Abstract
Adipose tissue CD11c+ myeloid cell is an independent risk factor associated with obesity and metabolic disorders. However, the underlying molecular basis remains elusive. Here, we demonstrated that liver kinase B1 (Lkb1), a key bioenergetic sensor, is involved in CD11c+ cell-mediated immune responses in diet-induced obesity. Loss of Lkb1 in CD11c+ cells results in obesity resistance but lower glucose tolerance, which accompanies tissue-specific immune abnormalities. The accumulation and CD80’s expression of Lkb1 deficient adipose-tissue specific dendritic cells but not macrophages is restrained. Additionally, the balance of IL-17A and IFN-γ remarkably tips towards the latter in fat T cells and CD11c− macrophages. Mechanistically, IFN-γ promotes apoptosis of preadipocytes and inhibits their adipogenesis while IL-17A promotes the adipogenesis in vitro, which might account in part for the fat gain resistant phenotype. In summary, these findings reveal that Lkb1 is essential for fat CD11c+ dendritic cells responding to HFD exposure and provides new insights into the IL-17A/IFN-γ balance in HFD-induced obesity.
Similar content being viewed by others
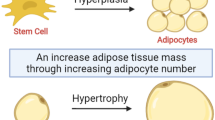
Avoid common mistakes on your manuscript.
Introduction
The immune and metabolic systems are critical for species survival and share many underpinnings [1]. Obesity, a representative metabolic immune disorder in adipose tissue, has become a serious public concern over the past few decades [2,3,4]. Adipose tissue plays a systemically metabolic role and is also an endocrine immune organ expressing high levels of adiponectin to preserve systemic insulin sensitivity and adipose immune balance [5]. However, continuous overnutrition can lead to pathological expansion of adipocytes. Excess adiposity makes the enlarged adipocytes more susceptible to endoplasmic reticulum stress and death, resulting in local hypoxia and ectopic lipids. These damage-associated molecular patterns and metabolic signals recruit dendritic cells, macrophages, and other immune cells and initiate proinflammatory responses such as TNF-α secretion, which further disrupts the metabolic function of adipocytes. Such a metabolic-immune vicious cycle alters systemic glucose and lipid metabolism and insulin action, leading to metabolic diseases, including insulin resistance and type 2 diabetes [1, 6,7,8].
Various immune cells and immune cytokines compose the adipose tissue immune environment. Macrophages, dendritic cells (DC), CD4+ Th1/17 cells, CD8+ cytotoxic T cells, B cells, and mast cells are recognized as pro-inflammatory cells, which are responsible for the metabolic pathogenesis of obesity. CD4+Foxp3+ regulatory T cells (Treg), Th2 cells, and eosinophils are recognized as protective cells that maintain a healthy status and insulin sensitivity [9,10,11]. Among these immune populations, CD11c+ cells are considered a therapeutic target for obesity-associated metabolic disorders because ablation of this population could alleviate the obese-induced insulin sensitivity [12,13,14,15,16]. Fat CD11c+ cells are composed of adipose tissue macrophages (ATM) and dendritic cells (ATDC), which could be clearly distinguished with CD64 and CD11c in CD45+cells [14, 17]. There is another well-recognized gating strategy as “CD45+MHCII+CD11chi” to identify ATDC [13].
Treg-mediated immune suppression plays a central role in minimizing harmful immune responses and maintaining peripheral tolerance [18]. Visceral adipose tissue (VAT) resident Tregs are more abundant than lymphoid Tregs and exhibit sex-specificity; they are derived from thymic Tregs during the early weeks after birth. Their expansion is dependent on antigens presented by DCs and cytokines such as IL33. It could also be recruited via the CCL2-CCR2 axis. Notably, VAT Tregs are specifically and strikingly reduced in obese mice with metabolic disorders [19,20,21,22]. Surrogate agonist peptides that cause VAT Tregs expansion could effectively protect the AT from inflammation and improve metabolic indices [23]. These results indicate that VAT Tregs are also promising therapeutic targets to restrain the metabolic-immune vicious cycle and improve metabolic disease.
Liver kinase B1 (Lkb1), as a serine-threonine kinase, is defective in cancer-susceptible Peutz–Jeghers syndrome [24]. Our previous study revealed that Lkb1 stabilizes the expression of Foxp3 and maintains Tregs lineage stability [25]. In addition, Lkb1 deletion in CD11c+ dendritic cells can strongly promote lymphoid Tregs expansion in contact manner [26, 27]. It has also been reported that Lkb1 coordinates metabolic and immune quiescence of DCs to enforce antitumor immunity [28]. However, the specific role of Lkb1 in CD11c+ myeloid cells in diet-induced obesity and VAT Tregs has not yet been studied.
In the present study, we generated CD11cCre Lkb1f/f mice to elucidate the role Lkb1 of in CD11c+ cells under high-fat diet exposure. We found that Lkb1 deletion in CD11c+ cells leads to an obesity-resistant phenotype in a high-fat diet (HFD)-induced obesity (DIO) model. We dissected the differences in adipose tissue immune profile between CD11cCre Lkb1f/f mice and Lkb1f/f mice. The results showed that, the lost of Lkb1 in CD11c+ cells caused several changes regarding immune factors. 1. Reduction in ATDC’s accumulation and CD80 expression; 2. The advantage of VAT Tregs in CD11cCre Lkb1f/f mice narrowed in DIO model; 3. The effector-cytokine balance of IL-17A and IFN-γ tips towards the latter in both VAT-T cells and ATMs. Mechanistically, the addition of IFN-γ inhibits the differentiation of preadipocytes into adipocytes and increases apoptosis while IL-17A promotes the adipogenesis ex vivo. Thus, these findings reveal a dominant role of CD11c+ dendritic cells’ Lkb1 in shaping the immune microenvironment of adipose tissue and provide new insights into the IL-17A/IFN-γ balance in HFD-induced obesity.
Material and methods
Mice and diet
All animals were raised in specific pathogen-free barrier (SPF) facilities. We used these animals following the protocol approved by the Institutional Animal Care and User Committee at the Institute of Hematology, Chinese Academy of Medical Sciences. CD11cCre and Lkb1f/f mice purchased from Jackson Laboratories (Bar Harbor, ME, USA) were backcrossed with C57BL/6 mice for at least seven generations. Lkb1f/f mice were hybridized with CD11cCre ones to produce CD11cCreLkb1f/f mice. In control and obesity group, male mice were fed with an NFD (product data-D12450B, 3.85 kcal/g, 20% protein, 10% fat, 70% carbohydrates) [29] and an HFD (research diets D12492, 5.24 kcal/g, 20% protein, 60% fat, 20% carbohydrates), respectively, from 6 weeks of age [15], for 16 weeks. We housed the different genotypic littermates in one cage for the same experiments.
Metabolic evaluation
From the beginning of HFD feeding, the body weight of the experimental mice was measured weekly, and fasting blood glucose was measured every four weeks after 12 h of fasting during the night (around 8:00 p.m.–8:00 a.m.).
Intraperitoneal glucose tolerance test (IPGTT)
The mice were fasted for 6 h and then intraperitoneal injection with glucose (1.0 g/kg), and the blood glucose concentration (mmol/L) was measured with a glucose meter at 0, 15, 30, 60, and 120 min after injection [15, 30]. The area under the curve (AUC) was obtained by calculating the space between the x-axis and a given curve using GraphPad Prism software (GraphPad Software, version 7.0a).
Intraperitoneal insulin tolerance test (IPITT) and insulin secretion
The mice were fasted for 6 h and then intraperitoneal injection with insulin (1.0 U/kg), and the blood glucose concentration (mmol/L) was measured with a glucose meter at 0, 15, 30, 60, 90, and 120 min after injection [15, 30]. Blood insulin was tested during the glucose tolerance test [31].
Fat-associated stromal vascular fraction (SVF) cell isolation
Epididymal fat pads (VAT) and subcutaneous adipose tissue (SAT) around the lower limbs of male CD11cCreLkb1f/f and Lkb1f/f mice fed an NFD or HFD for 16–20 weeks were surgically dissected and weighed. The AT was chopped with scissors and digested by rotation with 1 mg/mL collagenase at 37 °C (Sigma-Aldrich, Germany) for 30 min. Digestion was terminated by Dulbecco's modified Eagle's medium (DMEM/F-12) (Gibco, USA) with 2% fetal bovine serum (FBS), followed by centrifugation at 1600 rpm for 5 min. The upper layer of mature adipocytes was discarded, and the precipitated interstitial vascular fraction was further purified by density gradient centrifugation to obtain the immune cells.
Adipocyte measurement
The AT obtained by dissection was fixed in 10% formalin. Sections were then stained with hematoxylin–eosin staining, observed with a microscope (100 ×), and analyzed with ImageJ software [15]. Three regions were randomly collected, and the average cell area was determined by calculating the number of cells and the total size in the region.
Flow cytometry
Single-cell suspensions were prepared from the spleen and VAT and stained with PE-MHC-II (107613, Biolegend), APC-cy7-CD11c (117323, Biolegend), FITC-CD64 (139315, Biolegend), Percp-cy5.5-CD45.2 (109827, Biolegend), APC-CD45 (147707, Biolegend), FITC-CD4 (100405, Biolegend), APC-CD8 (126613, Biolegend), PE-cy7-CD62L (104417, Biolegend), Percp-cy5.5-CD44 (103031, Biolegend), APC-cy7-FVD, or Pacific blue-DAPI. Intracellular staining was performed using PE-Foxp3 (12-5773-82, eBioscience) and antibodies against cytokines, including BV421-CD8, PE-cy7-IFN-γ (505825, Biolegend), Percp-cy5.5-IL-17A (506919, Biolegend), and APC-IL-4 (504105, Biolegend). Cells from the spleen and VAT were treated with phorbol myristate acetate (PMA, 50 ng/mL) and ionomycin (500 ng/mL) (Sigma-Aldrich) for 4.5 h. Then the cytokines in specific cell populations were analyzed. The cells were stained at 4 °C for 30 min or 1 h for surface and intracellular staining, respectively, followed by detection using the FACS Canto II (BD Biosciences) system and analysis of the data using FlowJo (Tree Star, version 10.0).
Real-time quantitative PCR
RNA of adipose tissue was extracted using TRIzol reagent (Life Technologies, Grand Island, NY). 50 mg adipose tissue/sample was ground into homogenization in liquid nitrogen before RNA extraction. We employed the QuantiTect Reverse Transcription kit (Qiagen, Hilden, Germany) to reverse mRNA into cDNA. For quantitative RT–PCR (qPCR), aliquots of cDNA were employed for Fast SYBR green qPCR (Applied Biosystems, Foster City, USA) and quantified with the QuantStudio 5 Real-Time PCR System (Applied Biosystems, Foster City, USA). The sequence of primers are summarized in the Supplementary Table 1.
ELISA
Serum insulin concentrations of Lkb1f/f and CD11cCreLkb1f/f mice were detected by ELISA (EZRMI-13 K, Merckmillipore) following the manufacturer's instructions. The blood collections are random and without fasting in Fig. 3E. The insulin secretion experiment followed the IPGTT in Fig. 4F.
Cholesterol, triglyceride, and glucose measurements
Total cholesterol and triglycerides were measured using the Sigma kit, and free cholesterol was measured using the Wako kit (Richmond, VA). Glucometers (Bayer, Elkhart, IN, USA) was used to measure blood glucose levels. The blood collections are random and without fasting in Fig. 3G.
Isolation and in vitro differentiation assay of mice preadipocytes
Epididymal fat pads from male Lkb1f/f mice were surgically dissected. The AT digestion and extraction methods were the same as those described above for SVF isolation. The suspended mature adipocytes were discarded, and the precipitated interstitial vascular fraction was cultured in DMEM/F-12 supplemented with 10% FBS. After two-day cell confluence (designated as day 0), we washed and discarded the suspended cells, and the confluent cells are mostly the preadipocytes. Preadipocyte differentiation was carried out with DMEM/F-12 containing 10% FBS and three kinds of induction agents (0.75 mM isobutylmethylxanthine (IBMX), 1.5 μM dexamethasone, and 1.5 μg/mL insulin) for three days. The cells were then incubated in a 10% FBS/DMEM/F-12 culture medium with 1.5 μg/mL insulin and 25, 50 ng/mL IFN-γ or IL-17A, and the IFN-γ or IL-17A-free group was used as the control. The culture medium with or without IFN-γ or IL-17A was refreshed every other day. On day 14, the cells were washed twice with phosphate-buffered saline (PBS) and stained with Oil Red O solution for 20 min at room temperature. Stained Oil Red O was eluted with isopropanol after the cells were washed, and the differentiation capacity of preadipocytes was determined by measuring the optical density (OD) at 510 nm [32, 33].
Detection of mice preadipocyte size, proliferation, and apoptosis
After 24-h adherent preadipocytes enrichment, the culture medium was replaced with a complete medium containing 50 ng/mL IFN-γ or IFN-γ-free medium for 72 h. Then, the cells were digested with trypsin and harvested for downstream flow cytometry analysis. The FITC Annexin V Apoptosis Detection Kit with PI (640,914, Biolegend) was used to detect apoptosis, while proliferation was determined by the expression of Ki-67 (652,409, Biolegend).
Statistics
Differences between the two groups were determined using an unpaired, two-tailed Student’s t-test, and one-way ANOVA was performed to compare more than two groups using Graph Pad Prism 7 software. Values are presented as mean ± SEM or mean ± SD. Statistical significance was set at P < 0.05 (*P < 0.05, **P < 0.01, ***P < 0.001, ****P < 0.0001).
Results
CD11cCreLkb1f/f mice exhibit obesity resistance in the diet-induced obesity model.
CD11c+ myeloid cells are active members in obese adipose tissue [16, 17, 34]. Lkb1 is considered a dominant energy sensor and mediator of cellular response by activation of AMP-activated protein kinase (AMPK) [35]. We therefore compared the expression of LKB1 and AMPKA in ATDC and ATM among fasting vs fed and NFD vs HFD (Fig. S1A-B). Among the fasting vs fed cohort, the results showed that these two genes in ATDC rather than ATM sensitively responded to starvation, in which LKB1 increased significantly while the AMPKA on the opposite. As for the NFD vs HFD cohort, we observed a significant increase in AMPKA’s expression in ATDC rather ATM. We further compared the expression of LKB1 between splenic DC and ATDC, the splenic DC has a higher level of LKB1 than ATDC, meanwhile, both splenic DC and ATDC has a higher level of LKB1 than their macrophage counterparts (Fig. S1C). To investigate the role of Lkb1 in adipose tissue CD11c+ cells, we thus generated Lkb1 conditional knockout CD11cCreLkb1f/f mice. The knockout deficiency of Lkb1 in CD11c+ cells was determined in our previous study at protein levels. We further tested the Lkb1 in CD11C+ CD64- splenic DC and CD11C+ CD64+ splenic macrophages from the CD11cCreLkb1f/f and Lkb1f/f mice at mRNA levels, which demonstrate that the knockout efficiency is significant (Fig. S1D). We then established a high fat diet-induced obesity (DIO) model using CD11cCreLkb1f/f and Lkb1f/f mice. Notably, CD11cCre Lkb1f/f mice gained lower body weight than their wild-type Lkb1f/f littermates, especially when exposed to HFD (Fig. 1A–C). With interest, we weighed various tissues and observed that adipose tissues (VAT and SAT) but not liver are responsible for the lower body weight of CD11cCreLkb1f/f than Lkb1f/f mice upon HFD challenge (Fig. 1D, E). The histology examination further indicated that the average area of adipocytes from VAT and SAT in CD11cCreLkb1f/f mice was significantly smaller than that in Lkb1f/f mice when fed with HFD (Fig. 2A–D). In addition, the livers from both genotypic mice have generated obvious steatosis and there is no significant difference in liver steatosis between CD11cCreLkb1f/f and Lkb1f/f mice (Fig. S1H). And we calculated the liver versus body weight, there is no difference observed between NFD and HFD (Fig. S1I). These results collectively suggested that CD11cCre Lkb1f/f mice displayed a fat gain resistant phenotype in the DIO model featured with limited expansion of adipocytes.
Loss of Lkb1 in CD11c+ cells leads to decreased weight gain of adipose tissue in the diet-induced obesity model. A Body weight monitoring of mice in the normal fat diet group beginning at week 6 (n = 4). And statistical analysis of the area under the curve (AUC). B Body weight of mice in the high-fat diet group beginning at week 6 (n = 4). And statistical analysis of AUC. C Representative images of Lkb1f/f and CD11cCreLkb1f/f mice after 16 weeks of HFD. D Tissue weight of VAT, SAT, spleen, liver, kidney, pancreas, heart, and lung from Lkb1f/f and CD11cCreLkb1f/f mice after 16 weeks NFD or HFD feeding. E Weight of visceral adipose tissue (VAT) and subcutaneous adipose tissue (SAT) standardized by body weight. The results are presented as the mean ± SD, n = 4–10, *P < 0.05, **P < 0.01, ***P < 0.001, ****P < 0.0001. Black asterisks represent multiple t-test (A, B, D, E) and the red asterisk represent two-way ANOVA (B)
Limited expansion of adipocyte in CD11cCreLkb1f/f mice is partially responsible for the weight-gain resistance of adipose tissue. A, C Representative H&E staining images of VAT and SAT from the indicated group. B, D Statistical analysis of mean size of adipocytes in VAT and SAT from the indicated group. Every counted adipocyte area was determined by at least an unbiased 100 adipocytes per image, and each indicated group selected four images. This result was analyzed by ImageJ. The results are presented as the mean ± SD, *P < 0.05, **P < 0.01, ***P < 0.001, ****P < 0.0001, by Student's t-test
CD11cCreLkb1f/f mice display normal energy intake and expenditure but a complex expression of UCP1.
To determine whether the body weight difference between CD11cCreLkb1f/f and Lkb1f/f mice is derived from the difference energy intake or energy expenditure [36], we measured the food intake and rectal temperature. The results showed that there is no difference between two genotypic mice under HFD feeding in both perspectives (Fig. S1E-F). Additionally, we examined the expression of uncoupling protein 1 (UCP1) in VAT from HFD fed mice and BAT from NFD fed mice. Consistent with the previous knowledge [37], UCP1 is specifically expressed in BAT as the expression levels in BAT was 100 times higher than VAT. Additionally, we observed that BAT from CD11cCreLkb1f/f mice had a significant and 1.44 times higher than Lkb1f/f mice (Fig. S1G). However, CD11Ccre LKB1f/f mice had significantly lower expression of UCP1 in VAT (Fig. S1G, I) compared to Lkb1f/f mice.
CD11cCreLkb1f/f mice display impaired glucose metabolism on HFD feeding.
Obesity and systemic metabolism disorder are closely associated [8, 38]. To explore whether the obesity resistance of CD11cCreLkb1f/f mice has protective roles in systemic metabolism, we performed a series of tests regarding glucose and lipid metabolism. There is no significant difference in fasting blood glucose between NFD-fed CD11cCreLkb1f/f and Lkb1f/f mice, whereas CD11cCreLkb1f/f mice had a moderately higher levels of fasting blood glucose than Lkb1f/f mice post 11 and 15 weeks of HFD feeding. However, the difference was narrowing down after 11 weeks post HFD feeding (Fig. 3A, B). We further performed intraperitoneal glucose tolerance testing (IPGTT), which showed that HFD induces a higher level of blood glucose and area under the curve (AUC) in CD11cCreLkb1f/f mice than that in Lkb1f/f mice (Fig. 3C), meanwhile, CD11cCreLkb1f/f mice had a tendency to higher glucose intolerance but without a significant difference compared with Lkb1f/f mice (Figure S2A). On the other hand, there is no significant difference in the intraperitoneal insulin tolerance test (IPITT) between CD11cCreLkb1f/f and Lkb1f/f mice in both NFD and HFD conditions (Fig. 3D, S2B). Additionally, CD11cCreLkb1f/f mice had slightly lower levels of serum insulin than Lkb1f/f mice both in NFD and HFD conditions with no statistical significance (Fig. 3E). Considering the body weight itself might affect the body metabolism, we therefore measured the IPGTT and ATDC composition in an age and weight-matched cohort. The results showed that CD11cCreLkb1f/f mice still have significant glucose intolerance compared to the Lkb1f/f mice (Fig S2 C-E), but the differences narrow down when compare with the random cohort (Fig. 3D). Meanwhile, we measured the insulin levels during the IPGTT to determine the function of islet in weight matched Lkb1f/f and CD11cCreLkb1f/f mice fed with HFD (Fig. 3F, S2C-E). The results showed that KO mice secreted a higher level of insulin but impaired to reduce glucose compared to WT mice (Fig. 3F, S2E). As for the lipid indices, no significant differences between CD11cCreLkb1f/f mice and Lkb1f/f mice, including cholesterol, high-density lipoprotein, low-density lipoprotein, and triglyceride (Fig. 3F). Taken together, these results indicated that obesity-resistant CD11cCreLkb1f/f mice could normalize fat-induced insulin resistance to some extent, slightly increases glucose intolerance, and has no apparent effects on systemic lipid metabolism in diet induced obesity model.
Lkb1 deficiency in CD11c+ myeloid cells impairs glucose tolerance and improves insulin sensitivity while does not alter systemic lipid metabolism. A, B Fasting blood glucose of Lkb1f/f and CD11cCreLkb1f/f mice on NFD (A) and HFD (B) at indicated time point. C Intraperitoneal glucose tolerance test (IPGTT) of mice after 16 weeks of HFD feeding. And statistical analysis of the area under the curve (AUC) of the IPGTT from Lkb1f/f and CD11cCreLkb1f/f mice. D Intraperitoneal insulin tolerance test (IPITT) of mice after 20 weeks of HFD feeding and statistical analysis of AUC. E Insulin detection of mice fed with NFD or HFD. F Insulin detection during IPGTT experiment in Supplementary Fig. 2E and statistical analysis of AUC. G Cholesterol, high-density lipoprotein (HDL) low-density lipoprotein (LDL), and triglyceride were detected in mice fed with HFD. The results are presented as the mean ± SD (A, B) and the mean ± SEM. C–F, n = 4–10, *P < 0.05, **P < 0.01, by Student's t-test
Loss of Lkb1 in CD11c+ cells specifically restrains the accumulation and CD80’s expression of ATDC in the DIO model
Deletion of the Lkb1 gene in CD11c+ cells is the primary variable between CD11cCreLkb1f/f and Lkb1f/f mice. Therefore, we followed the reported gating strategy combining CD11c and CD64 to determine the effects of Lkb1 on the adipose tissue CD11c+ dendritic cells and macrophages (Fig. S5A) [14]. The results showed that ATDC is the major component among the CD11c+ myeloid cells in Lkb1f/f mice fed with NFD or HFD. In addition, HFD-fed Lkb1f/f mice had a higher percentage and normalized cell number of CD11c−ATM, CD11c+ recruited ATM, and ATDC than NFD-fed Lkb1f/f mice (Fig. 4A, B), which is consistent with the observations in previous DIO studies [10, 14, 34, 39]. Whereas the percentage and number of ATDC rather than CD11c+CD64+ATM in CD11cCreLkb1f/f mice were significantly lower than their Lkb1f/f counterparts (Fig. 4A, B). The similar results was also observed in an age and weight-matched cohort with a slightly lower difference (Figure S2F). However, SAT from CD11cCreLkb1f/f mice has a lower percentage of ATM and ATDC than that from Lkb1f/f mice and has no difference under HFD exposure (Fig. S3A, B). MHC-II is recognized as the mature marker of dendritic cells [40, 41], we thus detected the expression of MHC-II in ATDC. And we observed the percentage of MHC-II+ mature ATDC (mATDC) significantly increased after HFD feeding but there were no differences between these two kinds of genotypic mice in both diet groups (Fig. 4C). Furthermore, we examined the expression of co-stimulatory molecules, CD80 and CD86, on ATDC and ATM (Fig. 4D–G). The results demonstrated that immature ATDC (imATDC) sparely expressed CD80 and CD86 whereas mature ATDC (mATDC) were highly expressed and elevated on HFD exposure. Intriguingly, the upregulation of CD80 in mATDC from CD11cCreLkb1f/f mice was significantly impaired. ATM had higher levels of these two molecules while there is no impaired expression of CD80/86 in ATM from CD11cCreLkb1f/f mice. To validate these observations in ATDC and explore whether these differences are tissue-specific, we utilized another gating strategy (Figs. S4A-C, S5B) and tested splenic DC (Fig. S4D-E). The results further elaborated the above observations in ATDCs and showed that there was no significant difference between splenic DC in terms of cell number and the expression of CD80/CD86. Since the previous reports demonstrated the alteration of IL1A, CCL5, and OX40 in the Lkb1-deficient DC and expression of those genes are also well known to regulate adipose tissue inflammation in obesity [42,43,44,45], we examined the expression of those essential genes on ATM and ATDC. The results showed that ATDC from CD11cCreLkb1f/f has lower levels of CCL5 and OX40 while higher levels of IL1A compared to Lkb1f/f ATDC. The difference in ATM from two genotypic mice is relatively minor in which only OX40 displays a lower expression from CD11cCreLkb1f/f mice (Fig. S1K-M). While the overall levels of IL6 in VAT is comparable between WT and KO mice (Fig. S1J). Therefore, we concluded that HFD feeding favorably induces adipose tissue-specific changes in CD11c+ ATDC, and loss of Lkb1 in these cells curtails the accumulation and the expression of CD80, CCL5, OX40 in ATDC.
Lkb1 deficiency in CD11c+ myeloid cells limited the accumulation and expression of CD80 on ATDC. A, B Representative flow plot and statistical analysis of adipose tissue CD45+ cells from Lkb1f/f (WT) and CD11cCreLkb1f/f (KO) mice after 16 weeks’ NFD or HFD feeding. C Representative overlay histograms of the ATDC and statistical analysis of the composition of ATDC subsets from WT and KO mice after 16 weeks’ NFD or HFD feeding. D, E Representative overlay histograms of the ATDC and ATM subsets and statistical analysis of the MFI of CD80 in indicated groups. F, G Representative overlay histograms of the ATDC and ATM subsets and statistical analysis of the MFI of CD86 in indicated groups. imATDC, immature ATDC; mATDC, mature ATDC. The results are presented as the mean ± SEM, *P < 0.05, **P < 0.01, ***P < 0.001, ****P < 0.0001, by Student's t-test. Data are representative of at least three independent experiments
Lkb1 deficiency in CD11c+ cells specifically reshapes adipose tissue T cell profile upon HFD exposure.
To investigate whether loss of Lkb1 in CD11c+ cells remodels the T cell landscape in AT and its tissue specificity, we examined T cells from the spleen and VAT. As for the spleen, HFD significantly reduced the percentage of CD8+ T cells but not CD4+ T cells. In addition, the percentage of CD4+ T cells from CD11cCreLkb1f/f mice was significantly higher than those from Lkb1f/f mice independent of the type of diet, whereas CD8+ T cells had a lower percentage with no significance (Fig. 5A). Different from the spleen, both VAT CD4+ and CD8+ T cells from CD11cCreLkb1f/f mice were significantly higher than that from Lkb1f/f mice (Fig. 5B, S2G). As for the Treg cell, consistent with our previous study [26], the proportion of splenic and VAT Tregs from CD11cCreLkb1f/f mice was significantly higher than that from Lkb1f/f mice in the NFD group. Unexpectedly, this VAT Tregs advantage in CD11cCreLkb1f/f mice narrowed down on HFD exposure (Fig. 5C, S2G). On the contrary, the proportion of splenic Tregs increased (Fig. 5C). We further detected the expression of the Helios, a good marker of mice thymic Tregs [46], in VAT CD4+ FOXP3+ T cells as you mentioned. The results showed that there is no difference between CD11cCreLkb1f/f and Lkb1f/f mice (Fig. S2I). As for the T cell activation status, we observed that splenic T cells from CD11cCreLkb1f/f mice were markedly activated (CD44hiCD62Llow) compared to those from Lkb1f/f mice with HFD feeding. Meanwhile, VAT had a high level (> 75%) of CD44hiCD62Llow effector T cells, and they were comparable between the two genotypic mice fed with HFD (Fig. 5D, S2H). To determine whether Lkb1 knockout ATDC has depot-specific effects regarding the T cell composition, we further detected the T cell composition in SAT. The results showed that CD8 T cells and Treg cells had a higher percentage in KO mice under NFD and HFD condition, respectively (Fig. S3C-D). Overall, these results indicated that Lkb1 deletion in CD11c+ cells shaped a higher level of the activation status of splenic T cells and T cell-abundant VAT immune microenvironment, and HFD narrowed down the VAT Tregs advantages in CD11cCreLkb1f/f mice.
Loss of Lkb1 in CD11c+ myeloid cells leads to the shape of a T cell-abundant immune profile in the VAT. A, B Representative flow plot and statistical analysis of splenic (A) and VAT (B) T cells from Lkb1f/f (WT) and CD11cCreLkb1f/f (KO) mice with NFD and HFD. C Representative flow plot and statistical analysis of splenic and VAT Tregs from Lkb1f/f and CD11cCreLkb1f/f mice with NFD and HFD. D Representative flow plot and statistical analysis illustrating effector/memory phenotype of splenic and VAT CD8+, Tcon, and Tregs from the two genotypes of mice fed with HFD. The results are presented as the mean ± SD, *P < 0.05, **P < 0.01, ***P < 0.001, ****P < 0.0001, by Student's t-test. Data are representative of at least three independent experiments
Lkb1 deficiency in CD11c+ cells tips the IL-17A and IFN-γ balance towards IFN-γ in various immune cells upon HFD challenge
Except for the immune cells, cytokines as the pivotal factors that affect the AT immune microenvironment and the metabolic outcomes of DIO (Fig. 6A). Therefore, we performed real-time qPCR of total adipose tissue to detect the mRNA levels of several well-recognized cytokines (Fig. 6B, S1J). Compared to Lkb1f/f mice, adipose tissue from CD11cCre Lkb1f/f expressed higher levels of IFNG, whereas IL-17A and LEP, TNFA were significantly lower (Fig. 6B, S1J). Leptin is well defined and mainly derived from adipocytes. Given these indications, we further conducted intracellular cytokine detection of VAT resident immune cells to testify the IFN-γ and IL-17A imbalance at the protein level and figure out their cellular origins (Fig. 6C–E). Consistent with the mRNA observations, we found that IFN-γ and IL-17A imbalance existed in various immune cells. More specifically, myeloid cells including CD11c− ATM, CD11c+ ATM, and ATDC from CD11cCreLkb1f/f mice had significantly lower IL-17A, whereas IFN-γ was higher in these myeloid populations, in which the CD11c−ATM was the most significant (Fig. 6C). As for the CD45+CD11c−CD64− cells, we detected these two cytokines in T cells (Fig. 6D, E). In Lkb1f/f mice, HFD feeding mainly promoted the generation of CD4+T derived IL-17A (Th17 cells) rather than IFN-γ. Surprisingly, CD11cCre Lkb1f/f mice on the opposite, HFD feeding rarely upregulated the generation of Th17 but significantly induced higher levels of IFN-γ. Thus, compared with Lkb1f/f mice, a lower percentage of IL-17A+ and a higher percentage of IFN-γ+ CD4/CD8 T cells from CD11cCre Lkb1f/f mice were detected. The IL-17A and IFN-γ are mainly from CD4 and CD8 T cells, respectively, the corresponding significant differences as well. Taken together, these results collectively suggest that Lkb1 deficiency in CD11c+ cells tips the IL-17A and IFN-γ balance towards IFN-γ in VAT T cells and macrophages upon HFD challenge.
Deficiency of Lkb1 tips IL-17A/IFN-γ towards IFN-γ in both adipose-tissue T cells and ATMs. A Schematic represents major components of the adipose tissue in DIO model. B Real-time qPCR analysis of the indicated cytokine expression in adipose tissue from Lkb1f/f and CD11cCreLkb1f/f mice fed with HFD. C Representative flow plot (left) and statistical analysis (right) of the expression of IL-17A and IFN-γ in CD45+ ATM and ATDC cells from Lkb1f/f and CD11cCreLkb1f/f mice fed with HFD. D Representative flow plot (left) and statistical analysis (right) of the expression of IL-17A in CD4 and CD8 T cells from Lkb1f/f and CD11cCreLkb1f/f mice fed with HFD. E Representative flow plot (left) and statistical analysis (right) of the expression of IFN-γ in CD4 and CD8 T cells from Lkb1f/f and CD11cCreLkb1f/f mice fed with HFD. The results are presented as the mean ± SD, *P < 0.05, **P < 0.01, ***P < 0.001, ****P < 0.0001, by Student's t-test. Data are representative of at least three independent experiments
IFN-γ ex vivo promotes apoptosis of preadipocytes and inhibits their adipogenesis while IL-17A promotes their adipogenesis
To further testify the role of IFN-γ and IL17A in AT, we performed in vitro functional assay of IFN-γ and IL17A on preadipocytes. The results showed no difference in the Ki-67+ cell rate and cellular size between the 50-ng/mL IFN-γ group and the IFN-γ-free group (Fig. S2J, 7A). Compared to the IFN-γ-free control group, the addition of 50-ng/mL IFN-γ in the preadipocytes culture system after 72 h significantly increased the percentage of Annexin V+ apoptosis cells, including the early Annexin V+ PI− and late Annexin V+ PI+ apoptosis cells (Fig. 7B, C). The in vitro differentiation assay of mice preadipocytes showed that the lipid formation was significantly decreased in both 25- and 50-ng/mL IFN-γ group compared to that in the IFN-γ-free group and displayed a dose dependent effect (Fig. 7D). On the contrary, there are more adipocytes differentiated in IL-17A treated group (Fig. 7E). In summary, the ex vivo experiments indicate that IFN-γ promoted apoptosis and inhibited the differentiation of preadipocytes, with no significant effects on their proliferation and cellular size. Meanwhile, IL-17A has a positive effect on the adipogenesis.
In vitro IFN-γ promoted apoptosis and inhibited differentiation of mice preadipocytes but had no significant effect on proliferation and cell size. A Representative overlay histogram (left) and statistical analysis (right) of proliferation index Ki-67 in preadipocytes from Lkb1f/f mice in vitro cultured 72 h with 0 and 50 ng/ml IFN-γ. B Representative flow plot illustrating the apoptosis analysis of preadipocytes from Lkb1f/f mice treated with 0 and 50 ng/ml IFN-γ. C Statistical analysis of early apoptosis, late apoptosis, and total apoptosis of preadipocytes from Lkb1f/f mice treated with 0 and 50 ng/ mL IFN-γ. D, E Representative images and statistical analysis of cell morphology (up) and Oil red “O” staining (bottom) at the end of in-vitro differentiation (Day 14) with indicated conditions of the preadipocytes from Lkb1f/f mice. The results are presented as the mean ± SEM, *P < 0.05, **P < 0.01, ***P < 0.001, ****P < 0.0001, by One-way ANOVA. Data are representative of at least three independent experiments
Discussion
Mounting evidences have elaborated adipose immune status is closely associated with obesity and threatening metabolism disorders [1, 8, 38]. CD11c+ myeloid cells are involved in overnutrition associated immune-metabolic disorders [14, 16, 17, 39]; therefore, understanding of the underlying molecular basis becomes essential in developing intervention strategies for these threatens. Previous studies have revealed the critical role of the nutrient sensor Lkb1 in controlling the function of splenic and lymphoid CD11c+ dendritic cells [26,27,28, 47]. However, the specific role of Lkb1 in fat CD11c+ cells, especially under the high-fat diet exposure, has not been addressed. The present study has elucidated how Lkb1 in fat CD11c+ cells modulate the adipose immune environment and affect the outcomes of diet-induced obesity (DIO).
DIO is the most widely investigated model of type 2 diabetes with hyperglycemia, glucose intolerance, and insulin resistance [8, 10, 16, 39]. There is no significant metabolic dysfunction in CD11cCre Lkb1f/f mice on NFD feeding compared to Lkb1f/f mice. Upon HFD exposure, different from normal mice CD11cCre Lkb1f/f mice had a lower adipose-weight gain and smaller expansion of adipocytes accompanying significantly impaired glucose tolerance. Moreover, according to the metabolic analysis from body weight matched cohort, CD11cCre Lkb1f/f mice still have significant glucose intolerance compared to the Lkb1f/f mice, but the differences narrow down slightly when compared with the random cohort, indicating the body composition partially participates in the metabolic, and also suggesting that there are other potential mechanisms in the downstream of Lkb1 deficiency in CD11c+ cells involved in the regulation of metabolic status in the CD11cCre Lkb1f/f mice.
We observed no significant changes in obesity resistant CD11cCre Lkb1f/f mice regarding the calories intake and rectal temperature. At gene expression level, UCP1, mainly expressed on brown adipose tissue (BAT) and brown-like beige cells [37], upregulates thermogenesis and energy expenditure and therefore protect mice from obesity [48, 49]. The BAT CD11cCre Lkb1f/f mice has 1.44 times higher expression of UCP1 than Lkb1f/f mice under the NFD feeding, which might be associated with the fat gain resistance phenotype of CD11cCre Lkb1f/f mice. Meanwhile, the morphology, expression of UCP1 and oxygen consumption in isolated BAT from DIO model mice are needed to address. Adipose tissue browning is a reversible process that the brown-like beige cells recruit from progenitor cells and transdifferentiate from white adipocytes, which is critical for thermogenic demand and metabolic healthy [50]. The extremely lower expression of UCP1 in CD11cCre Lkb1f/f VAT might indicate that there is a smaller number of beige cells within VAT or lower levels of adipose tissue browning in CD11cCre Lkb1f/f mice. And whether these differences in UCP1 expression ascribe to the obesity resistance and impaired glucose tolerance is warranted to future studies.
Adipose tissue and liver have high risks in steatosis upon HFD exposure [51, 52]. The gained weight of liver varies and the liver/body weight ratio always decreases in different studies with different diet regimens [51,52,53]. Similar with a previous study [53], we did not observe significant liver weight gain and higher liver/body weight ratio in our DIO model. Only the adipose tissue has significantly increased weight among multiple tissues, suggesting the body weight gain of obese mice are mainly from adipose tissue. The increase in hypertrophy (the size) and hyperplasia (the number) of adipocyte causes fat-weight gain and storage the excess calories under HFD exposure [54]. Additionally, it is reported that hypertrophy is strongly correlated with diet and occurs earlier, whereas hyperplasia is more dependent on genetics [55]. Both the CD11cCre Lkb1f/f and Lkb1f/f mice are obesity-prone C57BL/6 (C57) mouse strain, therefore we could exclude the genetic background on the hyperplasia between them. Hypertrophy of adipocyte is basically determined by energy intake and expenditure, which is regulated by adipokines and immune cytokines. The most well-known adipokines are leptin and adiponectin. Obese people and mice has high levels of leptin that inhibits food intake while adiponectin is responsible for energy metabolism [56, 57]. The fat gain resistant CD11cCre Lkb1f/f mice had remarkably lower levels of LEP and comparable levels of ADIPOQ in adipose tissue than Lkb1f/f mice, indicating leptin and adiponectin are not the direct contributors for the body weight decrease in CD11cCre Lkb1f/f mice. Previous studies demonstrated that leptin is required for IL-17A production [58, 59], which might indicate the significant lower secretion of IL-17A in adipose tissue possibly due to the lower expression of LEP from CD11cCre Lkb1f/f mice. Notably, a recent study shows that the inhibition of IL-17A axis through inhibition of RORγt-mediated IL-17A production and selective deletion of IL17RA in adipocytes could dramatically lead to the reduction in adipocyte size, increased white adipose browning and thermogenesis, as well as promote the expression of diabetogenic and obese genes in adipocytes [60]. Besides, IL-17A could accelerate the inflammatory progression in obese mice via the TBK1/IKBKE pathway using IL-17A deficient mice [61]. Moreover, it is also reported that IL-17A directly promotes the cellular uptake of fatty acids [62]. In agreement with these evidences, our study also observed that the most significantly elevated cytokine in Lkb1f/f mice is IL-17A upon HFD exposure, supporting IL-17A is a strong factor for the pathogenesis of DIO. Compared with IL-17A, IFN-γ secretion in T cells is less sensitive in HFD-fed Lkb1f/f mice. However, the IL23-IFNG axis are highly activated in fat tissue from HFD-fed CD11cCre Lkb1f/f mice. And we detected remarkably higher IFN-γ secretion in CD8+ T cells and CD11c− ATM from CD11cCreLkb1f/f mice on HFD challenge. Further tests suggested IFN-γ could promote apoptosis of preadipocytes and inhibit their adipogenesis in vitro, which indicate IFN-γ might inhibit hyperplasia of adipose tissue in vivo. Taken together, the downregulated IL-17A and increased IFN-γ are potentially responsible for the fat gain resistance and smaller adipocytes of CD11cCre Lkb1f/f mice. However, the functional capacity and gene expression including metabolism, survival, and differentiation of preadipocyte and adipocyte in vivo, and other potential contributors in fat gain resistance of CD11cCre Lkb1f/f mice await future studies.
Obesity and hypertrophic adipocytes are associated with dysmetabolism, on the contrary, reduced adipocyte size is usually coupled with body weight loss and improved glucose and lipid metabolism [54]. In the present fat gain resistant CD11cCre Lkb1f/f mice, however, we observed normalized insulin sensitivity after 20 weeks HFD feeding but impaired glucose tolerance after 16 weeks HFD feeding. The initial levels of glucose in IPGTT and IPITT was slightly different which might ascribe to the different time point after HFD feeding and minor difference of blood glucose between two genotypic mice. The impaired glucose tolerance might partially due to the decreased insulin levels. Meanwhile, it might suggest that there are complicating factors involved.
Both IL-17A and IFN-γ are considered proinflammatory contributors in the pathogenesis of metabolic disorders [30, 63, 64]. The dysregulation of IL-17A and IFN-γ in adipose tissue might be one of the critical contributors. Moreover, loss of Lkb1 in CD11c+ cells remarkably increases splenic T cell responses under HFD exposure, which is consistent with other reported evidence on CD11cCre Lkb1f/f mice [26,27,28, 47]. It is reported that activation of autoreactive T cells is one of critical contributors in autoimmune destruction of beta cells [65]. Therefore, additional experiments to explore whether the overactivated peripheral T cells we observed in CD11cCre Lkb1f/f mice affect the function of islet including the insulin secretion are needed. In spite of the pathogenic factors, VAT Tregs play an essentially positive role in adipose homeostasis [18,19,20,21, 23]. In the present study, VAT Tregs in NFD-fed CD11cCreLkb1f/f mice were more abundant than those in Lkb1f/f mice, which indicates Lkb1-deficient CD11c+ cells promote the expansion of VAT Tregs, which is consistent with previous studies [26, 28]. However, this advantage of VAT Tregs sharply decreased upon HFD exposure. Despite that, the overall levels of VAT Tregs in CD11cCreLkb1f/f mice was higher than Lkb1f/f mice during the DIO process. In this respect, VAT Tregs might play a positive role in metabolic outcomes at some extent. As for the origin of these increased VAT Tregs, we observed no difference between CD11cCreLkb1f/f and Lkb1f/f mice, indicating the increased percentage and number of Tregs in VAT is more likely from thymic derived rather than tissue induced Treg cells. According to the splenic and VAT Tregs examination from both NFD and HFD Fig. 5C, the changes of Treg cells in VAT is less dependent on the splenic Tregs, suggesting the VAT Tregs would be likely tissue-specific, otherwise, LKB1 could regulate ATDC’s chemotaxis effects on Tregs during HFD exposure because there is more splenic Tregs when VAT Tregs declined. Our previous work demonstrated that the expanded Treg pool is mediated by the DC-Treg interaction directly [26], which favors the prior assumption. But we do not know exactly whether these increased Treg cells are from VAT Tregs proliferation or migrated from lymphoid tissue or both. Further studies would be needed. Collectively, multiple factors including the imbalance of IL-17A/IFN-γ and decreased expansion of VAT Tregs potentially play a role in the unconventional metabolism outcomes of CD11cCre Lkb1f/f mice.
The factors discussed above are secondary ones, the initial factor is CD11c+ cells because the Lkb1 gene is selectively deleted in CD11c+ cells in CD11cCre Lkb1f/f mice. In addition to adipose tissue dendritic cells (ATDC), CD11c is also expressed on recruited macrophages (ATM). CD64 has been identified as an ideal marker to distinguish ATM from ATDC rather than F4/80 or CD11b and the author demonstrated that ATDCs are independent contributors to adipose tissue inflammation in DIO [14]. In agreement with this study, we found that ATDCs is the dominant CD11c+ cell population in adipose tissue by the high-fat diet regimen induced. Moreover, the Lkb1 expression pattern in ATDC is more sensitive than ATM during fasting. Among the Lkb1f/f mice, ATDC increased in number and the expression of co-stimulation molecules CD80/CD86, and the generation of Th17 was enhanced in HFD fed condition compared to NFD condition, which are consistent with the previous studies [34, 39, 66]. Interestingly, Lkb1 deficiency more specifically affects ATDC than CD11c+ATM regarding the cell number and the expression of CD80, OX40 and CCL5. These evidence might indicate ATDCs are the major contributors to the fat gain resistant phenotype and adipose-tissue immune profile in CD11cCreLkb1f/f mice. The selective effects of Lkb1 on ATDC are possibly due to the striking difference of the functional profiles between ATDC and ATM. ATM is more capable of storing lipid in the setting of obesity than ATDC [14]. Moreover, it is reported that Lkb1 deficiency results in lipid accumulation and aberrant metabolism/mTOR activation in DCs [28]. The overaccumulation of lipid under HFD condition should be further explored. It might make the Lkb1−/− ATDC more fragile and could be a potential mechanism for the reduction in ATDC. Even though we observed more specific changes in ATDC, we cannot exclude the primary contributions of CD11c+ATM on the metabolic and immune phenotypes. Further studies are needed to determine the exact mechanisms underlying Lkb1 on the maintenance of the accumulation and function of ATDCs upon HFD exposure. To determine the role of Lkb1 on ATM, LysmcreLkb1f/f mice would be needed.
The production of IL-17A is enhanced by ATDCs in both mice and humans, which has clear clinical implications in obesity-associated metabolic disorders [39, 66]. Therefore, the decreased number of ATDCs potentially contribute to the downregulated IL-17A in CD11cCreLkb1f/f mice. CD80 and CD86 in DCs provided the second co-stimulatory signals necessary for T cells activation and function. The HFD condition and tissue specific CD80 reduction in dendritic cells is interesting. Two previous studies reported that loss of Lkb1 promotes the maturation of splenic DC with higher levels of CD86 but not CD80 [27, 28], which is similar with our observation in spleen, and the CD80’s stagnation of ATDC under HFD exposure. They proposed that deficiency of Lkb1 leads to lipid accumulation, increased glycolysis, enhanced phospholipase C β1, and activation of both mTORC1 and mTORC2 signaling. These cellular changes in metabolism and signaling release DC from quiescence. But why the expression of CD80 in DC did not increase under activation status is not discussed. In the current HFD induced obesity model, the CD80’s stagnation in Lkb1-/-ATDC becomes more significant might be partially due to the higher lipid accumulation in ATDC, which suppressed the CD80 regulation pathway. Additionally, AMPK and HIF-1α signaling, the canonical pathways of Lkb1 [67, 68], and other potential signaling are warranted to reveal the detailed mechanisms underlying LKB1 selective CD80 regulation in ATDCs under HFD exposure. The expression of these two Ig B7 superfamily member shares the same regulatory underpinnings such as transcription factor PU.1 [69]. Meanwhile, they also had independent regulatory mechanisms. It is reported that decreasing the expression of CD80 /CD86 tips the Th1/Th2 balance towards the Th1 response featuring higher IFN-γ expression [70]. Whether the impaired CD80 expression contributes to the imbalance of IL-17A/IFN-γ secretion await future studies.
It is well recognized that selective deletion of Lkb1 in CD11c+ dendritic cells enhances their capacity to prime effector T cell response and prevailingly expands Treg pools throughout the body [26,27,28, 47]. Therefore, the decreased number of ATDCs possibly contributed to the decreased advantage of VAT Tregs from CD11cCreLkb1f/f mice under HFD exposure. Additionally, elevated production of the adipokine leptin in obese mice can strongly inhibit the proliferation of Tregs by upregulating the cyclin-dependent kinase inhibitor p27 and phosphorylation of ERK1/2 [71]. Our results showed that the LEP mRNA of adipose tissue from CD11cCreLkb1f/f mice was significantly lower than Lkb1f/f mice in DIO. On the other hand, the splenic Treg increased significantly in obese CD11cCreLkb1f/f mice. Thus, the contributors including ATDC, leptin, and recruitment collectively potentially play a role in the decreased advantage of VAT Tregs from fat gain resistant CD11cCreLkb1f/f mice. Future studies are needed to elucidate the specific mechanisms.
There are several limitations of this study. 1. The overall obesity phenotype including liver weight gain and fasting glucose changed in the DIO model is moderate, Lkb1f/f gene modification or other potential reasons should be identified. 2. We did not validate the direct effects of Lkb1−/− ATDC/ATM on adipose T cells, the co-culture experiments to see antigen presentation and cross-presentation function on T cells by AT CD11c+ cells or BMDC from CD11cCreLkb1f/f and Lkb1f/f mice are needed to be confirmed. 3. The concentration of IFN-γ and IL-17A used in in vitro system is much higher than the in vivo levels, therefore, the in vitro data is far equal to physiological status. Meanwhile, purity of the preadipocytes in the system also should be considered. 4. The knock out deficiency and effects of the CD11c+ subsets like CD8+ CD11c+ cells [72] were not investigated and loss of Lkb1 in these cells could also affect the phenotypes we observed. 5. Adipose tissue fibrosis is a classic pathogenically process in DIO model along with inflammatory responses [73], we did not investigate the influences of loss of Lkb1 in CD11c+ cell on this critical process. 6. We observed some phenotypic differences between SAT and VAT but lack the deeper investigations on the Lkb1−/− CD11c+ cells on depot-specific effects.
In summary, this study firstly investigated the role of CD11c+ cells’ Lkb1 in metabolic disease using conditional knockout CD11cCreLkb1f/f mice and DIO model. We demonstrate that Lkb1 is an essential metabolic kinase for ATDCs responding to high-fat exposure. Loss of Lkb1 primarily disturbs the accumulation and CD80’s expression of ATDCs, which potentially leads to the dysregulated balance of IL-17A and IFN-γ and reduced VAT Tregs advantage of CD11cCreLkb1f/f mice in DIO model. These immune factors collectively affect the adipocytes and preadipocytes and ultimately determine the overall fat gain resistant outcome of CD11cCreLkb1f/f mice. Despite adipose tissue specific changes, systemic deletion Lkb1 in CD11c+ cells accompanies T cell over activation. In summary, this study reveals the metabolic sensor, Lkb1, is required for CD11c+ myeloid cells in response to HFD exposure. Meanwhile, it provides new insights into the role of IL-17A and IFN-γ in diet-induced obesity. These findings suggest specific Lkb1 intervention in ATDC might be a potential strategy for the prevention and treatment of diet induced obesity.
Data availability
The datasets used and/or analyzed during the current study are available from the corresponding author on reasonable request.
References
Hotamisligil GS (2006) Inflammation and metabolic disorders. Nature 444:860–867. https://doi.org/10.1038/nature05485
Patton GC, Sawyer SM, Santelli JS, Ross DA, Afifi R, Allen NB, Arora M, Azzopardi P, Baldwin W, Bonell C, Kakuma R, Kennedy E, Mahon J, McGovern T, Mokdad AH, Patel V, Petroni S, Reavley N, Taiwo K, Waldfogel J, Wickremarathne D, Barroso C, Bhutta Z, Fatusi AO, Mattoo A, Diers J, Fang J, Ferguson J, Ssewamala F, Viner RM (2016) Our future: a lancet commission on adolescent health and wellbeing. The Lancet 387:2423–2478. https://doi.org/10.1016/s0140-6736(16)00579-1
Lobstein T, Jackson-Leach R, Moodie ML, Hall KD, Gortmaker SL, Swinburn BA, James WPT, Wang Y, McPherson K (2015) Child and adolescent obesity: part of a bigger picture. The Lancet 385:2510–2520. https://doi.org/10.1016/s0140-6736(14)61746-3
Collaborators GBDO, Afshin A, Forouzanfar MH, Reitsma MB, Sur P, Estep K, Lee A, Marczak L, Mokdad AH, Moradi-Lakeh M, Naghavi M, Salama JS, Vos T, Abate KH, Abbafati C, Ahmed MB, Al-Aly Z, Alkerwi A, Al-Raddadi R, Amare AT, Amberbir A, Amegah AK, Amini E, Amrock SM, Anjana RM, Arnlov J, Asayesh H, Banerjee A, Barac A, Baye E, Bennett DA, Beyene AS, Biadgilign S, Biryukov S, Bjertness E, Boneya DJ, Campos-Nonato I, Carrero JJ, Cecilio P, Cercy K, Ciobanu LG, Cornaby L, Damtew SA, Dandona L, Dandona R, Dharmaratne SD, Duncan BB, Eshrati B, Esteghamati A, Feigin VL, Fernandes JC, Furst T, Gebrehiwot TT, Gold A, Gona PN, Goto A, Habtewold TD, Hadush KT, Hafezi-Nejad N, Hay SI, Horino M, Islami F, Kamal R, Kasaeian A, Katikireddi SV, Kengne AP, Kesavachandran CN, Khader YS, Khang YH, Khubchandani J, Kim D, Kim YJ, Kinfu Y, Kosen S, Ku T, Defo BK, Kumar GA, Larson HJ, Leinsalu M, Liang X, Lim SS, Liu P, Lopez AD, Lozano R, Majeed A, Malekzadeh R, Malta DC, Mazidi M, McAlinden C, McGarvey ST, Mengistu DT, Mensah GA, Mensink GBM, Mezgebe HB, Mirrakhimov EM, Mueller UO, Noubiap JJ, Obermeyer CM, Ogbo FA, Owolabi MO, Patton GC, Pourmalek F, Qorbani M, Rafay A, Rai RK, Ranabhat CL, Reinig N, Safiri S, Salomon JA, Sanabria JR, Santos IS, Sartorius B, Sawhney M, Schmidhuber J, Schutte AE, Schmidt MI, Sepanlou SG, Shamsizadeh M, Sheikhbahaei S, Shin MJ, Shiri R, Shiue I, Roba HS, Silva DAS, Silverberg JI, Singh JA, Stranges S, Swaminathan S, Tabares-Seisdedos R, Tadese F, Tedla BA, Tegegne BS, Terkawi AS, Thakur JS, Tonelli M, Topor-Madry R, Tyrovolas S, Ukwaja KN, Uthman OA, Vaezghasemi M, Vasankari T, Vlassov VV, Vollset SE, Weiderpass E, Werdecker A, Wesana J, Westerman R, Yano Y, Yonemoto N, Yonga G, Zaidi Z, Zenebe ZM, Zipkin B, Murray CJL (2017) Health effects of overweight and obesity in 195 countries over 25 years. N Engl J Med 377:13–27. https://doi.org/10.1056/NEJMoa1614362
Ajuwon KM, Spurlock ME (2005) Adiponectin inhibits LPS-induced NF-κB activation and IL-6 production and increases PPARγ2 expression in adipocytes. Am J Physiol Regul Integr Comp Physiol 288:R1220–R1225. https://doi.org/10.1152/ajpregu.00397.2004
Sun K, Kusminski CM, Scherer PE (2011) Adipose tissue remodeling and obesity. J Clin Invest 121:2094–2101. https://doi.org/10.1172/JCI45887
Greenberg AS, Obin MS (2006) obesity and the role of adipose tisssue in inflammation and metabolism. Am J Clin Nutr 83:461S-465S
Stolarczyk E (2017) Adipose tissue inflammation in obesity: a metabolic or immune response? Curr Opin Pharmacol 37:35–40. https://doi.org/10.1016/j.coph.2017.08.006
Kalathookunnel Antony A, Lian Z, Wu H (2018) T cells in adipose tissue in aging. Front Immunol 9:2945. https://doi.org/10.3389/fimmu.2018.02945
Lee BC, Lee J (2014) Cellular and molecular players in adipose tissue inflammation in the development of obesity-induced insulin resistance. Biochim Biophys Acta 1842:446–462. https://doi.org/10.1016/j.bbadis.2013.05.017
Klein M, Varga I (2018) Microenvironment of immune cells within the visceral adipose tissue Sensu Lato vs. epicardial adipose tissue: What do we know? Inflammation 41:1142–1156. https://doi.org/10.1007/s10753-018-0798-3
Mildner A, Jung S (2014) Development and function of dendritic cell subsets. Immunity 40:642–656. https://doi.org/10.1016/j.immuni.2014.04.016
Macdougall CE, Wood EG, Loschko J, Scagliotti V, Cassidy FC, Robinson ME, Feldhahn N, Castellano L, Voisin MB, Marelli-Berg F, Gaston-Massuet C, Charalambous M, Longhi MP (2018) Visceral adipose tissue immune homeostasis is regulated by the crosstalk between adipocytes and dendritic cell subsets. Cell Metab 27:588–601. https://doi.org/10.1016/j.cmet.2018.02.007. (e4)
Cho KW, Zamarron BF, Muir LA, Singer K, Porsche CE, DelProposto JB, Geletka L, Meyer KA, O’Rourke RW, Lumeng CN (2016) Adipose tissue dendritic cells are independent contributors to obesity-induced inflammation and insulin resistance. J Immunol 197:3650–3661. https://doi.org/10.4049/jimmunol.1600820
Porsche CE, Delproposto JB, Patrick E, Zamarron BF, Lumeng CN (2020) Adipose tissue dendritic cell signals are required to maintain T cell homeostasis and obesity-induced expansion. Mol Cell Endocrinol 505:110740. https://doi.org/10.1016/j.mce.2020.110740
Patsouris D, Li PP, Thapar D, Chapman J, Olefsky JM, Neels JG (2008) Ablation of CD11c-positive cells normalizes insulin sensitivity in obese insulin resistant animals. Cell Metab 8:301–309. https://doi.org/10.1016/j.cmet.2008.08.015
Antony A, Lian Z, Perrard XD, Perrard J, Liu H, Cox AR, Saha P, Hennighausen L, Hartig SM, Ballantyne CM, Wu H (2021) Deficiency of Stat1 in CD11c(+) cells alters adipose tissue inflammation and improves metabolic dysfunctions in mice fed a high-fat diet. Diabetes 70:720–732. https://doi.org/10.2337/db20-0634
Josefowicz SZ, Lu LF, Rudensky AY (2012) Regulatory T cells: mechanisms of differentiation and function. Annu Rev Immunol 30:531–564. https://doi.org/10.1146/annurev.immunol.25.022106.141623
Vasanthakumar A, Chisanga D, Blume J, Gloury R, Britt K, Henstridge DC, Zhan Y, Torres SV, Liene S, Collins N, Cao E, Sidwell T, Li C, Spallanzani RG, Liao Y, Beavis PA, Gebhardt T, Trevaskis N, Nutt SL, Zajac JD, Davey RA, Febbraio MA, Mathis D, Shi W, Kallies A (2020) Sex-specific adipose tissue imprinting of regulatory T cells. Nature 579:581–585. https://doi.org/10.1038/s41586-020-2040-3
Feuerer M, Herrero L, Cipolletta D, Naaz A, Wong J, Nayer A, Lee J, Goldfine AB, Benoist C, Shoelson S, Mathis D (2009) Lean, but not obese, fat is enriched for a unique population of regulatory T cells that affect metabolic parameters. Nat Med 15:930–939. https://doi.org/10.1038/nm.2002
Kolodin D, van Panhuys N, Li C, Magnuson AM, Cipolletta D, Miller CM, Wagers A, Germain RN, Benoist C, Mathis D (2015) Antigen- and cytokine-driven accumulation of regulatory T cells in visceral adipose tissue of lean mice. Cell Metab 21:543–557. https://doi.org/10.1016/j.cmet.2015.03.005
Li C, DiSpirito JR, Zemmour D, Spallanzani RG, Kuswanto W, Benoist C, Mathis D (2018) TCR transgenic mice reveal stepwise, multi-site acquisition of the distinctive fat-Treg phenotype. Cell 174:285–299. https://doi.org/10.1016/j.cell.2018.05.004. (e12)
Fernandes RA, Li C, Wang G, Yang X, Savvides CS, Glassman CR, Dong S, Luxenberg E, Sibener LV, Birnbaum ME, Benoist C, Mathis D, Garcia KC (2020) Discovery of surrogate agonists for visceral fat Treg cells that modulate metabolic indices in vivo. Elife. https://doi.org/10.7554/eLife.58463
Hemminki A, Markie D, Tomlinson I, Avizienyte E, Roth S, Loukola A, Bignell G, Warren W, Aminoff M, Höglund P, Järvinen H, Kristo P, Pelin K, Ridanpää M, Salovaara R, Toro T, Bodmer W, Olschwang S, Olsen AS, Stratton MR, de la Chapelle A, Aaltonen LA (1998) A serine/threonine kinase gene defective in Peutz-Jeghers syndrome. Nature 391:184-187. https://doi.org/10.1038/34432
Wu D, Luo Y, Guo W, Niu Q, Xue T, Yang F, Sun X, Chen S, Liu Y, Liu J, Sun Z, Zhao C, Huang H, Liao F, Han Z, Zhou D, Yang Y, Xu G, Cheng T, Feng X (2017) Lkb1 maintains Treg cell lineage identity. Nat Commun 8:15876. https://doi.org/10.1038/ncomms15876
Chen S, Fang L, Guo W, Zhou Y, Yu G, Li W, Dong K, Liu J, Luo Y, Wang B, Li Z, Zhao C, Sun Z, Shen Y, Leng Q, Zhou D, Han Z, Huang H, Ren H, Xu G, Feng X (2018) Control of Treg cell homeostasis and immune equilibrium by Lkb1 in dendritic cells. Nat Commun 9:5298. https://doi.org/10.1038/s41467-018-07545-8
Pelgrom LR, Patente TA, Sergushichev A, Esaulova E, Otto F, Ozir-Fazalalikhan A, van der Zande HJP, van der Ham AJ, van der Stel S, Artyomov MN, Everts B (2019) LKB1 expressed in dendritic cells governs the development and expansion of thymus-derived regulatory T cells. Cell Res 29:406–419. https://doi.org/10.1038/s41422-019-0161-8
Wang Y, Du X, Wei J, Long L, Tan H, Guy C, Dhungana Y, Qian C, Neale G, Fu YX, Yu J, Peng J, Chi H (2019) LKB1 orchestrates dendritic cell metabolic quiescence and anti-tumor immunity. Cell Res 29:391–405. https://doi.org/10.1038/s41422-019-0157-4
Liu Y, Cheng F, Luo Y, Zhan Z, Hu P, Ren H, Tang H, Peng M (2017) PEGylated curcumin derivative attenuates hepatic steatosis via CREB/PPAR-γ/CD36 pathway. Biomed Res Int 2017:8234507. https://doi.org/10.1155/2017/8234507
Surendar J, Frohberger SJ, Karunakaran I, Schmitt V, Stamminger W, Neumann AL, Wilhelm C, Hoerauf A, Hubner MP (2019) Adiponectin limits IFN-γ and IL-17 producing CD4 T cells in obesity by restraining cell intrinsic glycolysis. Front Immunol 10:2555. https://doi.org/10.3389/fimmu.2019.02555
Al Rijjal D, Wheeler MB (2022) A protocol for studying glucose homeostasis and islet function in mice. STAR Protoc 3:101171. https://doi.org/10.1016/j.xpro.2022.101171
Ariemma F, D’Esposito V, Liguoro D, Oriente F, Cabaro S, Liotti A, Cimmino I, Longo M, Beguinot F, Formisano P, Valentino R (2016) Low-dose bisphenol-a impairs adipogenesis and generates dysfunctional 3T3-L1 adipocytes. PLoS ONE 11:e0150762. https://doi.org/10.1371/journal.pone.0150762
Ajuwon KM, Spurloc ME (2004) Direct regulation of lipolysis by interleukin-15 in primary pig adipocytes. Am J Physiol Regul Integr Comp Physiol 287:R608–R611. https://doi.org/10.1152/ajpregu.00192.2004
Stefanovic-Racic M, Yang X, Turner MS, Mantell BS, Stolz DB, Sumpter TL, Sipula IJ, Dedousis N, Scott DK, Morel PA, Thomson AW, O’Doherty RM (2012) Dendritic cells promote macrophage infiltration and comprise a substantial proportion of obesity-associated increases in CD11c+ cells in adipose tissue and liver. Diabetes 61:2330–2339. https://doi.org/10.2337/db11-1523
Kottakis F, Bardeesy N (2012) LKB1-AMPK axis revisited. Cell Res 22:1617–1620. https://doi.org/10.1038/cr.2012.108
Kouyama R, Suganami T, Nishida J, Tanaka M, Toyoda T, Kiso M, Chiwata T, Miyamoto Y, Yoshimasa Y, Fukamizu A, Horiuchi M, Hirata Y, Ogawa Y (2005) Attenuation of diet-induced weight gain and adiposity through increased energy expenditure in mice lacking angiotensin II type 1a receptor. Endocrinology 146:3481–3489. https://doi.org/10.1210/en.2005-0003
Poher AL, Veyrat-Durebex C, Altirriba J, Montet X, Colin DJ, Caillon A, Lyautey J, Rohner-Jeanrenaud F (2015) Ectopic UCP1 overexpression in white adipose tissue improves insulin sensitivity in lou/C rats, a model of obesity resistance. Diabetes 64:3700–3712. https://doi.org/10.2337/db15-0210
Greenberg AS, Obin MS (2006) Obesity and the role of adipose tissue in inflammation and metabolism. Am J Clin Nutr 83:461S-S465. https://doi.org/10.1093/ajcn/83.2.461S
Bertola A, Ciucci T, Rousseau D, Bourlier V, Duffaut C, Bonnafous S, Blin-Wakkach C, Anty R, Iannelli A, Gugenheim J, Tran A, Bouloumie A, Gual P, Wakkach A (2012) Identification of adipose tissue dendritic cells correlated with obesity-associated insulin-resistance and inducing Th17 responses in mice and patients. Diabetes 61:2238–2247. https://doi.org/10.2337/db11-1274
Shin JS, Ebersold M, Pypaert M, Delamarre L, Hartley A, Mellman I (2006) Surface expression of MHC class II in dendritic cells is controlled by regulated ubiquitination. Nature 444:115–118. https://doi.org/10.1038/nature05261
Adachi Y, Toki J, Ikebukuro K, Tomita M, Kaneda H, Tanabe A, Jun L, Minamino K, Suzuki Y, Taketani S, Ikehara S (2002) Immature dendritic cells (CD11c+ CD3- B220- cells) present in mouse peripheral blood. Immunobiology 206:354–367. https://doi.org/10.1078/0171-2985-00186
Um JY, Rim HK, Kim SJ, Kim HL, Hong SH (2011) Functional polymorphism of IL-1 alpha and its potential role in obesity in humans and mice. PLoS ONE 6:e29524. https://doi.org/10.1371/journal.pone.0029524
Ballak DB, Stienstra R, Tack CJ, Dinarello CA, van Diepen JA (2015) IL-1 family members in the pathogenesis and treatment of metabolic disease: Focus on adipose tissue inflammation and insulin resistance. Cytokine 75:280–290. https://doi.org/10.1016/j.cyto.2015.05.005
Chan PC, Lu CH, Chien HC, Tian YF, Hsieh PS (2022) Adipose tissue-derived CCL5 enhances local pro-inflammatory monocytic MDSCs accumulation and inflammation via CCR5 receptor in high-fat diet-fed mice. Int J Mol Sci. https://doi.org/10.3390/ijms232214226
Liu B, Yu H, Sun G, Sun X, Jin H, Zhang C, Shi W, Tian D, Liu K, Xu H, Li X, Yin J, Hong X, Zhang D (2017) OX40 promotes obesity-induced adipose inflammation and insulin resistance. Cell Mol Life Sci 74:3827–3840. https://doi.org/10.1007/s00018-017-2552-7
Thornton AM, Korty PE, Tran DQ, Wohlfert EA, Murray PE, Belkaid Y, Shevach EM (2010) Expression of Helios, an Ikaros transcription factor family member, differentiates thymic-derived from peripherally induced Foxp3+ T regulatory cells. J Immunol 184:3433–3441. https://doi.org/10.4049/jimmunol.0904028
Wculek SK, Sancho D (2019) LKB1 restrains dendritic cell function. Cell Res 29:429–431. https://doi.org/10.1038/s41422-019-0169-0
Feldmann HM, Golozoubova V, Cannon B, Nedergaard J (2009) UCP1 ablation induces obesity and abolishes diet-induced thermogenesis in mice exempt from thermal stress by living at thermoneutrality. Cell Metab 9:203–209. https://doi.org/10.1016/j.cmet.2008.12.014
Li B, Nolte LA, Ju JS, Han DH, Coleman T, Holloszy JO, Semenkovich CF (2000) Skeletal muscle respiratory uncoupling prevents diet-induced obesity and insulin resistance in mice. Nat Med 6:1115–1120. https://doi.org/10.1038/80450
Bartelt A, Heeren J (2014) Adipose tissue browning and metabolic health. Nat Rev Endocrinol 10:24–36. https://doi.org/10.1038/nrendo.2013.204
Pilling D, Karhadkar TR, Gomer RH (2021) High-fat diet-induced adipose tissue and liver inflammation and steatosis in mice are reduced by inhibiting sialidases. Am J Pathol 191:131–143. https://doi.org/10.1016/j.ajpath.2020.09.011
Liu C, Rajapakse AG, Riedo E, Fellay B, Bernhard MC, Montani JP, Yang Z, Ming XF (2016) Targeting arginase-II protects mice from high-fat-diet-induced hepatic steatosis through suppression of macrophage inflammation. Sci Rep 6:20405. https://doi.org/10.1038/srep20405
Zhang J, Powell CA, Kay MK, Sonkar R, Meruvu S, Choudhury M (2021) Effect of chronic western diets on non-alcoholic fatty liver of male mice modifying the PPAR-γ pathway via miR-27b-5p regulation. Int J Mol Sci. https://doi.org/10.3390/ijms22041822
Stenkula KG, Erlanson-Albertsson C (2018) Adipose cell size: importance in health and disease. Am J Physiol Regul Integr Comp Physiol 315:R284–R295. https://doi.org/10.1152/ajpregu.00257.2017
Jo J, Gavrilova O, Pack S, Jou W, Mullen S, Sumner AE, Cushman SW, Periwal V (2009) Hypertrophy and/or hyperplasia: dynamics of adipose tissue growth. PLoS Comput Biol 5:e1000324. https://doi.org/10.1371/journal.pcbi.1000324
Kern PA, Di Gregorio GB, Lu T, Rassouli N, Ranganathan G (2003) Adiponectin expression from human adipose tissue: relation to obesity, insulin resistance, and tumor necrosis factor-alpha expression. Diabetes 52:1779–1785. https://doi.org/10.2337/diabetes.52.7.1779
Zhang Y, Proenca R, Maffei M, Barone M, Leopold L, Friedman JM (1994) Positional cloning of the mouse obese gene and its human homologue. Nature 372:425–432. https://doi.org/10.1038/372425a0
Yu Y, Liu Y, Shi FD, Zou H, Matarese G, La Cava A (2013) Cutting edge: leptin-induced RORgammat expression in CD4+ T cells promotes Th17 responses in systemic lupus erythematosus. J Immunol 190:3054–3058. https://doi.org/10.4049/jimmunol.1203275
Reis BS, Lee K, Fanok MH, Mascaraque C, Amoury M, Cohn LB, Rogoz A, Dallner OS, Moraes-Vieira PM, Domingos AI, Mucida D (2015) Leptin receptor signaling in T cells is required for Th17 differentiation. J Immunol 194:5253–5260. https://doi.org/10.4049/jimmunol.1402996
Teijeiro A, Garrido A, Ferre A, Perna C, Djouder N (2021) Inhibition of the IL-17A axis in adipocytes suppresses diet-induced obesity and metabolic disorders in mice. Nat Metab 3:496–512. https://doi.org/10.1038/s42255-021-00371-1
Lee SH, Jhun J, Byun JK, Kim EK, Jung K, Lee JE, Choi JY, Park SH, Cho ML (2017) IL-17 axis accelerates the inflammatory progression of obese in mice via TBK1 and IKBKE pathway. Immunol Lett 184:67–75. https://doi.org/10.1016/j.imlet.2017.02.004
Yu C, Niu X, Du Y, Chen Y, Liu X, Xu L, Iwakura Y, Ma X, Li Y, Yao Z, Deng W (2020) IL-17A promotes fatty acid uptake through the IL-17A/IL-17RA/p-STAT3/FABP4 axis to fuel ovarian cancer growth in an adipocyte-rich microenvironment. Cancer Immunol Immunother 69:115–126. https://doi.org/10.1007/s00262-019-02445-2
Veikko AKOIVISTORP, KARI CANTELL. (1989) Effect of Interferon on Glucose Tolerance and Insulin Sensitivity insulin resistance and hyperinsulinaemia in patients with chronic. Diabetes 38:641–647
Eiichi Imano TK, Ishigami Y, Kubota M, Ikeda M, Matsuhisa M, Kawamori R, Yamasaki Y (1998) Interferon induces insulin resistance in patients with chronic active hepatitis C. J Hepatol 28:189–193
Burrack AL, Martinov T, Fife BT (2017) T cell-mediated beta cell destruction: autoimmunity and alloimmunity in the context of type 1 diabetes. Front Endocrinol (Lausanne) 8:343. https://doi.org/10.3389/fendo.2017.00343
Chen Y, Tian J, Tian X, Tang X, Rui K, Tong J, Lu L, Xu H, Wang S (2014) Adipose tissue dendritic cells enhances inflammation by prompting the generation of Th17 cells. PLoS ONE 9:e92450. https://doi.org/10.1371/journal.pone.0092450
Shackelford DB, Shaw RJ (2009) The LKB1-AMPK pathway: metabolism and growth control in tumour suppression. Nat Rev Cancer 9:563–575. https://doi.org/10.1038/nrc2676
Faubert B, Vincent EE, Griss T, Samborska B, Izreig S, Svensson RU, Mamer OA, Avizonis D, Shackelford DB, Shaw RJ, Jones RG (2014) Loss of the tumor suppressor LKB1 promotes metabolic reprogramming of cancer cells via HIF-1alpha. Proc Natl Acad Sci U S A 111:2554–2559. https://doi.org/10.1073/pnas.1312570111
Kanada S, Nishiyama C, Nakano N, Suzuki R, Maeda K, Hara M, Kitamura N, Ogawa H, Okumura K (2011) Critical role of transcription factor PU.1 in the expression of CD80 and CD86 on dendritic cells. Blood 117:2211–2222. https://doi.org/10.1182/blood-2010-06-291898
Li JG, Du YM, Yan ZD, Yan J, Zhuansun YX, Chen R, Zhang W, Feng SL, Ran PX (2016) CD80 and CD86 knockdown in dendritic cells regulates Th1/Th2 cytokine production in asthmatic mice. Exp Ther Med 11:878–884. https://doi.org/10.3892/etm.2016.2989
De Rosa V, Procaccini C, Cali G, Pirozzi G, Fontana S, Zappacosta S, La Cava A, Matarese G (2007) A key role of leptin in the control of regulatory T cell proliferation. Immunity 26:241–255. https://doi.org/10.1016/j.immuni.2007.01.011
Vinay DS, Kwon BS (2010) CD11c+CD8+ T cells: two-faced adaptive immune regulators. Cell Immunol 264:18–22. https://doi.org/10.1016/j.cellimm.2010.05.010
Pessin JE, Kwon H (2012) How does high-fat diet induce adipose tissue fibrosis? J Investig Med 60:1147–1150. https://doi.org/10.2310/JIM.0b013e318271fdb9
Acknowledgements
This work was supported by the Non-profit Central Research Institute Fund of Chinese Academy of Medical Sciences (2019-RC-HL-013), the National Natural Science Foundation of China (81870090, 81760035), and the National Key R&D Program of China (2021YFA1100703).
Author information
Authors and Affiliations
Contributions
XF, MS, and YL designed the study. YS performed most of the experiments and acquired data. BW performed the qPCR test and gave supports in flow, ICS, and tissue immune cell isolation. QH participated in mouse breeding, DIO establishment, and performed some experiments. YS, BW, and YL analyzed the data. XL, CZ, TW, JW, XZ, QN, and BH helped perform some experiments. QH, HZ completed most of the revision experiments. BW, YS, EJ, YL, and XF discussed and wrote the manuscript. XF and YL supervised the project.
Corresponding authors
Ethics declarations
Conflict of interest
The authors declare no conflict of interests.
Additional information
Publisher's Note
Springer Nature remains neutral with regard to jurisdictional claims in published maps and institutional affiliations.
Supplementary Information
Below is the link to the electronic supplementary material.
Supplemental Fig. 1. (A-B)
mRNA quantitation of LKB1 (A) and AMPKA (B) of adipose tissue CD11c+ cells among fasting, fed, NFD, and HFD conditions. (C) The expression of LKB1 in the adipose tissue and splenic CD11c+ subsets. (D) The expression of LKB1 in splenic CD11c+ subsets from WT and KO mice. (E) Daily monitoring data of accumulated food intake in Lkb1f/f and CD11cCre Lkb1f/f mice fed with HFD (5.24 kcal/g). (F) Monitoring data of rectal temperature in Lkb1f/f and CD11cCre Lkb1f/f mice in DIO model. (G) The UCP1 expression of VAT and BAT tissue from Lkb1f/f mice fed with NFD or HFD. (H) Histological analysis of liver from Lkb1f/f and CD11cCre Lkb1f/f mice in DIO model. The red arrow represents the infiltrated immune cells; the black arrow represents the hepatic steatosis; the yellow arrow represents ballooning degeneration of hepatocytes. (I) Statistical analysis of the liver versus body weight ratio in the indicated groups. (J) The expression of UCP1, TNFA, IL6 in adipose tissue from WT and KO mice. (I) The expression of IL1A, CCL5, OX40 in ATM and ATDC from WT and KO mice. The gating strategy of DC and macrophage are based on the expression of CD64 and CD11c as shown in Supplementary Fig. 5. The cells in A-C were sorted from Lkb1f/f mice. WT and KO represent Lkb1f/f and CD11cCre Lkb1f/f mice, respectively. The results are presented as the mean ± SEM, *P < 0.05, **P < 0.01, ***P < 0.001, ****P < 0.0001, by Student's t-test. Data are representative of at least three independent experiments. Supplemental Fig. 2. (A) Intraperitoneal glucose tolerance test (IPGTT) of mice after 16 weeks of NFD feeding. And statistical analysis of the area under the curve (AUC) of the IPGTT from Lkb1f/f and CD11cCreLkb1f/f mice. (B) Intraperitoneal insulin tolerance test (IPITT) of mice after 16 weeks of NFD feeding. (C-F) Metabolic and immune analysis of the age and body weight matched cohort from Lkb1f/f and CD11cCreLkb1f/f mice with 16 weeks of HFD feeding. (C) Body weight statistical analysis. (D) Fasting glucose. (E) IPGTT tests and corresponding statistical analysis of the area under the curve (AUC) of the IPGTT. (F) Flow dot plots of ATDC and ATM among CD45+ cells. And statistical analysis of the composition of ATDC and ATM. (G) Statistical analysis of the absolute number of CD4, CD8, and Treg cells in adipose tissue corresponding to Fig. 5B-C. (H) Statistical analysis of absolute number of activated CD4, CD8, and Treg cells in adipose tissue corresponding to Fig.5D. (I) Flow dot plot with smooth model represents the expression of Helios in Foxp3+ VAT Tregs from WT and KO in DIO model. (J) Representative overlay histogram (left) and statistical analysis (right) of preadipocytes size in vitro cultured 72 hours with 0 and 50 ng/ml IFN-γ. The comparison of preadipocytes’ size was based on the FSC-A value. The results are presented as the mean ± SEM, *P < 0.05, **P < 0.01, ***P < 0.001, ****P < 0.0001, by Student's t-test. Data are representative of at least three independent experiments. Supplemental Fig. 3. (A-B) Representative flow plot and statistical analysis of SAT ATM and ATDC cells from Lkb1f/f and CD11cCreLkb1f/f mice with NFD and HFD (A) and (B) corresponding statistical analysis. (C-D) Representative flow plot and statistical analysis of SAT T cell subsets and their effector/memory marker expression (C) and (D) Statistical analysis the composition of SAT T cells and effector/memory subsets. The results are presented as the mean ± SEM, *P < 0.05, **P < 0.01, ***P < 0.001, ****P < 0.0001, by Student's t-test. Data are representative of at least three independent experiments. Supplemental Fig. 4. Loss of Lkb1 in CD11c+ cells limits the accumulation and expression of CD80’s of ATDC but not splenic DCs with classic gating analysis. (A) Representative flow plot and statistical analysis of the CD45+MHC-II+CD11Chi cells in VAT. (B) Representative histogram and statistical analysis illustrating CD64+ ATMs and CD64- ATDCs from Lkb1f/f and CD11cCreLkb1f/f mice with NFD and HFD. (C) Representative overlay histogram and statistical analysis of the expression of CD80 and CD86 in CD64-ATDCs from Lkb1f/f and CD11cCreLkb1f/f mice with NFD and HFD. (D) Representative flow plot and statistical analysis of CD45+MHC-II+CD11chi splenic dendritic cells. (E) Representative overlay histogram and statistical analysis of the expression of CD80 and CD86 in splenic DCs from Lkb1f/f and CD11cCreLkb1f/f mice with NFD and HFD. The results are presented as the mean ± SEM, *P < 0.05, **P < 0.01, ***P < 0.001, ****P < 0.0001, by Student's t-test. Data are representative of at least three independent experiments. Supplemental Fig. 5. Gating strategies. (A) The gating strategy of CD45+ myeloid subsets used in Figure 4 and Figure 6 C. (B) The gating strategy of classic DC and ATM used in Supplementary Figure 1. (C) The gating strategy of T cells used in Figure 5 and Figure 6 D-E. (D) The gating strategy of preadipocytes used in Figure 7. (PDF 629 KB)
Rights and permissions
Open Access This article is licensed under a Creative Commons Attribution 4.0 International License, which permits use, sharing, adaptation, distribution and reproduction in any medium or format, as long as you give appropriate credit to the original author(s) and the source, provide a link to the Creative Commons licence, and indicate if changes were made. The images or other third party material in this article are included in the article's Creative Commons licence, unless indicated otherwise in a credit line to the material. If material is not included in the article's Creative Commons licence and your intended use is not permitted by statutory regulation or exceeds the permitted use, you will need to obtain permission directly from the copyright holder. To view a copy of this licence, visit http://creativecommons.org/licenses/by/4.0/.
About this article
Cite this article
Sun, Y., Wang, B., Hu, Q. et al. Loss of Lkb1 in CD11c+ myeloid cells protects mice from diet-induced obesity while enhancing glucose intolerance and IL-17/IFN-γ imbalance. Cell. Mol. Life Sci. 80, 63 (2023). https://doi.org/10.1007/s00018-023-04707-w
Received:
Revised:
Accepted:
Published:
DOI: https://doi.org/10.1007/s00018-023-04707-w