Abstract
Landslides govern the evolution of landforms and pose a serious threat across the globe, especially in mountainous areas. In the northwestern area of Vietnam, a deep-seated landslide occurred near the new Mong Sen bridge in Trung Chai commune, Sapa town, Laocai province. In October 2020, some serious cracks were observed above a natural slope near a road, which was under construction to connect the Noi Bai-Laocai highway to Sapa town. The crack area was divided into two zones. Since this phenomenon could not only cause loss of life but also damage to the properties located downhill, the construction was stopped until the slope was rehabilitated. In this study, geological investigations, laboratory tests, and stability analysis were conducted to understand the failure mechanism. The analysis results showed that cutting activities during the construction of the road contributed to the sliding of the sloping soil mass. The following rehabilitation works at the failed area were conducted: (1) removing the soil above the natural slope; and (2) constructing the retaining system, including ground anchors and soil nails to retain the remaining slope. Results of stability analysis demonstrated that the retained slope would be stable after treatment.
You have full access to this open access chapter, Download chapter PDF
Similar content being viewed by others
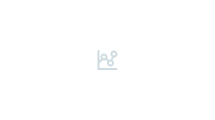
Keywords
1 Introduction
Landslides are one of the most prominent geo-hazards experienced by many countries around the world. They are more predominant in coastal and mountainous regions. Landslides can be caused due to various geological and environmental factors, and endanger the safety of human beings, properties, and infrastructure. Besides the effects of heavy and/or long rainfall events, landslides may occur due to human activities, such as cutting and filling the slope during the course of construction projects on slopes. These activities have not only affected the living environment but also increased the number of landslides. In Vietnam, many landslides have been reported in previous studies (Nguyen et al. 2020; Luong et al. 2017; Tien et al. 2016; Duc 2013).
Laocai is a mountainous province, located between the Northeast and Northwest regions of Vietnam. Previously, the old Mong Sen bridge landslide occurred many times in 1990, 1994, 1996, 1998, 2000, 2001, and 6/2002 due to heavy rainfall and geological complex condition in this area. With the increasing demand for transportation on National Highway 4D from Laocai City to Sapa Town, the Laocai-Sapa expressway was constructed in 2018. However, the section from Km12+720 to Km12+860 of the new expressway is located in an area with complex topography, geomorphology, and geological conditions. In 2020, two deep-seated landslides happened during the construction of excavated slope in this section. The landslides mass needs to be handled to ensure the safety of the area.
In this study, the failure mechanism of a new Mong Sen landslide was examined based on comprehensive laboratory testing and back analysis data. The effectiveness of countermeasures was then evaluated through stability analysis using the finite element method with shear strength reduction. This paper presents these findings along with recommended practices for field implementation to mitigate landslides.
2 Topography and Geologic Setting
The new Mong Sen landslide occurred in the Trung Chai commune, Sapa town, Laocai province, Vietnam. It is located at 22° 25′ 1.68″ N and 103° 54′ 18.03″ E, approximately 0.6 km far from the new Mong Sen bridge (Fig. 1). Different formations and features located in the region can be observed in Fig. 1. The slope related to the landslide is a part of the Lo Suay Tong Mountain in the Hoang Lien Son range. This region is generally aligned in the northwest-southeast direction and covered by lush green vegetation. Its altitude varies from 1300 to 1700 m above sea level with many slopes having a horizontal angle greater than 25°. This area is based on Posen formation (dgPZ1ps) with intrusive rocks including diorite, granodiorite, and granite. The Posen formation was observed in many landslide events in Laocai province (Chu and Nguyen 2008; Nguyen et al. 2021). The angle of slope in this area was about 25° and the soil/rock layer was weathered crust.
3 Climate and Rainfall
During the winter season, the weather is foggy and cold with occasional snowfall (Leisz 2017). The rainy season begins from May to October and accounts for 80% of the total annual rainfall. The average annual rainfall is about 1500 mm per year with a maximum rainfall of more than 3500 mm. The least rainfall months have an average rainfall from 50 to 100 mm/month. Hail sometimes occurs in February, March, and April. The monthly rainfall during 2018–2020 can be seen in Fig. 2, according to the recorded data from the Sapa Meteorological station (http://dulieu.phongchongthientai.vn/vi/RealTime2/Data#). The cumulative rainfall in 2020 was 3000 mm.
4 Landslide Characteristics
The new Mong Sen landslide occurred in Trung Chai commune, Sapa town, Laocai province. In this area, a new road is under construction, connecting the Noi Bai-Laocai highway to Sapa town. If the road was finished, it would enhance the tourism of Sapa town. However, since the construction included cutting slopes, many landslides have occurred. Among them, the landslide near the new Mong Sen bridge on October 27th, 2020 was the biggest one. Figure 3 shows the morphological changes of the slope during the course of construction from October 30th, 2019 to October 27th, 2020. The landslide area included 2 zones, of which zone 1 was about 2.5 ha and zone 2 was about 0.5 ha. As shown in Fig. 3a, the slope was originally covered by lush green vegetation. Its appearance changed significantly during the road construction with lack of vegetation. Zones 1 and 2 were differentiated by the range of cracks, as marked in Fig. 3b. Particularly, in zone 1, the local road was broken with a 0.4 m-wide crack (Fig. 3c). There were many cracks along the head scarf with an average subsidence of 0.5 m (Fig. 3d, e). On the other hand, in zone 2, different head scarfs were observed, which indicated that the landslide took place in many stages (Fig. 3f).
Morphological changes of the slope by time and landslide views: a before road construction on October 30th, 2019; b during road construction on October 27th, 2020; c crack on local road 155; d crack on head scarf at zone 1; e crack along slide body at zone 1, and f head scarfs at different stages at zone 2
After the site investigation on October 27th, 2020, observation stations were installed to monitor the ground surface displacement. As shown in Fig. 4, 30 stations from QT1 to QT30 were distributed in the main sliding zone only (zone 1). During the construction of a retaining system, ground surface displacement developed much along the head scarf with a maximum value of 0.4 m. This increase in ground surface displacement was partially attributed to the dynamic load of the drilling machine when installing ground anchors and soil nails. The layout of the cracks occurring in zones 1 and 2 was sketched in Fig. 5, in which the overall failure of the slope could be seen more clearly.
5 Geological Drilling and Soil Properties
Figure 5 shows the layout of boreholes and cross-sections performed in the geological investigation. There were 9 boreholes, among which 6 boreholes were distributed in zone 1 and 3 boreholes were in zone 2. Based on them, the cross-sections 1-1 and 2-2 of the slope were created for zone 1 while cross-section 3-3 was built for zone 2. Standard penetration tests (SPTs) were carried out at each of the boreholes and soil samples were taken to perform laboratory tests.
Figure 6 shows three cross-sections in the landslide area. As shown in the figure, there are ten layers within the stratigraphy. The 1st layer is yellow/brown medium sandy clay mixed with 40% boulder (ML). Its thickness changes from 5.0 to 15 m with uncorrected Nspt = 15–50. The 2nd layer is yellow/brown medium to hard sandy clay mixed with 40% boulder. This layer is located only at the cross-section 1-1 with the boreholes LKBS1, LKBS2, and LKBS3. Its thickness varies from 1 to 3 m with uncorrected Nspt = 21–30. The 3rd layer is medium sandy clay mixed with 40% boulders (ML). This layer is distributed only at the cross-section 1-1, which is the same as the 2nd layer. Its average thickness is 5 m with uncorrected Nspt = 5–14. The 4th layer is yellow/brown medium sandy clay mixed with gravel (ML). This layer is found at the cross-section 3-3 with the boreholes LKBS7, LKBS8, and LKBS9. Its thickness varies from 4 to 10 m with uncorrected Nspt = 12–20. The 5th layer is yellow/dark medium to hard sandy clay mixed with gravel (ML). This layer is distributed across the site with the thickness from 5 to 15 m and uncorrected SPT value, Nspt = 20–50. The 6th layer is gray very hard gravel. Its thickness changes from 2 to 7 m with uncorrected Nspt = 50. The 7th layer is gray hard sandy clay mixed with gravel (ML). This layer is about 15 m thick with uncorrected Nspt = 30–50. The 8th layer is gray very hard sandy clay mixed with gravel (ML). Its thickness is approximately 10 m with uncorrected Nspt = 50. The 6th, 7th, and 8th layers can be found only in the cross-section 2-2. The 9th layer is gray strongly weathered rock. This layer has a total core recovery (TCR) = 40–50% and rock mass quality (RQD) = 30–40%. Its thickness varies from 3 to 10 m. The 10th layer is gray weathered rock. This layer has TCR = 60–70% and RQD = 50–60%. Its thickness is very large. The rock layers are found at the depth of 10 m, 30 m, and 15 m corresponding to the cross-sections 1-1, 2-2, and 3-3, respectively. Due to the existence of thick topsoil, the slope in this area is prone to instability during road construction.
The grain-size curves of the 1st, 3rd, 4th, 5th, 7th, and 8th layers are plotted in Fig. 7. Their data were collected from both sieving and hydrometer tests. It can be observed that at each of the soil layers, the curves are consistent among the boreholes. Soil properties determined from laboratory tests are summarized in Table 1. The friction angle and cohesion of soil were determined from direct shear tests for both natural and saturated conditions. For the 9th and 10th layers, testing of compressive strength of weathered rock samples was conducted. The result showed that the average compressive strengths of saturated rock samples for the layer 9th and 10th layers were 26.27 MPa and 53.33 MPa, respectively.
6 Countermeasures and Stability Analysis
Based on the results of the site investigation and tests, countermeasures have been proposed to retain the sliding slope. Firstly, the very thick topsoil of the slope was partially removed, from 5 to 10 m, in order to reduce the soil weight and widen the new road to the designed width. Secondly, the ground anchor system was installed with length from 15 to 30 m. Each of the anchors had a 10 m bond length, which was located mostly in the rock layers. Also, it was composed of 4 prestressed strands of 12.7 mm type. The average anchor spacing was 3 m. A reinforced concrete beam network was employed to evenly distribute the anchor load to the ground surface. The beam cross-section was 0.3 m × 0.3 m. Thirdly, the soil nail system was mobilized to retain the topsoil against shallow failure. Each of the soil nails consisted of a 32 mm steel bar, which was installed in 91 mm drilling holes and fixed by cement grout. The soil nail length was 11.7 m and the spacing was 3 m. Fourthly, the piping system was applied to lower the groundwater table. Each pipe was 75 mm in diameter and from 10 to 30 m in length. Finally, runoff water was collected using the shotcrete on the ground surface and the ditch system.
Stability analysis was performed using Plaxis 2D. This software is capable of modeling complicated stratigraphy and soil behaviors. Stability analysis will be performed using the finite element method with reduced shear strength. In principle, both input parameters, c, and tanϕ of soil were reduced from the original ones by dividing the Msf coefficient (Nie et al. 2019; Tschuchnigg et al. 2015; Plaxis 2020). During stability analysis, the Msf coefficient is gradually increased, which leads to the continuous reduction of the input c and tanϕ values. The analyzed slope will be weakened until the numerical solution cannot converge. This time is defined as the failure of the slope and the corresponding Msf coefficient is treated as the factor of safety of the slope. The input parameters of soil and rock layers used in the analysis are summarized in Table 2. In this analysis, behaviors of both soil and weathered rock were assumed to be elastic perfectly plastic based on the Mohr–Coulomb model. This soil model purely requires five parameters. They are Young’s modulus (E), Poisson’s ratio (ν), friction angle (ϕ), cohesion (c), and dilatancy angle (ψ).
Figure 8a is the incremental displacement plot at the failure of the slope in zone 2 (the cross-section 3-3) before the application of the retaining system. The influence of the road construction is considered by removing a part of the soil at the slope toe. In essence, the incremental displacement exhibits the development of the nodal displacements during stability analysis when the Msf coefficient is increased or the soil is weakened. Therefore, the failure surface of the slope can be determined based on the change of shadings in this plot. As marked in Fig. 8a, the predicted failure surface is consistent with the observed range of cracks at the site so that the result is reasonable. In addition, the maximum Msf coefficient or the factor of safety in the analysis is 0.93, which is in good agreement with the instability of the slope. After the application of the retaining system, as shown in Fig. 8b, the predicted failure surface goes through the anchor system. The corresponding safety factor is 1.27, which is greater than the required value of 1.25 in the Vietnamese standard (22TCN 171-87) and demonstrates the stability of the slope after treatment. Note that the influence of the anchor system is modeled through the structural elements and prestressed forces. Since the results of cross-sections 1-1 and 2-2 are similar to those of cross-section 3-3 as described above, they are not mentioned in this section.
7 Discussion
Based on the distribution of cracks throughout zones 1 and 2 (Figs. 3 and 5), the deep-seated failure mechanism has been identified in both zones. It is characterized by the curved cracks on the ground surface observed in Fig. 3b and the subsidence of the ground surface near the head scarf in Fig. 3d, f. Particularly, in the zone 2, many head scarfs could be seen in Fig. 3f, which demonstrates the occurrence of many failures at different stages during the course of road construction. Also, the deep-seated failure of the slope in the zone 2 might be inferred from the shape of the failure surface predicted by the stability analysis in Fig. 8a, which is deep and extends throughout the slope.
The results of the site investigation and back analysis show that the construction of the new road was a major triggering factor of the landslide. During the road construction, part of the soil located at the slope toe at both of the zones 1 and 2 was removed, which led to the instability of the slopes. Besides, the construction site locates at near the new Mong Sen bridge, at which many landslides have been recorded (Nguyen and Dao 2006). The site has a very thick topsoil, which is composed of clay, sand, gravel, and small rock. This topsoil is easily eroded and reduces shear strength during rainfall. In addition, since the inclination angle of the rocks layers (the 9th and 10th layers) is rather high (from 15° to 45° in Fig. 6), the geological and morphological features also play a role in the occurrence of the slope failure in the area.
8 Conclusions
This paper presented a study on a landslide that occurred near the new Mong Sen bridge in Trung Chai commune, Sapa town, Laocai province. The sliding area could be divided into zones 1 and 2. Based on the results of the study, some conclusions can be obtained as follows:
-
(i)
The landslide mechanism belongs to a deep-seated type, which is characterized by the curved cracks along the failure surface on the ground and the subsidence of the ground surface near the head scarf. In particular, in zone 2, many head scarfs corresponding to the different failures were observed during the road construction. The failure surface predicted by the stability analysis also agrees with the observed data.
-
(ii)
The construction of the new road was the main trigger of the landslide, which removed part of the soil at the slope toe. Besides, the complicated geological conditions, including the thick topsoil and the high inclination angle of bedrock (from 15° to 45°), were also triggering factors for the landslide.
-
(iii)
The countermeasures, which were applied to retain the landslide mass, were performed with the following steps: (1) removed part of the soil on the slope to reduce soil weight; (2) installed ground anchors and soil nails to retain the remaining slope; (3) lowered groundwater table using long pipes; and (4) gathered runoff water using the shotcrete and ditch system on the ground surface. The effectiveness of the countermeasures has been validated through the results of stability analysis, in which the factor of safety of the slope was greater than the required value.
References
22TCN 171-87 Soil investigation procedure and design of stabilizing method for road embankment in area vulnerable to landslide (in Vietnamese)
Chu VN, Nguyen TTH (2008) Assess the risk of landslides along the 4D route based on studying the relationship between geological structure and topography (in Vietnamese). http://idm.gov.vn/nguon_luc/Xuat_ban/2008/A305/a1.htm
Duc DM (2013) Rainfall-triggered large landslides on 15 December 2005 in Van Canh District, Binh Dinh province, Vietnam. Landslides 10:219–230. https://doi.org/10.1007/s10346-012-0362-4
Leisz SJ (2017) Land-cover and land-use transitions in northern Vietnam from the early 1990s to 2012 BT. Redefining diversity and dynamics of natural resources management in Asia, chap 6, vol 2, pp 77–86. http://doi.org/10.1016/B978-0-12-805453-6.00006-1
Luong LH, Miyagi T, Tien PV, Loi DH, Hamasaki E, Abe S (2017) Landslide risk evaluation in central provinces of Vietnam. In: Advancing culture of living with landslides, pp 1145–1153. https://doi.org/10.1007/978-3-319-53498-5
Nguyen VC, Dao VT (2006) Investigation and research of landslide geohazard in north-western part of Vietnam for the sustainable development of the territory. The Core University Program between Japan Society for the Promotion of Science (JSPS) and Vietnamese Academy of Science and Technology (VAST). Annual report of FY 2006, pp 269–280. http://hdl.handle.net/11094/13027
Nguyen LC, Tien PV, Do TN (2020) Deep-seated rainfall-induced landslides on a new expressway: a case study in Vietnam. Landslides 17:395–407. https://doi.org/10.1007/s10346-019-01293-6
Nguyen KT, Miyagi T, Isurugi S, Dinh VT (2021) Developing recognition and simple mapping by UAV/SfM for local resident in mountainous area in Vietnam—a case study in Po Xi Ngai community, Laocai province. In: Understanding and reducing landslide disaster risk, pp 103–109. https://doi.org/%2010.1007/978-3-030-60227-7_10
Nie Z, Zhang Z, Zheng H (2019) Slope stability analysis using convergent strength reduction method. Eng Anal Boundary Elem 108:402–410. https://doi.org/10.1016/j.enganabound.2019.09.003
Plaxis connect V20 (2020) Material models manual
Tien PV, Sassa K, Takara K, Tam DM, Quang LH, Khang DQ, Luong LH, Loi DH (2016) The influence of rainfalls on the potential of landslide occurrence on Hai Van Mountain in Vietnam. In: Proceeding of the final SATREPS workshop on landslides, 13 October, Hanoi, Vietnam, pp 112–121. ISBN: 978-4-9903382-3-7
Tschuchnigg F, Schweiger HF, Sloan SW, Lyamin AV, Raissakis I (2015) Comparison of finite-element limit analysis and strength reduction techniques. Geotechnique 65(4):249–257. https://doi.org/10.1680/geot.14.P.022
Author information
Authors and Affiliations
Corresponding author
Editor information
Editors and Affiliations
Rights and permissions
Open Access This chapter is licensed under the terms of the Creative Commons Attribution 4.0 International License (http://creativecommons.org/licenses/by/4.0/), which permits use, sharing, adaptation, distribution and reproduction in any medium or format, as long as you give appropriate credit to the original author(s) and the source, provide a link to the Creative Commons license and indicate if changes were made.
The images or other third party material in this chapter are included in the chapter's Creative Commons license, unless indicated otherwise in a credit line to the material. If material is not included in the chapter's Creative Commons license and your intended use is not permitted by statutory regulation or exceeds the permitted use, you will need to obtain permission directly from the copyright holder.
Copyright information
© 2023 The Author(s)
About this chapter
Cite this chapter
Nguyen, L.C., Do, TN., Nguyen, Q.D. (2023). Characteristics and Remedy Solutions for a New Mong Sen Deep-Seated Landslide, Sapa Town, Vietnam. In: Alcántara-Ayala, I., et al. Progress in Landslide Research and Technology, Volume 1 Issue 2, 2022. Progress in Landslide Research and Technology. Springer, Cham. https://doi.org/10.1007/978-3-031-18471-0_29
Download citation
DOI: https://doi.org/10.1007/978-3-031-18471-0_29
Published:
Publisher Name: Springer, Cham
Print ISBN: 978-3-031-18470-3
Online ISBN: 978-3-031-18471-0
eBook Packages: Earth and Environmental ScienceEarth and Environmental Science (R0)