Abstract
Sterols are known for a plethora of 250 different structures. Between 5 and 10% of them usually occur with varying abundance ratios (~ four orders of magnitude) and total amounts (0.4–1000 mg/100 g oil) in samples. Yet, quantitative data are mostly restricted to the few major sterols which are available as reference standards. Here, we developed a gas chromatography with mass spectrometry method operated in selected ion monitoring mode (GC/MS-SIM) that enabled the quantitation of 30 (silylated) sterols although only ten were available as reference standards. This could be obtained by studying the full-scan mass spectra of these ten sterol standards and 20 additional sterols measured in seven oils. In the next step, sterols were assigned to different groups. Values for quantification were then selected on the premise that response factors were constant within a sterol group. The deviation of the response factors within one sterol group was frequently below ± 10% and otherwise about ± 11–12%. Using mean response factors for all sterols, the novel GC/MS-SIM quantification method was superior to GC/FID which was exemplarily applied to two oils. Between eight and 21 of the 30 studied sterols and pentacyclic triterpenols were detected and quantified in 18 vegetable oils and two vegetable fats. The much higher number of sterols that could be quantified resulted in higher sterol amounts and the method and data may be useful for food authentication.
Similar content being viewed by others
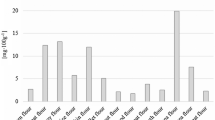
Avoid common mistakes on your manuscript.
Introduction
Sterols are (predominantly) tetracyclic lipophilic compounds that are omnipresent in food, but their contributions to the lipids of samples are low (typically ~ 1% [1]). More than 250 structural variants are known, and many of them are isomers, because, with very few exceptions, sterols consist of 27–31 carbon atoms along with 0–3 double bonds. Typically, between five and 15 sterols are analyzed in edible oils [2,3,4,5]. However, the amounts of individual sterols in samples can vary by four orders of magnitude (~ 0.4–1000 mg/100 g), but usually only the most abundant representatives are reported in scientific publications [6, 7]. Most data exist for members of the subgroup of ∆5-desmethylsterols (which feature a double bond on C-5 and no methyl group on C-4) like β-sitosterol, stigmasterol, and campesterol in plants and cholesterol in animals.
Vice versa, comparatively little knowledge exists on the quantities of members of the subgroups of (i) ∆7-desmethylsterols (double bond on C-7, no methyl group on C-4), (ii) 4,4-dimethylsterols (two methyl groups on C-4), and (iii) 4-methylsterols (one methyl group on C-4). The same is also a fact for pentacyclic triterpenols (amyrins and lupeol), which, in a narrower sense, do not belong to the true sterols but whose biosynthesis starts with the same precursor (i.e., squalene) [8]. However, the additional determination of the members of these subgroups would be beneficial in different aspects. For example, such data can provide valuable information about distinct biosynthesis pathways and for the authentication of food [9, 10]. In certain edible oils, such as amaranth or quinoa, members of these subgroups can also occur in high abundances [7, 11, 12].
Most frequently, sterols are enriched with the so-called unsaponifiable matter, and, after silylation, they are determined by gas chromatography with flame ionization detection (GC/FID) [2, 3, 5]. GC/FID is an easy-to-handle method, but its application requires the availability of reference standards and/or reliable retention times (relative to internal standard betulin), which are only available for 20 sterols with only three representatives of ∆7-desmethylsterols and no representatives of 4,4-dimethylsterols and 4-methylsterols [2, 3, 5, 13, 14]. While some of the problems in the GC/FID determination of sterols can be overcome by GC with mass spectrometry (GC/MS), its application is still a challenge due to the numerous co-elutions [6]. Especially in the last five years, attempts were made to quantify sterols by GC/MS operated in selected ion monitoring (SIM) mode [4, 15, 16] or by high-performance liquid chromatography (HPLC) [17, 18]. Still, data were usually restricted to sterols available as reference standards.
In this study, we aimed to develop a method which enables the quantification of minor sterols without the necessity to have them available as individual reference standards. In the first step, GC/MS full-scan spectra were recorded of ten sterol standards and ~ 20 sterols in seven plant oils followed by the selection of suitable quantification ions. Then, the sterols were assigned to individual subgroups in dependence on the structure of the ring system (double bond, methyl group(s), and cyclopropane ring). Finally, data of at least three representatives within the same subgroup were selected, which summed up to 30 sterols and pentacyclic triterpenols. The performance of the new method was compared with GC/FID determinations, and finally, the GC/MS-SIM method was applied to those of the 30 sterols that could be detected in 18 vegetable oils and two vegetable fats.
Materials and methods
Chemicals and standards
Stigmasterol (~ 95%) and cholestanol (≥ 99%) were from Fluka (Taufkirchen, Germany), whereas lupeol (≥ 98%), α-amyrin (≥ 98%), and campesterol (≥ 90%) were obtained from Santa Cruz Biotechnology (Heidelberg, Germany). Spinasterol was from Cayman Chemical (Tallinn, Estonia). A semipure β-sitosterol standard (total sterols, 95%; unsaturated sterols calculated as β-sitosterol, 85%) was ordered from Merck (Darmstadt, Germany). Lanosterol (95%) was isolated by Rüttler et al. [19]. Cholesterol (≥ 99%, sigma grade), cycloartenol (≥ 90%), and trifluorotoluene (benzotrifluoride, BTF) were from Sigma-Aldrich (Steinheim, Germany). The silylating agent consisting of N,O-bis(trimethylsilyl)-trifluoroacetamide (BSTFA) and trimethylchlorosilane (TMCS), 99:1, v/v (SILYL-991) was from Macherey-Nagel (Düren, Germany). Dichloromethane (≥ 99.8%), acetonitrile, and n-hexane (both HPLC grade) were from Th. Geyer (Renningen, Germany). Potassium hydroxide (> 85%) was from Carl Roth (Karlsruhe, Germany), and ethanol (technical grade, distilled prior to use) was from BASF (Ludwigshafen, Germany). Helium (99.9990% purity) and nitrogen (99.95% purity) were from Westfalen Company (Münster, Germany). 5α-Cholestane (98%) was from Acros Organics (Geel, Belgium). The internal standards (IS)—fatty acid pyrrolidines (FAP) and cholestanyl-tert-butyldimethylsilylether (cholestanyl-TBDMS)—were prepared as previously described [7, 20].
Samples
Eighteen vegetable oils and two vegetable fats (see below) were bought in local supermarkets and stored at 4 °C until sample preparation.
Sample preparation
Sample saponification, liquid–liquid extraction, and trimethylsilylation of aliquots were performed as previously described [20]. Silylated sample solutions were evaporated to dryness and then taken up in 100 µL TBDMS-IS solution (15 µg/mL cholestanyl-TBDMS), which also contained 5α-cholestane (6 µg/mL).
Gas chromatography with mass spectrometry (GC/MS)
Trimethylsilylated sterols were measured on a 6890 GC/5973N instrument (Hewlett-Packard/Agilent, Waldbronn, Germany) with the parameters of system 1 (30 m × 0.25 mm i.d. × 0.25 µm film thickness Optima 5HT column (Macherey-Nagel, Düren, Germany)) of Schlag et al. [20]. GC/MS full-scan spectra (m/z 50–650) were recorded to derive the following 17 m/z values measured in the selected ion monitoring (SIM) mode in five time windows with time window 1 (17–30.56 min): m/z 113.0, 129.1, 211.2, 213.2, 215.2, 217.2, 253.2, 255.2, 296.2, 456.4, 458.4, 460.4, 468.4, 470.4, 472.4, 474.4, and 482.4; time window 2 (30.56–32.5 min): m/z 113.0, 126.0, 129.1, 211.2, 213.2, 215.2, 253.2, 255.2, 296.2, 470.4, 472.4, 474.4, 482.4, 484.4, 486.4, 498.4, and 500.4; time window 3 (32.5–33.8 min): m/z 113.0, 129.1, 189.2, 211.2, 213.2, 215.2, 218.2, 253.2, 255.2, 296.2, 482.4, 484.4, 486.4, 488.4, 498.4, 500.4, and 512.5; time window 4 (33.8–34.5 min): m/z 113.0, 129.1, 189.2, 211.2, 213.2, 215.2, 218.2, 253.2, 255.2, 296.2, 365.3, 393.4, 408.4, 484.4, 486.4, 498.4, and 512.5; and time window 5 (34.5–41.8 min): m/z 113.0, 129.1, 211.2, 213.2, 215.2, 253.2, 255.2, 296.2, 445.4, 496.4, 498.4, 500.4, 502.5, 510.5, 512.5, 514.5, and 516.5. Retention indices (RIFAP) were calculated based on a homologous series of fatty acid pyrrolidides (FAPs), and retention time locking (RTL) was implemented as previously described [20]. The method precision, which was defined as the (mean) relative standard deviation of the sample (RSDsample), was determined as already described [7, 21].
Complementary GC/MS measurements were performed on a second 6890 GC/5973N instrument (Hewlett-Packard/Agilent, Waldbronn, Germany) equipped with an HP-5MS column (30 m, 0.25 mm i.d., 0.25 µm film thickness, Hewlett-Packard/Agilent, Waldbronn, Germany). Parameters were the same as those mentioned above (system 1) with the exception of the lengths of the time windows (here: time window 1: 16–23.4 min; time window 2: 23.4–25.5 min, time window 3: 25.5–26.85 min, time window 4: 26.85–27.6 min, and time window 5: 27.6–41.8 min).
Gas chromatography with flame ionization detection (GC/FID)
Analyses were performed on a 5890 Series II gas chromatograph with flame ionization detector (FID) system (Hewlett-Packard/Agilent, Waldbronn, Germany) operated in split mode (1.5 μL injected, split ratio: 7.9:1). The GC oven, equipped with the same HP-5MS column type as in GC/MS system II (30 m, 0.25 mm i.d. 0.25 µm film thickness, Hewlett-Packard/Agilent, Waldbronn, Germany), was heated as follows: After 1 min at 60 °C, T was raised at 20 °C/min to 283 °C and then at 1.5 °C/min to 300 °C (hold time: 20 min). The injector and detector were maintained at 250 °C and 310 °C, whereas the flow rate of the carrier gas nitrogen was set at 0.9 mL/min.
Isolation of β-sitosterol by countercurrent chromatography (CCC)
The semipure β-sitosterol standard (β-sitosterol content ~ 55%) was purified by CCC according to Schröder et al. [7, 22] except that a Quickprep MK8 (AECS London, UK) instrument (operated in tail-to-head mode) was used with the parameters of Schlag et al. [7, 22]. The sample (410 mg) was diluted in 9 mL of the biphasic solvent system (n-hexane:acetonitrile:BTF, 20:13:7, v/v/v) and injected via a 10 mL sample loop. Separations were performed in coil 2 and coil 3 (total volume 236 mL), and the retention of the stationary phase (Sf value) was 81% which corresponded with the presence of 191 mL lower phase and 45 mL upper mobile phase). At a flow rate of 2 mL/min, twenty-five 2 mL fractions were collected between 55 min (110 mL) and 80 min (160 mL). After solvent removal and weighing, the residue was rediluted in 1 mL solvent (n-hexane/dichloromethane, 9:1, v/v), and aliquots (~ 80 µg) were silylated and analyzed by GC/MS. Fractions with > 70% β-sitosterol were pooled and CCC-fractionated for a second time (Sf = 77%; 182/54 mL lower/upper phase). Ten of the twenty 2 mL fractions taken between 47.5 min (95 mL) and 67.5 min (135 mL) contained pure β-sitosterol (~ 99%), and the resulting standard was used for quantitative sterol analysis (Table S1).
Results and discussion
GC/MS-SIM values, response factors, and quality control criteria
After silylation, GC/MS full-scan spectra were recorded for ten reference standards and 20 additional sterols that were present with sufficient purity and abundance in seven edible oils. Then, the percentage contributions of the respective molecular ion M+ (Fig. 1a) and the following substance-specific SIM values were determined for the 30 sterols (Table S1), i.e., (i) the general “sterol ion” m/z 129 (Fig. 1b), (ii) m/z 255 [M-90-SC]+ (Fig. 1d), and (iii) m/z 213 ([M-90-SC-42]+) (Fig. 1c) for 4-desmethylsterols (with 90 u being “TMSOH”, 42 u being “C3H6” (C-15 to C-17), and SC being the mass of the side chain attached to C-17, which consists of at least eight carbons) (Table S2), as well as m/z 189 (which was superior to m/z 218) for the determination of pentacyclic triterpenols (Fig. S1) [20].
Initially, it was intended to determine (4-desmethyl-) stanols via m/z 215 [M-90-SC-42]+. Unfortunately, this SIM value turned out to be unsuited (see below). In addition, m/z 215 was also prominent in the GC/MS spectra of 4,4-dimethyl- and 4-methylsterols (the second most abundant next to m/z 129). However, in these cases, m/z 215 cannot be formed in the same way due to the presence of additional methyl groups (e.g., methyl groups in 4,4,14-positions would lead to the [M-90-SC-42]+ fragment ion at m/z 257 for 4,4-dimethylsterols). Still, the lack of Si isotope peaks indicated that m/z 215 only consisted of carbon and hydrogen atoms, i.e., [C16H23]+ as in the case of 4-desmethylstanols (Fig. S2). Here, we suggest that the known fragment ion at m/z 297 of 4,4-dimethylsterols ([M-90-SC]+) [23] is further fragmented by a retro-Diels–Alder reaction in ring A to give m/z 215 (Fig. 2). A similar fragmentation pathway had also been described for 4-desmethylsterols to give m/z 201 for stanols and m/z 199 for ∆5- and ∆7-sterols from m/z 257 and m/z 255 [24]. While the formation of m/z 215 in the GC/MS spectra of silylated 4-methylsterols could not be unraveled in this study, 4-desmethylstanols could be distinguished from the other two subgroups due to the formation of different base peaks (i.e., m/z 215 for 4-desmethylstanols and m/z 129 for 4,4-dimethylsterols and 4-methylsterols) as well as their different molecular ions. Accordingly, the ratio m/z 129/m/z 215 (R129/215) could be used for the assignment with R129/215 being < 1 pointing to 4-desmethylstanols and > 1 for 4,4-dimethylsterols and 4-methylsterols.
Then, the 30 sterols were assigned to four subgroups in dependence of (i) the number of double bonds, (ii) the double bond position in ring B, (iii) the presence of one or (two) methyl groups at C-4, and (iv) the presence of a methylene bridge (cyclopropane ring) (Tables 1, S2). Evaluation of all data showed that the % contribution to the TIC of the corresponding GC/MS-SIM values of the selected quantification ion was frequently < ± 10% (Fig. 3) and scarcely (amyrins, ∆7,SC-sterols, and 4,4-dimethylsterols with a cyclopropane ring) at about ± 11–12% RSD within a subgroup. For instance, m/z 129 contributed with 4.72 ± 0.38% (RSD ± 8.0%) to the TIC of diunsaturated ∆5-desmethylsterols with a second double bond in the side chain (∆5,SC-desmethylsterol, #10–14, n = 5) (Table 1). Likewise, M+ of ∆7- and ∆8-desmethylsterols (#7–9, n = 3) represented 5.64 ± 0.54% (RSD ± 9.6%) of the TIC (Table 1), so that both subgroups could be determined with the mean value as the response factor (Fig. 3a). However, the high RSD of ~ ± 37% observed with m/z 129, when 4,4-dimethylsterols with and without a cyclopropane ring were included, made it necessary to assign them to two different subgroups with different response factors, resulting in better RSDs of ± 1% and ± 13%. (Table 1, Fig. 3b). Besides, the contributions of m/z 129 to the TIC showed a rather high variation for 4-methylsterols with ~ ± 17% RSD. However, since the remaining ions could not always be detected due to the low concentration and the low response (Table S2), this ion was used nevertheless for quantification. As 4-methylsterols and 4,4-dimethylsterols with a cyclopropane ring had nearly the same correction factors, this problem made it impossible to determine unknown 4-methyl- and 4,4-dimethylsterols correctly with this method. However, 4-methyl- and 4,4-dimethylsterols can be separated into two fractions by SPE if this information is required [9]. Given the extraordinary role of the methyl groups on C-4, the thirty sterols determined in this study were numbered (from #1 to #30) and the numbers were additionally with a subscript number (“0” for 4-desmethylsterols, “1” for 4-methylsterols and “2” for 4,4-dimethylsterols) or letter (“t” for triterpenols). Accordingly, cholesterol will be presented as cholesterol (#40).
Since the % contribution of the base peak of stanols (m/z 215) to the TIC showed a rather high RSD of ~ ± 16%, they were determined via M+ which showed a much better performance (Table 2). This selection also enabled a direct quantification of sitostanol (#3), although it was co-eluting with ∆5-avenasterol (#14), which also formed m/z 215 (Fig. 4a; see also Supplemental Material section “Co-elutions”). Similarly, ∆7,SC-desmethylsterols (#15–17) were quantified by m/z 213, as it showed the smallest variation between the three members of this subgroup. In this context, ∆7,SC-desmethylsterols (base peak m/z 213 or m/z 255) could be distinguished from ∆5,SC-desmethylsterols (base peak m/z 129) (Table S2).
GC/MS-SIM ion chromatograms were selected to illustrate several co-eluting silylated sterols in sunflower oil. With #3 sitostanol, #8 ∆7-sitosterol, #9 ∆8-sitosterol, #14 ∆5-avenasterol, #22 cycloartenol, #28 β-amyrin, #29 α-amyrin, and #30 lupeol. Note that the abundance of the ion traces is not directly linked with the amount of the sterol
Although the set of sterols captured 30 representatives, two widely found but always low abundant ∆5,SC-desmethylsterols, 24-methylenecholesterol and ∆5,23-stigmastadienol, could not be used to establish the corresponding SIM factors because GC/MS full-scan spectra of sufficient quality could not be recorded. However, given the reasonable number of ∆5,SC-desmethylsterols that could be used to establish SIM factors of this subgroup, this disadvantage was considered acceptable.
All pentacyclic triterpenols, which were determined via m/z 189, co-eluted with a sterol (Fig. 4b, c; see Supplemental Material section “Co-elutions”). Since both classes formed the “general sterol ion” m/z 129 (Table S2), sterols co-eluting with pentacyclic triterpenols were quantified by M+ (when m/z 189 was detected). Despite a few co-elutions (see Supplemental Material section “Co-elutions”), 28 of the 30 ∆5-, ∆7-, ∆8-, ∆5,SC-, ∆7,SC-desmethylsterols, stanols, 4-methyl-, and 4,4-dimethylsterols, and pentacyclic triterpenols could be quantified by GC/MS-SIM using the mean multiplication factors (response factors) of the respective quantification ion (Fig. 3, Table 1).
Examination of the GC/MS-SIM method by sterol quantification in sunflower oil and pumpkin oil
Seven of the ten sterols available as reference standards were detected in sunflower oil and were quantified by means of calibration functions (Fig. 5, Table S1). Then, the corresponding function was applied to other members of the subgroup. Seven of the ten sterols available as reference standards were detected in sunflower oil and were quantified by means of calibration functions (Fig. 5, Table S1). Then, the corresponding function was applied to other members of the subgroup. Specifically, all C28-desmethylsterols without a reference standard were quantified with campesterol (Fig. 5, subgroup IIa), C29-∆7,SC-desmethylsterols with spinasterol (Fig. 5, subgroup IIIa), whereas residual C29-desmethylsterols were quantified with β-sitosterol (Fig. 5, subgroup IIIc). 4,4-Dimethyl- and 4-methylsterols without a reference standard were quantified with cycloartenol (Fig. 5, subgroup IVb) and all amyrins with α-amyrin (Fig. 5, subgroup Va). Finally, five groups (subgroups Ia, Ib, IIIb, IVa, and V) were quantified with the corresponding reference standard. Altogether, 20 sterols could be quantified by GC/MS-SIM and the 11 most abundant ones of them could also be determined via parallel measurements by GC/FID. The GC/MS amounts of individual sterols deviated by 0–40% from the GC/FID results (Table 3). However, inaccuracies were rather expected in the case of GC/FID due to (i) co-elutions (see below), (ii) an up to 30 times worse limit of quantification (LOQ), and (iii) varying (but uncorrected) response factors. Similarly, the method precision (RSDsample) for the analysis of sunflower oil by GC/MS-SIM (1.2 ± 1.9%) was strikingly better compared to the one by GC/FID (5.7 ± 2.8%). The day-to-day repeatability for sterols in sunflower oil by GC/MS-SIM (determined on three different days in two months) was < ± 10% RSD (mean method precision 6.3 ± 2.0%).
Overview of the 30 sterols and amyrins that were quantified in this study. The ten sterols available as reference standards are printed in bold style. These standards and further sterols were assigned to the following subgroups, with the additional sterols being quantified by means of the reference standard: (Ia) C27-desmethylsterols, (Ib) C27-desmethylstanols, (II) C28-desmethylsterols, (IIIa) C29-∆7,SC-desmethylsterols, (IIIb) stigmasterol, and (IIIc) all other C29-desmethylsterols, (IVa) lanosterol, (IVa) residual 4,4-dimethyl- and 4-methylsterols, (Va) amyrins, and (Vb) lupeol
Due to the individual calibration procedure and the excellent performance, the GC/MS-SIM determination of the presented sterols was considered reliable. Moreover, switching from individual response factors to the mean response factor of a subgroup barely changed the quantified results (Table 3). Hence, mean response factors were applied in the following. The summed-up quantities of the eleven (20) sterols were 430 mg/100 g by GC/FID and 430 mg/100 g (530 mg/100 g) by GC/MS-SIM. The sterol content of individual sterols in the sunflower oil (either based on 11 or 20 sterols) compared well with literature studies (Table 3). However, the number of quantified sterols was much higher in the present study. Further details could not be obtained from the literature because LOD and the quantification method were mostly not specified.
Complementary measurements on a DB-5MS column (system 2) resulted in more co-elutions (e.g., β-sitosterol (#60, index according to Table 3) with β-amyrin (#28t) or cycloartenol (#222) with ∆7-sitosterol (#80)) compared with the DB-5HT column in the main GC/MS system 1. This issue surely has played a role in the GC/FID quantifications, which were also performed with a DB-5MS column. Also, pentacyclic triterpenols and 4,4-dimethyl- and 4-methylsterols were shifted to lower retention times/retention indices compared to desmethylsterols. These differences in the performance were unexpected because both stationary phases represented the classic 95% methyl and 5% phenyl polysiloxane type. Deviations were also observed in the abundance ratios of the SIM ions but this was rather attributed to the impact of different GC/MS instruments. Nevertheless, the proposed response factors need to be individually checked when transferring the method to other devices. Similar observations were also made in the case of the GC/MS analysis of fatty acid methyl esters [25].
In the case of a pumpkin oil (Table S3), which mainly contained ∆7-desmethylsterols, GC/MS-SIM was also superior to GC/FID in terms of number of sterols that could be determined (11 by GC/FID vs. 17 by GC/MS-SIM) and method precision (11.5 ± 2.6% by GC/FID vs. 5.2 ± 3.4% by GC/MS-SIM). Unfortunately, only a few literature studies were available, which nevertheless featured similar sterol contents (Table S3).
Quantification of the 30 sterols in 20 vegetable oil and fat samples—general findings
Finally, 30 sterols were studied in 20 different vegetable oils and between eight (coconut oil) and 21 sterols (wheat germ oil) could be quantified by GC/MS-SIM (Table 4). The average method precision for all sterols was 3.4 ± 1.3%. The total sterol contents in the different oils varied between 100 and 2300 mg/100 g oil (Fig. 6, Table S4). Coconut oil not only contained the lowest number of sterols but also the lowest sterol content (Fig. 6, Table S4). Interestingly, the three oils with the highest total contents (amaranth oil, rice bran oil, and shea butter) were dominated by a different sterol (β-sitosterol (#60), spinasterol (#150), or β-amyrin (#28t), respectively).
The most common phytosterol, β-sitosterol (#60), predominated in 16 oils (80%) and its content varied between < 100 and > 1000 mg/100 g oil (Fig. 6, Table 4). Still, in 60% of the oils, it only ranged between 100 and 400 mg/100 g oil. In shea butter, which mainly consisted of pentacyclic triterpenols (#27t-#30t) and butyrospermol (#252) [26], β-sitosterol (#60) was only present as a minor sterol, while it was not detectable in amaranth oil, pumpkin seed oil, and argan oil (which contained spinasterol (#150) as the main sterol [4, 11, 27]).
Interestingly, Wang et al. detected traces of β-sitosterol (#60) in pumpkin seeds from China (Table S3) [4]. By selecting specific GC/MS-SIM values in the high-mass range (e.g., m/z 343 and m/z 357), Wang et al. were able to determine nine sterols (including β-sitosterol (#60)) with even better sensitivity, while the present GC/MS-SIM method allowed to determine more (virtually all) sterols. Apart from this possibility, (minor) variety-specific changes with impact on the presence or absence of β-sitosterol (#60) could also exist for pumpkin seed oil, similar to variations noticed in amaranth and quinoa accessions [7, 11, 12]. β-Sitosterol (#60) not only co-eluted with spinasterol (#150) (from which it could be distinguished by the present GC/MS-SIM method) but also with lanosterol (#242) and obtusifoliol (#241). Also, these two minor sterols could be distinguished from the up to 40-fold more abundant β-sitosterol (#60) and/or spinasterol (#150), and the presence of (at least) one of them could be confirmed in all samples except coconut oil. Due to virtually identical GC/MS spectra, peak #24 could not be unequivocally assigned to lanosterol (#242) and/or obtusifoliol (#241). However, both sterols were recently separated by SPE and, according to the distribution reported by Schlag et al. [9], lanosterol (#242) was present in six plant oils (Fig. S3). High lanosterol (#242) levels (130 mg/100 g oil) were found in chili seed oil, while medium-to-low contents were detected in the other oils (6.8–60 mg/100 g). Wu et al. also detected lanosterol (#242) in sunflower oil and found similar contents to our study, but not in nine other oils, which were tested positive for lanosterol (#242) in our study. Hitherto, lanosterol (#242) has been known from lanolin, but it was scarcely or not reported [9, 28, 29] in plants, but the genes for lanosterol synthase are known to occur in plants [30]. As until recently the connection between lanosterol (#242) and obtusifoliol (#241) was unknown [9], no differentiation was made in older studies.
Three further prominent ∆5-desmethylsterols (stigmasterol (#100), campesterol (#50), and ∆5-avenasterol (#140)) were detected in at least 17 oils (Table 4). Quantitative data could also be provided for some less studied sterols such as ∆7-desmethylsterols, 4,4-dimethyl- and 4-methylsterols, pentacyclic triterpenols, and cholesterol (#40). Each of them was present in at least 75% of the oils. Cholesterol (#40) contributed to the total sterols mostly < 1%, which corresponded with very low amounts (0.4–2.6 mg/100 g oil, two times non-detectable). Cholesterol (#40) had only rarely been quantified in vegetable oils [2, 3], since the low amounts and shares can only be determined with a very sensitive method. However, unusually high cholesterol (#40) levels were detected in mustard oil and chili seed oil (> 30 mg/100 g oil), which is in agreement with previous studies [31, 32]. Cholestanol (#10) was only quantifiable in chili seed oil where it reached 14% of the amount of cholesterol (#40) (Table 4). In mustard seed oil, cholestanol (#10) would have been detectable, if it had a similar abundance ratio with cholesterol (#40) as in chili seed oil, which was not the case. In all other samples with much lower cholesterol (#40) content, it was not surprising that cholestanol (#10) could not be detected.
Also, sitostanol (#30) was quantified in 18 oils (90%) at typically 5% of the amount of β-sitosterol (#60) (Table 4). Different from that, the present argan oil, amaranth oil, and pumpkin seed oil only featured sitostanol (#30) but not β-sitosterol (#60) (Table 4). However, these three plant oils contained ∆7-sitosterol (#60, also known as ∆7-stigmastenol (Table S2)), which is the precursor of β-sitosterol (#60) in the biosynthesis [33], and spinasterol (#150) (Table 4).
Detected in 15 oils (75%), campestanol (#20) was particularly rich in corn oil and wheat germ oil, and rice bran oil [3, 17, 34]. Thirteen of the 15 oils with campestanol (#20) also contained much higher amounts of campesterol (#50), and the exception were again argan oil and pumpkin oil (Table 4). However, campestanol (#50) had not been reported before in argan oil. The present pumpkin oil sample contained similar contents of campestanol (#20) and campesterol (#50), while Wang et al. found 3 times higher contents of campesterol (#50) in pumpkin seeds from China [4]. Accordingly, the abundance ratio of campesterol (#50) and campestanol (#20) may vary in pumpkin oils due to currently unknown reasons.
Amyrins could be quantified in 75% of the samples. β-Amyrin (#28t) and α-amyrin (#29t) were frequently co-occurring at similar levels (~ 50% of the positive tested samples). Zhang et al. detected both amyrins at similar levels in some (sunflower, safflower, and sea buckthorn oil) but not all oils investigated in the present study [15]. Apart from the very high contents in shea butter [15], the levels were relatively low (< 10 mg /100 g oil). Lupeol (#30t) could only be quantified in shea butter, as it was superimposed by cycloartenol (#222) in all other oils (due to the co-elution only the main sterol was quantified, see Supplemental Material section “Co-elutions”). In addition to shea butter, δ-amyrin (#27t) was also detected and quantified in argan oil. Due to its scarcity, it may serve as a potential marker for this valuable oil. Specifically, the presence of δ-amyrin (#27t) and spinasterol (#150) could be suggested to get first indications on the sole presence of argan oil.
Comparably high contents of 4,4-di- and 4-methylsterols were detected in chili seed oil and linseed oil (cycloartenol (#222) > 100 mg/100 g oil) and olive oil (24-methylenecycloartanol (#232) > 50 mg/100 g oil). Besides, amaranth oil and rice bran oil were also rich in 4,4-di- and 4-methylsterols (more than 100 mg/100 g oil) (Fig. 6). These findings (except for chili seed oil) are in agreement with the data of Zhang et al. [15] and León-Camacho et al. [35], but in the case of amaranth mainly qualitative.
Amaranth oil, pumpkin seed oil, and argan oil, not only featured spinasterol (#150) as main compound, but also contained higher amounts of stigmasta-7,25-dienol (#130) and ∆7(,SC)-desmethylsterols (Fig. 7, Table 4). However, also sunflower oil, safflower oil, and sea buckthorn oil stood out with ~ 25% ∆7-desmethylsterols. Li et al. and Mahboobeh et al. also found high shares of ∆7-desmethylsterols in safflower oil and/or sea buckthorn oil [36, 37]. By contrast, ∆7-desmethylsterols were low (e.g., for ∆7-sitosterol (#80) [2]) or unreported [4, 5] in sunflower oil.
After exclusion of the main sterol of each oil, the residual sterols contributed between 40 and 73% to the total sterol content (Fig. 7). Furthermore, (other) ∆5-desmethylsterols still contributed with < 60% to the minor sterols in 75% of the oils. In particular, oils dominated by spinasterol (#150) or α-amyrin (#29t) contained low amounts of ∆5-desmethylsterols (< 20%). On the contrary, all vegetable oils dominated by β-sitosterol (#60) contained > 20% of (further) ∆5-desmethylsterols. Unfortunately, ∆7-desmethylsterols or 4,4-di- and 4-methylsterols were scarcely quantified in other publications, most likely due to the lack of reference standards. However, an exclusion of these underrepresented sterols partly resulted in substantially lower total sterol contents. Also, this example illustrates the advantage of the present GC/MS-SIM method which enables their quantification without having a reference standard at hand.
Conclusions
The application of mean response factors was successfully introduced for the novel GC/MS-SIM quantification method. Comparative measurements of two different oils showed that the method was superior to GC/FID measurements. The method enabled the quantification of minor sterols due to (i) the much better sensitivity and (ii) the possibility to quantify sterols without a standard. Between eight and 21 sterols and pentacyclic triterpenols were quantified in 18 vegetable oils and two vegetable fats, which is a much higher number than has been quantified before. This data may be useful for food authentication, e.g., the presence of δ-amyrin (#27t) in argan oil. The developed quantification method may also be applied to the quantitative analysis of further unknown sterols, rare oils, or plant parts.
Data availability
The raw datasets generated during the current study are available from the corresponding author on reasonable request.
References
Cert A, Moreda W, Pérez-Camino MC (2000) Chromatographic analysis of minor constituents in vegetable oils. J Chromatogr A 881(1–2):131–148. https://doi.org/10.1016/S0021-9673(00)00389-7
Xu B, You S, Zhou L et al (2020) Simultaneous determination of free phytosterols and tocopherols in vegetable oils by an improved SPE-GC-FID method. Food Anal Methods 13:358–369. https://doi.org/10.1007/s12161-019-01649-7
Schwartz H, Ollilainen V, Piironen V et al (2008) Tocopherol, tocotrienol and plant sterol contents of vegetable oils and industrial fats. J Food Compos Anal 21(2):152–161. https://doi.org/10.1016/j.jfca.2007.07.012
Wang M, Zhang L, Wu X et al (2019) Quantitative determination of free and esterified phytosterol profile in nuts and seeds commonly consumed in China by SPE/GC-MS. LWT 100:355–361. https://doi.org/10.1016/j.lwt.2018.10.077
Verleyen T, Forcades M, Verhé R et al (2002) Analysis of free and esterified sterols in vegetable oils. J Am Oil Chem Soc 79(2):117–122. https://doi.org/10.1007/s11746-002-0444-3
Moreau RA, Nyström L, Whitaker BD et al (2018) Phytosterols and their derivatives: Structural diversity, distribution, metabolism, analysis, and health-promoting uses. Prog Lipid Res 70:35–61. https://doi.org/10.1016/j.plipres.2018.04.001
Schlag S, Götz S, Rüttler F et al (2022) Quantitation of 20 phytosterols in 34 different accessions of quinoa (Chenopodium quinoa). J Agric Food Chem 70(32):9856–9864. https://doi.org/10.1021/acs.jafc.2c03374
Kushiro T, Shibuya M, Masuda K et al (2000) A novel multifunctional triterpene synthase from Arabidopsis thaliana. Tetrahedron Lett 41(40):7705–7710. https://doi.org/10.1016/S0040-4039(00)01347-2
Schlag S, Bräckle Y, Jelečević M et al (2023) Gas chromatography with mass spectrometry analysis of 4,4-dimethylsterols and 4-methylsterols in edible oils after their enrichment by means of solid phase extraction. J Chromatogr A 1705:464166. https://doi.org/10.1016/j.chroma.2023.464166
Sommer K, Krauß S, Vetter W (2020) Differentiation of European and chinese truffle (Tuber sp.) species by means of sterol fingerprints. J Agric Food Chem 68(49):14393–14401. https://doi.org/10.1021/acs.jafc.0c06011
Dailey OD, Severson RF, Arrendale RF (1997) Nonpolar lipids of Amaranthus palmeri S. Wats. 2. Unsaturated esters and free fatty acids, sterols, and triterpenols. J Agric Food Chem 45(10):3914–3920. https://doi.org/10.1021/jf960708q
Ogrodowska D, Zadernowski R, Czaplicki S et al (2014) Amaranth seeds and products—the source of bioactive compounds. Pol J Food Nutr Sci 64(3):165–170. https://doi.org/10.2478/v10222-012-0095-z
Fiebig H-J, Brühl L, Aitzetmüller K (1998) Sterine—Isolierung und gaschromatographische Untersuchung. Lipid/Fett 100(9):422–428. https://doi.org/10.1002/(SICI)1521-4133(199809)100:9%3c422:AID-LIPI422%3e3.0.CO;2-7
Phillips KM, Ruggio DM, Toivo JI et al (2002) Free and esterified sterol composition of edible oils and fats. J Food Compos Anal 15(2):123–142. https://doi.org/10.1006/jfca.2001.1044
Zhang T, Xie L, Liu R et al (2020) Revisiting the 4,4-dimethylsterols profile from different kinds of vegetable oils by using GC-MS. LWT 124:109163. https://doi.org/10.1016/j.lwt.2020.109163
Feng S, Wang L, Shao P et al (2022) Simultaneous analysis of free phytosterols and phytosterol glycosides in rice bran by SPE/GC-MS. Food Chem 387:132742. https://doi.org/10.1016/j.foodchem.2022.132742
Bai G, Ma C, Chen X (2021) Phytosterols in edible oil: distribution, analysis and variation during processing. Grain Oil Sci Technol 4(1):33–44. https://doi.org/10.1016/j.gaost.2020.12.003
Santanatoglia A, Nzekoue FK, Sagratini G et al (2023) Development and application of a novel analytical method for the determination of 8 plant sterols/stanols in 22 legumes samples. J Food Compos Anal 118:105195. https://doi.org/10.1016/j.jfca.2023.105195
Rüttler F, Hammerschick T, Schlag S et al (2022) Isolation of lanosterol and dihydrolanosterol from the unsaponifiable matter of lanolin by urea complexation and countercurrent chromatography in heart-cut recycling mode. J Chromatogr B 1210:123470. https://doi.org/10.1016/j.jchromb.2022.123470
Schlag S, Huang Y, Vetter W (2022) GC/EI-MS method for the determination of phytosterols in vegetable oils. Anal Bioanal Chem 414(2):1061–1071. https://doi.org/10.1007/s00216-021-03730-9
Sommer K, Vetter W (2020) Gas chromatography with mass spectrometry detection and characterization of 27 sterols in two truffle (Tuber) species. J Food Compos Anal 94:103650. https://doi.org/10.1016/j.jfca.2020.103650
Schröder M, Vetter W (2011) High-speed counter-current chromatographic separation of phytosterols. Anal Bioanal Chem 400(10):3615–3623. https://doi.org/10.1007/s00216-011-4995-2
Audier HE, Beugelmans R, Das BC (1966) Mass spectrometry of tetracyclic triterpenes part II. The lanostane group: influence of the 9:19-cyclopropane ring. Tetrahedron Lett 7(36):4341–4347. https://doi.org/10.1016/S0040-4039(00)76062-X
Goad J, Akihisa T (1997) Analysis of Sterols. Blackie Academic & Professional, London
Thurnhofer S, Vetter W (2005) A gas chromatography/electron ionization-mass spectrometry-selected ion monitoring method for determining the fatty acid pattern in food after formation of fatty acid methyl esters. J Agric Food Chem 53(23):8896–8903. https://doi.org/10.1021/jf051468u
Di Vincenzo D, Maranz S, Serraiocco A et al (2005) Regional variation in shea butter lipid and triterpene composition in four African countries. J Agric Food Chem 53(19):7473–7479. https://doi.org/10.1021/jf0509759
Khallouki F, Younos C, Soulimani R et al (2003) Consumption of argan oil (Morocco) with its unique profile of fatty acids, tocopherols, squalene, sterols and phenolic compounds should confer valuable cancer chemopreventive effects. Eur J Cancer Prev 12(1):67–75. https://doi.org/10.1097/01.cej.0000051106.40692.d3
Wu L, Zhao J, Wu L et al (2022) Simultaneous determination of squalene, tocopherols and phytosterols in edible vegetable oil by SPE combined with saponification and GC-MS. LWT 169:114026. https://doi.org/10.1016/j.lwt.2022.114026
Itoh T, Tamura T, Matsumoto T (1973) Methylsterol compositions of 19 vegetable oils. J Am Oil Chem Soc 50(8):300–303. https://doi.org/10.1007/BF02641360
Suzuki M, Xiang T, Ohyama K et al (2006) Lanosterol synthase in dicotyledonous plants. Plant Cell Physiol 47(5):565–571. https://doi.org/10.1093/pcp/pcj031
Kozłowska M, Gruczyńska E, Ścibisz I et al (2016) Fatty acids and sterols composition, and antioxidant activity of oils extracted from plant seeds. Food Chem 213:450–456. https://doi.org/10.1016/j.foodchem.2016.06.102
Matthäus B, Özcan MM (2009) Chemical evaluation of some paprika (Capsicum annuum L.) seed oils. Eur J Lipid Sci Technol 111(12):1249–1254. https://doi.org/10.1002/ejlt.200900036
Nes WD (2011) Biosynthesis of cholesterol and other sterols. Chem rev 111(10):6423–6451. https://doi.org/10.1021/cr200021m
van Hoed V, Depaemelaere G, Ayala JV et al (2006) Influence of chemical refining on the major and minor components of rice brain oil. J Am Oil Chem Soc 83(4):315–321. https://doi.org/10.1007/s11746-006-1206-y
León-Camacho M, García-González DL, Aparicio R (2001) A detailed and comprehensive study of amaranth (Amaranthus cruentus L.) oil fatty profile. Eur Food Res Technol 213:349–355. https://doi.org/10.1007/s002170100340
Li TSC, Beveridge THJ, Drover JCG (2007) Phytosterol content of sea buckthorn (Hippophae rhamnoides L.) seed oil: extraction and identification. Food Chem 101(4):1633–1639. https://doi.org/10.1016/j.foodchem.2006.04.033
Mahboobeh VM, Ghavamib M, Gharachorloo M et al (2011) Lipid composition and oxidative stability of oils in safflower (Carthamus tinctorius L.) seed varieties grow in Iran. Adv Envirom Biol 5(5):897–902
Acknowledgements
W.V. acknowledges funding by Deutsche Forschungsgemeinschaft (DFG), and S.S. acknowledges initial financial support by Landesgraduiertenförderung Baden-Wuerttemberg.
Funding
Open Access funding enabled and organized by Projekt DEAL. Research in this field was funded by the Deutsche For-schungsgemeinschaft (DFG) via grant no. VE 164/20-1.
Author information
Authors and Affiliations
Corresponding author
Ethics declarations
Conflict of interest
The authors declare that they have no conflict of interest.
Ethical approval
All authors comply with Springer’s ethical policies regarding conflict of interest, informed consent, and ethical treatment of humans and animals in research.
Additional information
Publisher's Note
Springer Nature remains neutral with regard to jurisdictional claims in published maps and institutional affiliations.
Supplementary Information
Below is the link to the electronic supplementary material.
Rights and permissions
Open Access This article is licensed under a Creative Commons Attribution 4.0 International License, which permits use, sharing, adaptation, distribution and reproduction in any medium or format, as long as you give appropriate credit to the original author(s) and the source, provide a link to the Creative Commons licence, and indicate if changes were made. The images or other third party material in this article are included in the article's Creative Commons licence, unless indicated otherwise in a credit line to the material. If material is not included in the article's Creative Commons licence and your intended use is not permitted by statutory regulation or exceeds the permitted use, you will need to obtain permission directly from the copyright holder. To view a copy of this licence, visit http://creativecommons.org/licenses/by/4.0/.
About this article
Cite this article
Schlag, S., Vetter, W. Quantitative data of up to thirty sterols in vegetable oils and fats. Eur Food Res Technol 250, 469–482 (2024). https://doi.org/10.1007/s00217-023-04397-5
Received:
Revised:
Accepted:
Published:
Issue Date:
DOI: https://doi.org/10.1007/s00217-023-04397-5