange II by pre-reduction using nanoscale zero-valent iron. Desalination 268(1–3):249–252
Park H, Choi W (2003) Visible light and Fe (III)-mediated degradation of acid orange 7 in the absence of H2O2. J Photochem Photobiol A 159(3):241–247
Devi LG, Kumar SG, Reddy KM, Munikrishnappa C (2009) Photo degradation of methyl orange an azo dye by advanced fenton process using zero valent metallic iron: Influence of various reaction parameters and its degradation mechanism. J Hazard Mater 164(2–3):459–467
Li X, Xue H, Pang H (2017) Facile synthesis and shape evolution of well-defined phosphotungstic acid potassium nanocrystals as a highly efficient visible-light-driven photocatalyst. Nanoscale 9(1):216–222
Taylor R, Coulombe S, Otanicar T, Phelan P, Gunawan A, Lv W et al (2013) Small particles, big impacts: a review of the diverse applications of nanofluids. J Appl Phys 113(1):011301
Ó Dálaigh C, Corr SA, Gun’ko Y, Connon SJ (2007) A magnetic-nanoparticle-supported 4-N, N-dialkylaminopyridine catalyst: excellent reactivity combined with facile catalyst recovery and recyclability. Angew Chem 119(23):4407–4410
Frey NA, Peng S, Cheng K, Sun S (2009) Magnetic nanoparticles: synthesis, functionalization, and applications in bioimaging and magnetic energy storage. Chem Soc Rev 38(9):2532–2542
Singamaneni S, Bliznyuk VN, Binek C, Tsymbal EY (2011) Magnetic nanoparticles: recent advances in synthesis, self-assembly and applications. J Mater Chem 21(42):16819–16845
Li D, Teoh WY, Selomulya C, Woodward RC, Amal R, Rosche B (2006) Flame-sprayed superparamagnetic bare and silica-coated maghemite nanoparticles: synthesis, characterization, and protein adsorption-desorption. Chem Mater 18(26):6403–6413
Pankhurst QA, Connolly J, Jones S, Dobson J (2003) Applications of magnetic nanoparticles in biomedicine. J Phys D 36(13):R167
Lévy M, Wilhelm C, Siaugue J-M, Horner O, Bacri J-C, Gazeau F (2008) Magnetically induced hyperthermia: size-dependent heating power of γ-Fe2O3 nanoparticles. J Phys 20(20):204133
Kolhatkar AG, Jamison AC, Litvinov D, Willson RC, Lee TR (2013) Tuning the magnetic properties of nanoparticles. Int J Mol Sci 14(8):15977–16009
Thomas R, Park I-K, Jeong YY (2013) Magnetic iron oxide nanoparticles for multimodal imaging and therapy of cancer. Int J Mol Sci 14(8):15910–15930
Berry CC, Curtis AS (2003) Functionalisation of magnetic nanoparticles for applications in biomedicine. J Phys D 36(13):R198
Huo L, Li W, Lu L, Cui H, Xi S, Wang J et al (2000) Preparation, structure, and properties of three-dimensional ordered α-Fe2O3 nanoparticulate film. Chem Mater 12(3):790–794
Suresh R, Prabu R, Vijayaraj A, Giribabu K, Stephen A, Narayanan V (2012) Facile synthesis of cobalt doped hematite nanospheres: magnetic and their electrochemical sensing properties. Mater Chem Phys 134(2):590–596
Biswal RC (2011) Pure and Pt-loaded gamma iron oxide as sensor for detection of sub ppm level of acetone. Sens Actuators B 157(1):183–188
Jing Z (2006) Fabrication and gas sensing properties of Ni-doped gamma-Fe2O3 by anhydrous solvent method. Mater Lett 60(28):3315–3318
Barroso M, Cowan AJ, Pendlebury SR, Grätzel M, Klug DR, Durrant JR (2011) The role of cobalt phosphate in enhancing the photocatalytic activity of α-Fe2O3 toward water oxidation. J Am Chem Soc 133(38):14868–14871
Darezereshki E (2011) One-step synthesis of hematite (α-Fe2O3) nano-particles by direct thermal-decomposition of maghemite. Mater Lett 65(4):642–645
Rocher V, Manerova J, Kinnear M, Evans DJ, Francesconi MG (2014) Direct amine-functionalisation of gamma-Fe2O3 nanoparticles. Dalton Trans 43(7):2948–2952
Xu YX, Li B, Zheng SS, Wu P, Zhang J, Xue H et al (2018) Ultrathin two-dimensional cobalt-organic framework nanosheets for high-performance electrocatalytic oxygen evolution. J Mater Chem A. https://doi.org/10.1039/C8TA03128B
Chi Y, Yuan Q, Li Y, Tu J, Zhao L, Li N et al (2012) Synthesis of Fe3O4@ SiO2–Ag magnetic nanocomposite based on small-sized and highly dispersed silver nanoparticles for catalytic reduction of 4-nitrophenol. J Colloid Interface Sci 383(1):96–102
Cheng K, He Y, Miao Y, Zou B, Wang Y, Wang T et al (2006) Quantum size effect on surface photovoltage spectra: alpha-Fe2O3 nanocrystals on the surface of monodispersed silica microsphere. J Phys Chem B 110(14):7259–7264
Banerjee A, Patra S, Chakrabarti M, Sanyal D, Pal M, Pradhan SK (2011) Microstructure, Mössbauer, and optical characterizations of nanocrystalline α-Fe2O3 synthesized by chemical route. ISRN Ceram. https://doi.org/10.5402/2011/406094
Li X, Wang X, Song S, Liu D, Zhang H (2012) Selectively deposited noble metal nanoparticles on Fe3O4/graphene composites: stable, recyclable, and magnetically separable catalysts. Chemistry 18(24):7601–7607
Gu H, Wang J, Ji Y, Wang Z, Chen W, Xue G (2013) Facile and controllable fabrication of gold nanoparticles-immobilized hollow silica particles and their high catalytic activity. J Mater Chem A 1(40):12471–12477
Pradhan N, Pal A, Pal T (2001) Catalytic reduction of aromatic nitro compounds by coinage metal nanoparticles. Langmuir 17(5):1800–1802
Gu Y, Jiao Y, Zhou X, Wu A, Buhe B, Fu H (2018) Strongly coupled Ag/TiO2 heterojunctions for effective and stable photothermal catalytic reduction of 4-nitrophenol. Nano Res 11(1):126–141
Baruah B, Gabriel GJ, Akbashev MJ, Booher ME (2013) Facile synthesis of silver nanoparticles stabilized by cationic polynorbornenes and their catalytic activity in 4-nitrophenol reduction. Langmuir 29(13):4225–4234
Fath RH, Hoseini SJ, Khozestan HG (2017) A nanohybrid of organoplatinum(II) complex and graphene oxide as catalyst for reduction of p-nitrophenol. J Organomet Chem 842:1–8
Arumugam V, Sriram P, Yen T-J, Redhi GG, Gengan RM (2018) Nano-material as an excellent catalyst for reducing a series of nitroanilines and dyes: triphosphonated ionic liquid-CuFe2O4-modified boron nitride. Appl Catal B 222:99–114
Sahiner N, Ozay H, Ozay O, Aktas N (2010) New catalytic route: hydrogels as templates and reactors for in situ Ni nanoparticle synthesis and usage in the reduction of 2-and 4-nitrophenols. Appl Catal A 385(1):201–207
Sahiner N, Ozay O (2012) Enhanced catalytic activity in the reduction of 4-nitrophenol and 2-nitrophenol by p (AMPS)-Cu (0) hydrogel composite materials. Curr Nanosci 8(3):367–374
Sahiner N, Ozay H, Ozay O, Aktas N (2010) A soft hydrogel reactor for cobalt nanoparticle preparation and use in the reduction of nitrophenols. Appl Catal B 101(1):137–143
Yang S, Nie C, Liu H, Liu H (2013) Facile synthesis and catalytic application of Ag–Fe2O3–carbons nanocomposites. Mater Lett 100:296–298
Tang S, Vongehr S, Meng X (2009) Carbon spheres with controllable silver nanoparticle doping. J Phys Chem C 114(2):977–982
Rashid MH, Mandal TK (2007) Synthesis and catalytic application of nanostructured silver dendrites. J Phys Chem C 111(45):16750–16760
Gao C, An Q, Xiao Z, Zhai S, Zhai B, Shi Z (2018) Alginate and polyethyleneimine dually mediated synthesis of nanosilver-containing composites for efficient p-nitrophenol reduction. Carbohydr Polym 181:744–751
Sau TK, Pal A, Pal T (2001) Size regime dependent catalysis by gold nanoparticles for the reduction of eosin. J Phys Chem B 105(38):9266–9272
Jiang Z, Jiang D, Hossain AS, Qian K, Xie J (2015) In situ synthesis of silver supported nanoporous iron oxide microbox hybrids from metal-organic frameworks and their catalytic application in p-nitrophenol reduction. Phys Chem Chem Phys 17(4):2550–2559
Feng J, Fan D, Wang Q, Ma L, Wei W, Xie J et al (2017) Facile synthesis silver nanoparticles on different xerogel supports as highly efficient catalysts for the reduction of p-nitrophenol. Colloids Surf A 520:743–756
Du X, He J, Zhu J, Sun L, An S (2012) Ag-deposited silica-coated Fe3O4 magnetic nanoparticles catalyzed reduction of p-nitrophenol. Appl Surf Sci 258(7):2717–2723
Alshehri SM, Almuqati T, Almuqati N, Al-Farraj E, Alhokbany N, Ahamad T (2016) Chitosan based polymer matrix with silver nanoparticles decorated multiwalled carbon nanotubes for catalytic reduction of 4-nitrophenol. Carbohydr Polym 151:135–143
Ismail M, Khan M, Khan SB, Khan MA, Akhtar K, Asiri AM (2018) Green synthesis of plant supported CuAg and CuNi bimetallic nanoparticles in the reduction of nitrophenols and organic dyes for water treatment. J Mol Liq 260:78–91
Arora N, Mehta A, Mishra A, Basu S (2018) 4-Nitrophenol reduction catalysed by Au-Ag bimetallic nanoparticles supported on LDH: homogeneous vs. heterogeneous catalysis. Appl Clay Sci 151:1–9
Gupta N, Singh HP, Sharma RK (2011) Metal nanoparticles with high catalytic activity in degradation of methyl orange: an electron relay effect. J Mol Catal A 335(1):248–252
Naraginti S, Stephen FB, Radhakrishnan A, Sivakumar A (2015) Zirconium and silver co-doped TiO2 nanoparticles as visible light catalyst for reduction of 4-nitrophenol, degradation of methyl orange and methylene blue. Spectrochim Acta A 135:814–819
Saleh TA, Gupta VK (2012) Photo-catalyzed degradation of hazardous dye methyl orange by use of a composite catalyst consisting of multi-walled carbon nanotubes and titanium dioxide. J Colloid Interface Sci 371(1):101–106
Cano OA, González CR, Paz JH, Madrid PA, Casillas PG, Hernández AM et al (2017) Catalytic activity of palladium nanocubes/multiwalled carbon nanotubes structures for methyl orange dye removal. Catal Today 282:168–173
Safavi A, Momeni S (2012) Highly efficient degradation of azo dyes by palladium/hydroxyapatite/Fe3O4 nanocatalyst. J Hazard Mater 201:125–131
Author information
Authors and Affiliations
Corresponding author
Ethics declarations
Conflict of interest
We declare that no conflict of interest exists.
Rights and permissions
About this article
Cite this article
Sallam, S.A., El-Subruiti, G.M. & Eltaweil, A.S. Facile Synthesis of Ag–γ-Fe2O3 Superior Nanocomposite for Catalytic Reduction of Nitroaromatic Compounds and Catalytic Degradation of Methyl Orange. Catal Lett 148, 3701–3714 (2018). https://doi.org/10.1007/s10562-018-2569-z
Received:
Accepted:
Published:
Issue Date:
DOI: https://doi.org/10.1007/s10562-018-2569-z