Abstract
The expression and activation of EphA4 in the various cell types in a knee joint was upregulated upon an intraarticular injury. To determine if EphA4 signaling plays a role in osteoarthritis, we determined whether deficient EphA4 expression (in EphA4 knockout mice) or upregulation of the EphA4 signaling (with the EfnA4-fc treatment) would alter cellular functions of synoviocytes and articular chondrocytes. In synoviocytes, deficient EphA4 expression enhanced, whereas activation of the EphA4 signaling reduced, expression and secretion of key inflammatory cytokines and matrix metalloproteases. Conversely, in articular chondrocytes, activation of the EphA4 signaling upregulated, while deficient EphA4 expression reduced, expression levels of chondrogenic genes (e.g., aggrecan, lubricin, type-2 collagen, and Sox9). EfnA4-fc treatment in wildtype, but not EphA4-deficient, articular chondrocytes promoted the formation and activity of acidic proteoglycan-producing colonies. Activation of the EphA4 signaling in articular chondrocytes upregulated Rac1/2 and downregulated RhoA via enhancing Vav1 and reducing Ephexin1 activation, respectively. However, activation of the EphA4 signaling in synoviocytes suppressed the Vav/Rac signaling while upregulated the Ephexin/Rho signaling. In summary, the EphA4 signaling in synoviocytes is largely of anti-catabolic nature through suppression of the expression of inflammatory cytokines and matrix proteases, but in articular chondrocytes the signaling is pro-anabolic in that it promotes the biosynthesis of articular cartilage. The contrasting action of the EphA4 signaling in synoviocytes as opposing to articular chondrocytes may in part be mediated through the opposite differential effects of the EphA4 signaling on the Vav/Rac signaling and Ephexin/Rho signaling in the two skeletal cell types.









Similar content being viewed by others
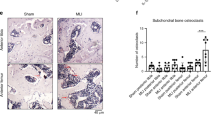
Data Availability
All research data of this study and materials used in this study are stored in the laboratory of Dr. Kin-Hing William Lau, and they may be available to others upon request in writing and approval from the Research Office of the Loma Linda VA Healthcare system.
References
Gale NW, Holland SJ, Valenzuela DM, Flenniken A, Pan L, Ryan TE, Henkemeyer M, Strebhardt K, Hirai H, Wilkinson DG, Pawson T, Davis S, Yancopoulos GD (1996) Eph receptors and ligands comprise two major specificity subclasses and are reciprocally compartmentalized during embryogenesis. Neuron 17:9–19
Pasquale EB (1997) The Eph family of receptors. Curr Opin Cell Biol 9:608–615
Poitz DM, Ende G, Stutz B, Augstein A, Friedrichs J, Brunssen C, Werner C, Strasser RH, Jellinghaus S (2015) EphrinB2/EphA4-mediated activation of endothelial cells increases monocyte adhesion. Mol Immunol 68:648–656
Zimmer G, Rudolph J, Landmann J, Gerstmann K, Steinecke A, Gampe C, Bolz J (2011) Bidirectional ephrinB3/EphA4 signaling mediates the segregation of medial ganglionic eminence- and preoptic area-derived interneurons in the deep and superficial migratory stream. J Neurosci 31:18364–18380
Himanen JP, Chumley MJ, Lackmann M, Li C, Barton WA, Jeffrey PD, Vearing C, Geleick D, Feldheim DA, Boyd AW, Henkemeyer M, Nikolov DB (2004) Repelling class discrimination: ephrin-A5 binds to and activates EphB2 receptor signaling. Nat Neurosci 7:501–509
Wybenga-Groot LE, Baskin B, Ong SH, Tong J, Pawson T, Sicheri F (2001) Structural basis for autoinhibition of the Ephb2 receptor tyrosine kinase by the unphosphorylated juxtamembrane region. Cell 106:745–757
Zisch AH, Pazzagli C, Freeman AL, Schneller M, Hadman M, Smith JW, Ruoslahti E, Pasquale EB (2000) Replacing two conserved tyrosines of the EphB2 receptor with glutamic acid prevents binding of SH2 domains without abrogating kinase activity and biological responses. Oncogene 19:177–187
Kullander K, Klein R (2002) Mechanisms and functions of Eph and ephrin signalling. Nat Rev Mol Cell Biol 3:475–486
Pasquale EB (2008) Eph-ephrin bidirectional signaling in physiology and disease. Cell 133:38–52
Kuijper S, Turner CJ, Adams RH (2007) Regulation of angiogenesis by Eph-ephrin interactions. Trends Cardiovasc Med 17:145–151
Genander M, Frisen J (2010) Ephrins and Eph receptors in stem cells and cancer. Curr Opin Cell Biol 22:611–616
Pasquale EB (2005) Eph receptor signalling casts a wide net on cell behaviour. Nat Rev Mol Cell Biol 6:462–475
Rundle CH, Xing W, Lau KW, Mohan S (2016) Bidirectional ephrin signaling in bone. Osteoporos Sarcopenia 2:65–76
Edwards CM, Mundy GR (2008) Eph receptors and ephrin signaling pathways: a role in bone homeostasis. Int J Med Sci 5:263–272
Compagni A, Logan M, Klein R, Adams RH (2003) Control of skeletal patterning by ephrinB1-EphB interactions. Dev Cell 5:217–230
Wieland I, Jakubiczka S, Muschke P, Cohen M, Thiele H, Gerlach KL, Adams RH, Wieacker P (2004) Mutations of the ephrin-B1 gene cause craniofrontonasal syndrome. Am J Hum Genet 74:1209–1215
Allan EH, Hausler KD, Wei T, Gooi JH, Quinn JM, Crimeen-Irwin B, Pompolo S, Sims NA, Gillespie MT, Onyia JE, Martin TJ (2008) EphrinB2 regulation by PTH and PTHrP revealed by molecular profiling in differentiating osteoblasts. J Bone Miner Res 23:1170–1181
Kaur A, Xing W, Mohan S, Rundle CH (2019) Changes in ephrin gene expression during bone healing identify a restricted repertoire of ephrins mediating fracture repair. Histochem Cell Biol 151:43–55
Zhao C, Irie N, Takada Y, Shimoda K, Miyamoto T, Nishiwaki T, Suda T, Matsuo K (2006) Bidirectional ephrinB2-EphB4 signaling controls bone homeostasis. Cell Metab 4:111–121
Cheng S, Zhao SL, Nelson B, Kesavan C, Qin X, Wergedal J, Mohan S, Xing W (2012) Targeted disruption of ephrin B1 in cells of myeloid lineage increases osteoclast differentiation and bone resorption in mice. PLoS ONE 7:e32887
Irie N, Takada Y, Watanabe Y, Matsuzaki Y, Naruse C, Asano M, Iwakura Y, Suda T, Matsuo K (2009) Bidirectional signaling through ephrinA2-EphA2 enhances osteoclastogenesis and suppresses osteoblastogenesis. J Biol Chem 284:14637–14644
Stiffel V, Amoui M, Sheng MH, Mohan S, Lau KH (2014) EphA4 receptor is a novel negative regulator of osteoclast activity. J Bone Miner Res 29:804–819
Kwan Tat S, Pelletier JP, Amiable N, Boileau C, Lajeunesse D, Duval N, Martel-Pelletier J (2008) Activation of the receptor EphB4 by its specific ligand ephrin B2 in human osteoarthritic subchondral bone osteoblasts. Arthritis Rheumatol 58:3820–3830
Kwan Tat S, Pelletier JP, Amiable N, Boileau C, Lavigne M, Martel-Pelletier J (2009) Treatment with ephrin B2 positively impacts the abnormal metabolism of human osteoarthritic chondrocytes. Arthritis Res Ther 11:R119
Tonna S, Poulton IJ, Taykar F, Ho PW, Tonkin B, Crimeen-Irwin B, Tatarczuch L, McGregor NE, Mackie EJ, Martin TJ, Sims NA (2016) Chondrocytic ephrin B2 promotes cartilage destruction by osteoclasts in endochondral ossification. Development 143:648–657
Kwan Tat S, Lajeunesse D, Pelletier JP, Martel-Pelletier J (2010) Targeting subchondral bone for treating osteoarthritis: what is the evidence? Best Pract Res Clin Rheumatol 24:51–70
Valverde-Franco G, Pelletier JP, Fahmi H, Hum D, Matsuo K, Lussier B, Kapoor M, Martel-Pelletier J (2012) In vivo bone-specific EphB4 overexpression in mice protects both subchondral bone and cartilage during osteoarthritis. Arthritis Rheumatol 64:3614–3625
Valverde-Franco G, Lussier B, Hum D, Wu J, Hamadjida A, Dancause N, Fahmi H, Kapoor M, Pelletier JP, Martel-Pelletier J (2016) Cartilage-specific deletion of ephrin-B2 in mice results in early developmental defects and an osteoarthritis-like phenotype during aging in vivo. Arthritis Res Ther 18:65
Kuroda C, Kubota S, Kawata K, Aoyama E, Sumiyoshi K, Oka M, Inoue M, Minagi S, Takigawa M (2008) Distribution, gene expression, and functional role of EphA4 during ossification. Biochem Biophys Res Commun 374:22–27
Lau KH, Amoui M, Stiffel V, Chen ST, Sheng MH (2015) An osteoclastic transmembrane protein-tyrosine phosphatase enhances osteoclast activity in part by dephosphorylating EphA4 in osteoclasts. J Cell Biochem 116:1785–1796
Stiffel V, Rundle CH, Sheng MH-C, Das S, Lau K-HW (2019) A mouse noninvasive intraarticular tibial plateau compression loading-induced injury model of posttraumatic osteoarthritis. Calcif Tissue Int 106:158–171
Zhao J, Ouyang Q, Hu Z, Huang Q, Wu J, Wang R, Yang M (2016) A protocol for the culture and isolation of murine synovial fibroblasts. Biomed Rep 5:171–175
Senolt L, Grigorian M, Lukanidin E, Simmen B, Michel BA, Pavelka K, Gay RE, Gay S, Neidhart M (2006) S100A4 is expressed at site of invasion in rheumatoid arthritis synovium and modulates production of matrix metalloproteinases. Ann Rheum Dis 65:1645–1648
Vickers SM, Johnson LL, Zou LQ, Yannas IV, Gibson LJ, Spector M (2004) Expression of alpha-smooth muscle actin by and contraction of cells derived from synovium. Tissue Eng 10:1214–1223
Steenvoorden MM, Tolboom TC, van der Pluijm G, Lowik C, Visser CP, DeGroot J, Gittenberger-DeGroot AC, DeRuiter MC, Wisse BJ, Huizinga TW, Toes RE (2006) Transition of healthy to diseased synovial tissue in rheumatoid arthritis is associated with gain of mesenchymal/fibrotic characteristics. Arthritis Res Ther 8:R165
Jonason JH, Hoak D, O’Keefe RJ (2015) Primary murine growth plate and articular chondrocyte isolation and cell culture. Methods Mol Biol 1226:11–18
Haudenschild DR, Chen J, Pang N, Lotz MK, D’Lima DD (2010) Rho kinase-dependent activation of SOX9 in chondrocytes. Arthritis Rheumatol 62:191–200
Kinne RW, Stuhlmuller B, Burmester GR (2007) Cells of the synovium in rheumatoid arthritis. Macrophages. Arthritis Res Ther 9:224
Edwards JC (1982) The origin of type A synovial lining cells. Immunobiology 161:227–231
Kim K, Lee SA, Park D (2019) Emerging roles of ephexins in physiology and disease. Cells 8(2):87
Winning RS, Ward EK, Scales JB, Walker GK (2002) EphA4 catalytic activity causes inhibition of RhoA GTPase in Xenopus laevis embryos. Differentiation 70:46–55
Ogita H, Kunimoto S, Kamioka Y, Sawa H, Masuda M, Mochizuki N (2003) EphA4-mediated Rho activation via Vsm-RhoGEF expressed specifically in vascular smooth muscle cells. Circ Res 93:23–31
Richter M, Murai KK, Bourgin C, Pak DT, Pasquale EB (2007) The EphA4 receptor regulates neuronal morphology through SPAR-mediated inactivation of Rap GTPases. J Neurosci 27:14205–14215
Vedham V, Phee H, Coggeshall KM (2005) Vav activation and function as a rac guanine nucleotide exchange factor in macrophage colony-stimulating factor-induced macrophage chemotaxis. Mol Cell Biol 25:4211–4220
Sahin M, Greer PL, Lin MZ, Poucher H, Eberhart J, Schmidt S, Wright TM, Shamah SM, O’Connell S, Cowan CW, Hu L, Goldberg JL, Debant A, Corfas G, Krull CE, Greenberg ME (2005) Eph-dependent tyrosine phosphorylation of ephexin1 modulates growth cone collapse. Neuron 46:191–204
Lopez-Lago M, Lee H, Cruz C, Movilla N, Bustelo XR (2000) Tyrosine phosphorylation mediates both activation and downmodulation of the biological activity of Vav. Mol Cell Biol 20:1678–1691
Martin JA, Buckwalter JA (2006) Post-traumatic osteoarthritis: the role of stress induced chondrocyte damage. Biorheology 43:517–521
Strobel S, Loparic M, Wendt D, Schenk AD, Candrian C, Lindberg RL, Moldovan F, Barbero A, Martin I (2010) Anabolic and catabolic responses of human articular chondrocytes to varying oxygen percentages. Arthritis Res Ther 12:R34
Lotz MK, Kraus VB (2010) New developments in osteoarthritis. Posttraumatic osteoarthritis: pathogenesis and pharmacological treatment options. Arthritis Res Ther 12:211
Noren NK, Pasquale EB (2004) Eph receptor-ephrin bidirectional signals that target Ras and Rho proteins. Cell Signal 16:655–666
Shamah SM, Lin MZ, Goldberg JL, Estrach S, Sahin M, Hu L, Bazalakova M, Neve RL, Corfas G, Debant A, Greenberg ME (2001) EphA receptors regulate growth cone dynamics through the novel guanine nucleotide exchange factor ephexin. Cell 105:233–244
Chen F, Liu Z, Peng W, Gao Z, Ouyang H, Yan T, Ding S, Cai Z, Zhao B, Mao L, Cao Z (2018) Activation of EphA4 induced by EphrinA1 exacerbates disruption of the blood–brain barrier following cerebral ischemia-reperfusion via the Rho/ROCK signaling pathway. Exp Ther Med 16:2651–2658
Fang WB, Brantley-Sieders DM, Hwang Y, Ham AJ, Chen J (2008) Identification and functional analysis of phosphorylated tyrosine residues within EphA2 receptor tyrosine kinase. J Biol Chem 283:16017–16026
Bustelo XR (2002) Regulation of Vav proteins by intramolecular events. Front Biosci 7:d24–30
Wegmeyer H, Egea J, Rabe N, Gezelius H, Filosa A, Enjin A, Varoqueaux F, Deininger K, Schnutgen F, Brose N, Klein R, Kullander K, Betz A (2007) EphA4-dependent axon guidance is mediated by the RacGAP alpha2-chimaerin. Neuron 55:756–767
Takeuchi S, Yamaki N, Iwasato T, Negishi M, Katoh H (2009) Beta2-chimaerin binds to EphA receptors and regulates cell migration. FEBS Lett 583:1237–1242
DeGeer J, Kaplan A, Mattar P, Morabito M, Stochaj U, Kennedy TE, Debant A, Cayouette M, Fournier AE, Lamarche-Vane N (2015) Hsc70 chaperone activity underlies Trio GEF function in axon growth and guidance induced by netrin-1. J Cell Biol 210:817–832
Razidlo GL, Wang Y, Chen J, Krueger EW, Billadeau DD, McNiven MA (2013) Dynamin 2 potentiates invasive migration of pancreatic tumor cells through stabilization of the Rac1 GEF Vav1. Dev Cell 24:573–585
Gulbranson DR, Davis EM, Demmitt BA, Ouyang Y, Ye Y, Yu H, Shen J (2017) RABIF/MSS4 is a Rab-stabilizing holdase chaperone required for GLUT4 exocytosis. Proc Natl Acad Sci USA 114:E8224–E8233
Nethe M, Hordijk PL (2010) The role of ubiquitylation and degradation in RhoGTPase signalling. J Cell Sci 123:4011–4018
Kamynina E, Kauppinen K, Duan F, Muakkassa N, Manor D (2007) Regulation of proto-oncogenic dbl by chaperone-controlled, ubiquitin-mediated degradation. Mol Cell Biol 27:1809–1822
Oehler R, Pusch E, Zellner M, Dungel P, Hergovics N, Homoncik M, Eliasen MM, Brabec M, Roth E (2001) Cell type-specific variations in the induction of hsp70 in human leukocytes by feverlike whole body hyperthermia. Cell Stress Chaperones 6:306–315
Schotte P, Denecker G, Van Den Broeke A, Vandenabeele P, Cornelis GR, Beyaert R (2004) Targeting Rac1 by the Yersinia effector protein YopE inhibits caspase-1-mediated maturation and release of interleukin-1beta. J Biol Chem 279:25134–25142
Zhuge Y, Xu J (2001) Rac1 mediates type I collagen-dependent MMP-2 activation. Role in cell invasion across collagen barrier. J Biol Chem 276:16248–16256
Tkach V, Bock E, Berezin V (2005) The role of RhoA in the regulation of cell morphology and motility. Cell Motil Cytoskelet 61:21–33
Shen DW, Pouliot LM, Gillet JP, Ma W, Johnson AC, Hall MD, Gottesman MM (2012) The transcription factor GCF2 is an upstream repressor of the small GTPAse RhoA, regulating membrane protein trafficking, sensitivity to doxorubicin, and resistance to cisplatin. Mol Pharm 9:1822–1833
Chi X, Wang S, Huang Y, Stamnes M, Chen JL (2013) Roles of rho GTPases in intracellular transport and cellular transformation. Int J Mol Sci 14:7089–7108
Deng Z, Jia Y, Liu H, He M, Yang Y, Xiao W, Li Y (2019) RhoA/ROCK pathway: implication in osteoarthritis and therapeutic targets. Am J Transl Res 11:5324–5331
Zhu S, Liu H, Wu Y, Heng BC, Chen P, Liu H, Ouyang HW (2013) Wnt and Rho GTPase signaling in osteoarthritis development and intervention: implications for diagnosis and therapy. Arthritis Res Ther 15:217
Pritchard S, Votta BJ, Kumar S, Guilak F (2008) Interleukin-1 inhibits osmotically induced calcium signaling and volume regulation in articular chondrocytes. Osteoarthr Cartil 16:1466–1473
Appleton CT, Usmani SE, Mort JS, Beier F (2010) Rho/ROCK and MEK/ERK activation by transforming growth factor-alpha induces articular cartilage degradation. Lab Investig 90:20–30
Liang J, Feng J, Wu WK, Xiao J, Wu Z, Han D, Zhu Y, Qiu G (2011) Leptin-mediated cytoskeletal remodeling in chondrocytes occurs via the RhoA/ROCK pathway. J Orthop Res 29:369–374
Woods A, Wang G, Beier F (2005) RhoA/ROCK signaling regulates Sox9 expression and actin organization during chondrogenesis. J Biol Chem 280:11626–11634
Wang G, Woods A, Agoston H, Ulici V, Glogauer M, Beier F (2007) Genetic ablation of Rac1 in cartilage results in chondrodysplasia. Dev Biol 306:612–623
Woods A, Pala D, Kennedy L, McLean S, Rockel JS, Wang G, Leask A, Beier F (2009) Rac1 signaling regulates CTGF/CCN2 gene expression via TGFbeta/Smad signaling in chondrocytes. Osteoarthr Cartil 17:406–413
Nishida T, Kubota S, Kojima S, Kuboki T, Nakao K, Kushibiki T, Tabata Y, Takigawa M (2004) Regeneration of defects in articular cartilage in rat knee joints by CCN2 (connective tissue growth factor). J Bone Miner Res 19:1308–1319
Wang G, Yan Q, Woods A, Aubrey LA, Feng Q, Beier F (2011) Inducible nitric oxide synthase-nitric oxide signaling mediates the mitogenic activity of Rac1 during endochondral bone growth. J Cell Sci 124:3405–3413
Acknowledgements
The work is supported in part by a Grant from the Department of Defense, the US Army Medical Research and Materiel Command (USAMRMC) under Grant No. W81XWH-14-1-0207 and a Merit Review Grant (IO1 BX002964) from the Biomedical Laboratory Research and Development Service, Department of Veterans Affairs, Veterans Health Administration, Office of Research and Development. The views, opinions and/or findings contained in this report are those of the authors and should not be construed as an official position, policy or decision of the US Army, the Department of Veterans Affairs, or the US Government, unless so designated by other documentation. The funders had no role in study design, data collection and analysis, decision to publish, or preparation of the manuscript. In conducting research using animals, the investigators adhered to the Animal Welfare Act Regulations and other Federal statutes relating to animals and experiments involving animals and the principles set forth in the current version of the National Institutes of Health (NIH) Guidelines for Research Involving Recombinant DNA Molecules. Dr. Lau is also the recipient of a Research Career Scientist Award (1K6 BX002782) from the Department of Veterans Affairs, Veterans Health Administration, Office of Research and Development.
Author information
Authors and Affiliations
Contributions
K-HWL, VS, and MS contributed to the conception and design of the study. Material preparation and data collection were performed by VS, AT, and CR. Data analyses were performed by K-HWL and VS. The first draft of the manuscript was written by K-HWL. All authors commented on previous versions of the manuscript, read and approved the final manuscript.
Corresponding author
Ethics declarations
Conflict of interest
All authors (Virginia Stiffel, Alexander Thomas, Charles H. Rundle, Matilda H.-C. Sheng, and Kin-Hing William Lau) have no conflict of interest to declare.
Human and Animal Rights
All animal protocols were reviewed and approved by the Animal Care and Use Committee of the Pettis Memorial VA Medical Center and the Animal Care and Use Review Office (ACURO) of US Army Medical Research and Materiel Command of the Department of Defense. In conducting research using animals, the investigators adhered to the Animal Welfare Act Regulations and other Federal statutes relating to animals and experiments involving animals.
Additional information
Publisher's Note
Springer Nature remains neutral with regard to jurisdictional claims in published maps and institutional affiliations.
Rights and permissions
About this article
Cite this article
Stiffel, V.M., Thomas, A., Rundle, C.H. et al. The EphA4 Signaling is Anti-catabolic in Synoviocytes but Pro-anabolic in Articular Chondrocytes. Calcif Tissue Int 107, 576–592 (2020). https://doi.org/10.1007/s00223-020-00747-7
Received:
Accepted:
Published:
Issue Date:
DOI: https://doi.org/10.1007/s00223-020-00747-7